Abstract
This review considers the role of α-cells in β-cell generation and regeneration. We describe recent evidence obtained from lineage-tracing studies showing that α-cells can serve as progenitors of β-cells and present a hypothetical model how injured β-cells might activate α-cells in adult islets to promote β-cell regeneration. β-cells appear to arise by way of their trans-differentiation from undifferentiated α progenitor cells, pro-α-cells, both during embryonic development of the islets and in the adult pancreas in response to β-cell injuries. Plasticity of α-cells is endowed by the expression of the gene encoding proglucagon, a prohormone that can give rise to glucagon and glucagon-like peptides (GLPs). The production of glucagon from proglucagon is characteristic of fully-differentiated α-cells whereas GLP-1 becomes a product of undifferentiated α-cells. GLP-1, a cell growth and survival factor, is proposed to promote the expansion of undifferentiated pro-α-cells during development. β-cells arise from pro-α-cells by a change in the relative amounts of the transcription factors Arx and Pax4, master regulators of the α- and β-cell lineages, respectively. A paracrine/autocrine model is proposed whereby injuries of β-cells in adult islets induce the production and release of factors, such as stromal cell-derived factor-1, that cause the de-differentiation of adjacent α-cells into pro-α-cells. Pro-α-cells produce GLP-1 and its receptor that renders them competent to trans-differentiate into β-cells. The trans-differentiation of pro-α-cells into β-cells provides a potentially exploitable mechanism for the regeneration of β-cells in individuals with type 1 diabetes.
Introduction
The functions of α-cells in the pancreatic islets have remained somewhat of an enigma.Citation1 They are known to produce the hormone glucagon in the post-absorptive state to maintain plasma glucose levels by stimulating hepatic glucose production. Based on recent studies, however, α-cells have been assigned a new role in the islets as direct progenitors of β-cells. In conditions of injury or depletion of β-cells, α-cells that lie adjacent to β-cells in the islets trans-differentiate into β-cells. This new role of α-cells to protect and to generate new β-cells might be their most important one.
Historically, the α-cells were discovered by virtue of the identification of a hyperglycemic factor in pancreas extracts whose actions appeared before the hypoglycemic actions of insulin.Citation2 This factor subsequently proved to consist of the hormone glucagon. The development of antisera to glucagon allowed for the immunocytochemical identification of α-cells as a subpopulation of endocrine cells in the islets distinct from the insulin-producing β-cells.Citation3 Seminal observations of the endocrine cells in early mouse pancreas development detected glucagon-positive cells as the earliest endocrine cells to appear at the onset of pancreas organogenesis in the rat.Citation4 These prescient findings suggested that the secreted products of these early glucagon-positive endocrine cells might have a function in early embryogenesis such as the regulation of growth and differentiation of embryonic endocrine cells.Citation4
The generally recognized function of glucagon is the stimulation of hepatic glucose production during periods of fasting to maintain plasma glucose levels in the post-absorptive state. The actions of glucagon are counter-regulatory to those of insulin, which are to promote glucose uptake and to lower plasma glucose levels. Glucagon, unlike insulin, however, is not essential for the general health of mice.Citation5 Loss of, or impaired, glucagon signaling in humans is not lethal.Citation6 In contrast to glucagon the near absence of insulin results in severe metabolic derangements resulting in lethality. Somewhat paradoxically, in the absence of glucagon signaling glucose homeostasis appears to be maintained in the absence of insulin. Mice lacking glucagon signaling, either by disruption of the glucagon receptorCitation7 or by lacking glucagon itself due to depletion of α-cells in islets by the disruption of Arx expression,Citation5 remain relatively healthy and do not develop diabetes in response to near complete ablation of β-cells by the administration of streptozotocin.Citation7 These observations of the benignity of the mouse phenotype in the absence of glucagon signaling suggests the existence of additional functions for α-cells, such as serving as guardians and progenitors for β-cell health, survival and regeneration.
In this review we discuss the evidence supporting the notion that α-cells are progenitors of β-cells, both in embryological development and in the regeneration of new β-cells in the adult pancreas. We present a hypothesis, the B > A > B hypothesis, supported by new experimental findings, that α-cells in the adult islets have a latent capacity to de-differentiate, expand, and switch-on the production of GLP-1, a known growth and survival hormone for β-cells. The de-differentiated pro-α-cells serve as progenitor cells for the formation of new β-cells. The production of GLP-1 by α-cells is initiated by factors secreted by β-cells in response to injuries. GLP-1 so produced and secreted feeds back in a local paracrine fashion on its constitutively expressed receptor in β-cells to promote their survival and regeneration. In conditions of severe β-cell injuries the de-differentiated pro-α-cells, resembling developmental endocrine progenitor cells, trans-differentiate into β-cells, recapitulating the ontologic development of the islet endocrine cells. Further evidence points to stromal cell-derived factor-1 (SDF-1) as one of the factors, produced in β-cells in response to their injury, that acts on adjacent α-cells to promote the production of GLP-1.
α-Cells Express the Proglucagon Gene (Gcg) Encoding a Prohormone Alternatively Cleaved to Glucagon and Glucagon-Like Peptides by the Actions of Prohomone Convertases
Since the discovery of α-cells as the source of glucagon it was found that glucagon production in α-cells occurs by way of selective proteolysis of a prohormone, proglucagon, encoded by a single gene (Gcg), that also contains other peptides such as glucagon-like peptides (GLPs) in addition to glucagon (). The regulation of the production of either glucagon or GLPs depends on the actions of the prohomone convertases PC2 or PC1/3, respectively. GLPs are growth factors and do not directly modulate glucose production per se. GLP-1 is a glucoincretin hormone with at least two distinct functions: the augmentation of glucose-dependent insulin secretion and the promotion of β-cell proliferation and survival.Citation8-Citation11 GLP-2 is a growth factor for intestinal epithelium.Citation12 It should be noted that paradoxically GLP-1 receptor agonists do not appear to stimulate the proliferation of human β-cellsCitation13 as they do so in the ratCitation14 and mouseCitation15,Citation16 β-cells.
Figure 1. Proglucagon expression in α cells. Alternative cleavages of proglucagon to glucagon and GLP-1. The relative levels of expression of the pro-hormone covertases PC2 and PC1/3 determine the production of glucagon or GLP-1, respectively. Glucagon is a metabolic (gluconeogenic) hormone produced in fully differentiated α-cells and GLP-1 is both an insulinotropic (insulin-releasing) hormone and a growth and survival peptide produced in undifferentiated pro-α-cells. GRPP, glucagon-related polypeptide. GLP-2, glucagon-like peptide-2 involved in intestinal growth.
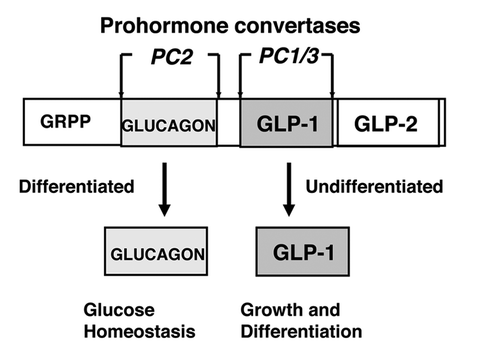
In fully differentiated α-cells of the adult pancreas GLP-1 is not normally produced in meaningful amounts. Glucagon instead is the hormone produced by the enzymatic cleavages of proglucagon. In contrast to mature α-cells, immature, undifferentiated α-cells, herein designated as pro-α-cells, produce GLP-1. Because proglucagon is constitutively expressed in α-cells, the potential exists for the production of GLP-1 as well as glucagon in the mature adult islet.Citation17,Citation18 Thus, α-cells appear to be endowed with an unusual plasticity in their ability to switch between the production of glucagon and GLP-1 depending on the relative levels of the prohormone convertases PC2 and PC1/3, respectively. The plasticity of α-cells resides with these two convertase switches in which fully-differentiated α-cells express only PC2 and produce glucagon, whereas undifferentiated pro-α-cells express PC1/3 as well as PC2 and produce GLP-1 in addition to glucagon.
α-Cells as Progenitors of β-Cells
Several lines of evidence indicate an important role for α-cells as direct progenitors of β-cells both in the embryonic development of the islets and in the regeneration of islets in the adult pancreasCitation19,Citation20 Here we review the evidence for α-cell progenitors in islet development (ontogeny), evidence derived from studies of islet tissues and embryonic stem cells in vitro, and in genetically altered mouse models using disruption and overexpression of key factors in α- and β-cell lineage commitment, lineage tracing approaches.
Ontogeny of islet cells
The pancreas arises during embryonic development from a nest of undifferentiated duct-like epithelial cells in the primitive foregut at mouse embryonic day e8.5 to e9.5. The endocrine cells destined to become islets differentiate from the undifferentiated epithelium at about e10-e11 during the primary transition involving lineage commitment from undifferentiated cells (reviewed in ref. Citation21). The endocrine lineage becomes distinct from that of the exocrine acinar tissue. The selective commitment of the undifferentiated epithelium into endocrine pancreas and the endocrine cells into the specific hormone-producing cells during development depends on the temporal and spatial expression of numerous transcription factors.Citation21 In brief, the undifferentiated duct-like epithelium expresses the transcription factor Pdx-1, a marker of cells committed to the pancreatic lineage (). The earliest endocrine cells that arise from the Pdx-1 positive duct-like epithelium express the endocrine specific transcription factor Ngn3Citation22 induces a population of cells that immunostain positive for glucagon (or proglucagon) and as such are designated to have an α-cell phenotype.Citation23 Some of these glucagon-positive cells co-express insulin but not Pdx-1, and are Ngn3 negative because the expression of Ngn3 in the mouse model used is under the control of the Pdx-1 promoter. Note that antisera to glucagon often cross react with the glucagon contained in the proglucagon precursor and are silent as to whether glucagon or GLP-1 peptides are the final products produced by cleavages of proglucagon in the cell. The co-expression of PC1/3 and proglucagon in cells would provide an indication that the cells are producing GLP-1.Citation24 The importance of Ngn3 for the programming of pancreas progenitor cells into a pro-endocrine lineage was demonstrated by the forced expression of Ngn3 in undifferentiated neonatal pig pancreas precursor cells resulting in their conversion into α-cells.Citation25 Additional support is provided by the findings that forced overexpression of Ngn3 in mice under the control of the Pdx-1 promoter drives early ectopic differentiation of an islet precursor population to a predominantly α-cell population.Citation26 These findings are consistent with a model in which pro-α-cells are the earliest islet endocrine phenotype to arise during development and are likely to be precursors of β-cells. During the late stages of the primary transition marking the beginning of the endocrine cell lineage (e8.5–e12.5) and into the early proliferation phase of the second transition (e11.5–14.5) the separate lineages of α, β, ™ and PP cells arise from Ngn3+ cells by selective lineage commitment.Citation23
Figure 2. Simplified model of the embryological development of islet endocrine cells (reviewed in ref. Citation21). Stem/progenitor (Stem/Prog) cells arise from the primitive undifferentiated epithelium of the gut tube and express the transcription factor Pdx-1, a master regulator of pancreas development. The endocrine lineage appears soon after and is characterized by the pro-endocrine transcription factor Ngn3. The earliest endocrine cells, pro-α-cells, identified in early development express the proglucagon gene and the prohormone convertase PC1/3 resulting in the production of GLP-1. The division of lineages into α- and β-cells is determined by the relative expression levels of the transcription factors Arx and Pax4. Mature α-cells express PC2 resulting in the production of glucagon. Fully-differentiated β-cells express the insulin gene and produce insulin. GLP-1 is proposed to be a growth factor important for the expansion of pro-α-cells Glucagon, produced late in α-cell development, is proposed to inhibit the growth of pro-α-cells.
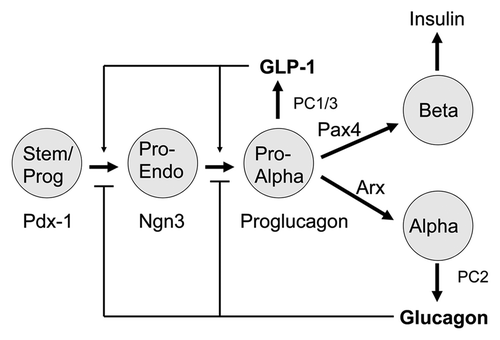
Numerous transcription factors are involved in the sequential derivation of the α- and β-cell lineages from Ngn3+ progenitors.Citation21 Certain of the transcription factors stand out as master switches of cell phenotype. Master switch factors are defined as those whose removal results in a cessation of further lineage development. As indicated above, Pdx-1 marks the early pancreatic lineage in the undifferentiated duct-like epithelium. Ngn3 marks the early pro-endocrine lineage as does the expression of proglucagon, PC1/3, and the GLP-1 receptor. The key transcription factors that appear to define the fully differentiated β-cell phenotype are Pdx-1, Pax4, Nkx 2.2, Nkx 6.1 and MafA. The differentiated α-cell phenotype is determined by the expression of Arx, Pax6, Brn4, Irx2, and the absence of Pdx-1, Pax4, PC1/3 and the GLP-1 receptor. The relative ratios of the factors Arx and Pax4 in endocrine progenitor cells appears to be critical for their commitment to an α- or β-cell fate, respectivelyCitation20,Citation21 (see below).
Whether or not β-cells are direct descendents of α-cells or arise independently from undifferentiated epithelial progenitor cells as distinct cell lineages remains controversial. One school of thought is based on lineage tracing experiments in mice showing that α- and β-cells arise independently as distinct lineages.Citation27 The other school of thought, based on sequential immunostaining of endocrine cells during embryonic development, showing co-expression of glucagon and insulin, and glucagon and somatostatin, suggests that α-cells differentiate into β-and ™-cells.Citation1,Citation28-Citation31 Cells expressing glucagon (proglucagon) appear during the initial formation of the pancreatic diverticulum in the rat embryo at the 20–25 somite stage.Citation4 At this early time of development the levels of glucagon in the diverticulum are 100–1,000 times higher than that of insulin suggesting that the glucagon-expressing cells might have a role in the growth and differentiation of endocrine cells.Citation4 The two schools of thought are not necessarily mutually exclusive. As discussed below, the recent evidence obtained from genetic manipulations and lineage tracing approaches in mice demonstrates a proof-of-concept that α-cells can give rise to β-cells in the adult pancreas. These findings are consistent with a developmental model in which α-cells might in some cell developmental lineages serve as obligate progenitors of β-cells.
Embryonic stem cells (ESCs)
Remarkably, GLP-1 and its receptor (GLP-1R) are expressed in embryonic stem cells (ESCS) Citation32,Citation33 raising the possibility that cell to cell signaling might occur via the GLP-1/GLP-1R axis and be involved not only in the expansion and differentiation of islet endocrine cells during development but also might have a role in the modulation of embryonic stem cell expansion and differentiation. The derivation of what appear to be fully-differentiated functional glucagon-producing α-cells from human ESCs in vitro has been accomplished providing a clear demonstration of a transition from stem cells to α-cells.Citation33,Citation34 Moreover, the cells derived from the ESCs expressed high levels of the Arx transcription factor and also produced GLP-1 suggesting that they are undifferentiated pro-α-cells in transition to fully-differentiated α-cells. These cells transiently co-expressed insulin along with proglucagon further supporting the remarkable plasticity of α-cells and their possible latent potential to be progenitors for β-cells as well as α-cells.Citation34
The finding of PC1/3 expression in developing endocrine cells suggests that the GLPs might be the major peptides expressed from the cleavage of proglucagon in the α-cell phenotype during embryonic development, and as such, these cells represent pro-α-cells.Citation24 Since GLP-1/GLP-1R signaling in islets cells has been shown to involve the Wnt signaling pathway consisting of β-catenin and Tcf7L2,Citation35-Citation37 it is tempting to speculate that Wnt signaling, driven in part by GLP-1 receptor activation in embryonic stem cells and in proglucagon-expressing endocrine progenitor cells, might be involved in the modulation of the development of endocrine progenitor cells.
Genetically altered mouse models
Studies accomplished in mice programmed with cell lineage tracing markers in vivo have revealed a remarkable plasticity of islet endocrine cells. α-cells trans-differentiate into β-cells and vice versa. Following severe injuries of β-cells in transgenic mice by diphtheria toxinCitation38 in mice with disruption of the multiple endocrine neoplasia-1 (Men 1) gene,Citation40 and overexpression of Pax4 in the pancreas lineage,Citation41 newly regenerated β-cells contain a lineage tracing marker of α-cells. The appearance of the α-cell-specific lineage marker in β-cells of these mouse models of β-cell generation and regeneration provides convincing evidence that the β-cells arose from progenitors of the α-cell phenotype. In addition to the lineage tracing studies, a mouse model in which neogenesis is stimulated by pancreatic duct ligation and β-cells are severely ablated by alloxan, shows a stepwise transition of α-cells to bihormonal cells (cells co-expressing glucagon and insulin) to β-cells.Citation39 In this study β-cells formed from α-cells by direct conversion with and without intervening cell division, suggesting that the asymmetric cell division is not required for α- to β-cell conversion, although some α-cells division appears necessary to maintain the pool of α-cells.Citation39 Remarkably, lineage tracing studies using mice with a marked β-cell lineage, instead of a marked α-cell lineage, show trans-differentiation of β into α-cells in response to the forced expression of Arx,Citation42 or the de-repression (activation) of Arx expression in β-cells.Citation43 Collectively, the lineage tracing and the pancreatic duct ligation/alloxan studies in mice, combined with the studies demonstrating the transient co-expression of proglucagon and insulin genes in early endocrine progenitor cells during islet development, provide proof-of-principle evidence that α-cells beget β-cells.Citation44
Arx and Pax4, master determinants of the α and β phenotypes
Of particular relevance to understanding the mechanisms responsible for the transition of α to β-cells, and vice versa, are the two transcription factors Arx and Pax4.Citation20 These factors appear to act in a reciprocal fashion in the early Ngn3 positive endocrine progenitor cells.Citation20 High expression of Arx relative to Pax4 promotes the α-cell phenotype and low expression of Arx relative to Pax4 drives differentiation to the β-cell phenotype. In genetically altered mouse models with either overexpression of ArxCitation42 or disruption of expression of Pax4 Citation45 result in excessive numbers of α-cells and a deficiency of β-cells. Likewise, the overexpression of Pax4 Citation41 or the disruption of the expression of ArxCitation46 leads to a deficiency of α-cells and an increase in β-cells. The expression of Pax4 is low in fully mature adult β-cells suggesting that the role of this transcription factor is to program Ngn3+ progenitor cells into the β-cell lineage pathway.Citation47 The lineage model emerging from current investigations is one in which Ngn3 is a key regulator of the undifferentiated endocrine progenitor cell. Pax4 is a key factor in the commitment of progenitor cells to a β-cell phenotype. Once committed to the β-cell phenotype expression of Pax4 defervesces and other transcription factors such as PDX-1 and MafA assume control of the adult mature β-cell.
Severe depletion of β-cells results in the trans-differentiation of α- to β-cells without proliferation
It is important to appreciate that in the studies of β-cell ablation by diphtheria toxin,Citation38 or alloxan in combination with partial pancreatic duct ligation to stimulate neogenesis,Citation39 the regeneration of new β-cells, although only ~20% efficient, occurred by their direct trans-differentiation from preexisting α-cells without evidence of replication of β-cells.Citation38,Citation39 In both studies the ablation of β-cells was extreme; > 99%. These mouse models of extreme injury of β-cells appears to differ from other models of less severe β-cell injuries in which α-cell hyperplasia is a hallmark and β-cell replication is involved in regeneration.Citation46,Citation48-Citation52
Intriguing new evidence, however, obtained from lineage tracing studies in mice support the existence of stem/progenitors in islets as an alternative mechanism for β-cell renewal in addition to replication.Citation48 Tamoxifen pulse-labeling of mice expressing the insulin gene reported by a tamoxifen-inducible lineage tracing marker (alkaline phosphatase) revealed that by 12 mo of age most new β-cells replacing senescent β-cells lost by aging during these 12 mo contained the alkaline phosphatase lineage marker. These cells had initiated the production of insulin during aging. In addition, the majority of regenerated β-cells 2 weeks after treatment of the mice with the β-cell toxin streptozotocin, contained the lineage tracing marker indicating the regeneration of new β-cells occurred by their differentiation from progenitor cells as well as by proliferation of existing β-cells.Citation53 An interesting aspect of these studies is that the linage-tracing marker was programmed by the insulin promoter, indicating that the lineage-marked cells at one time expressed insulin. These findings are consistent with similar lineage-tracing studies showing that adult mouse and human islets contain multipotent-progenitor cells marked by their expression of the insulin gene.Citation54
In studies of the neogenic response to alloxan with pancreatic duct ligation a prompt expansion of α-cells occurred followed by their efficient conversion to β-cells.Citation39 Although lineage tracing was not used in these experiments, the time-course of the appearances of bihormonal and β-cells supports a trans-differentiation model.Citation39 Notably, pancreatic duct ligation alone without ablation of β-cells by alloxan, produced the previously demonstrated ductal neogenesis involving the formation of glucagon-positive α-like progenitor cells from undifferentiated ductal epitheliumCitation55,Citation56 but no transformation of α- to β-cells was detected.Citation39 These findings raise the possibility that the severe injury of β-cells (streptozotocin or alloxan) and resulting very severe loss of β-cells might be in some way responsible for the absence of proliferation of the endocrine cells. Perhaps the loss of a growth factor produced by mature β-cells and not by the new regenerated β-cells derived by the trans-differentiation of α-cells, is required for proliferation. The loss of insulin in response to extreme injury of β-cells might be considered to be a factor in stimulating the proliferation of α-cells because of the known paracrine-mediated suppressive actions of insulin on α-cells.Citation57-Citation59 However, this scenario seems unlikely because α-cell-specific disruption of insulin signaling in mice does not lead to α-cell hyperplasia.Citation60,Citation61 The loss of insulin signaling on α-cells does result in an age-dependent trans-differentiation of a small number of α- into β-cells, suggesting that the release of insulin inhibitory influences on α-cells might in some circumstances render them competent to become β-cells.Citation61
Glucagon inhibits the expansion of Ngn3+ α-like endocrine progenitor cells
Either ectopic overexpression of Pax4 Citation41 or disruption of Arx expression Citation5 in the pancreas lineages of mice results in a severe depletion of α-cells and a corresponding decrease in the production of glucagon. In both of these circumstances of α-cell depletion, β-cell mass is greatly increased. Pax4 overexpression rapidly converted α-like progenitors into β-cells and thereby increased β-cell mass by 8-fold and drastically depleted the pool of α-cells resulting in a deficiency of glucagon.Citation41 The systemic administration of glucagon to these mice reduced the production and expansion of progenitor cells and correspondingly reduced the enlargement of β-cell mass. These observations raise the possibility that glucagon, the product of mature, fully-differentiated α-cells, exerts negative feed-back inhibition on the formation of the α-like progenitor cells and their subsequent differentiation into β-cells (). This feed-back mechanism might then modulate the mass of β-cells and prevent the over-production of β-cells (β-cell hyperplasia), in circumstances in which the α- and β-cell compartments of the islets are fully-formed and are balanced to meet physiological demands required to maintain nutrient homeostasis.
Role of α-Cells in the Regeneration of β-Cells in the Adult Pancreas
α-cell hyperplasia
An understanding of the mechanisms leading to the growth of α-cells, and consequent development of α-cell hyperplasia, in adult islets might provide insight into approaches to increase the progenitor cell pool for the formation on new β-cells. Adaptive α-cell hyperplasia occurs in circumstances of deficient glucagon signaling resulting in a loss of glucagon function on hepatic gluconeogenesis resulting in persistent hypoglycemia. Paradoxical α-cell hyperplasia occurs in response to injury of β-cells and a corresponding reduction in insulin production resulting in hyperglycemia.
Adaptive hyperplasia due to defective glucagon signaling
Alpha-cell hyperplasia develops in response to states of defective glucagon signaling. These states include glucagon deficiency due to the absence of the prohormone convertase, PC2, required for the cleavage of proglucagon into glucagon,Citation62-Citation64 disruption of the expression of the proglucagon gene,Citation65 or glucagon resistance induced in mice by transientCitation66-Citation68 or complete,Citation7,Citation69-Citation72 disruption of the glucagon receptor, and impairment of hepatic glucose production by liver-specific disruption of the expression of Gs α required for glucagon receptor signaling in the liver.Citation73 An intriguing aspect of these studies in mice with impaired glucagon signaling is that α-cell hyperplasia is invariably accompanied by increased plasma levels of GLP-1 (and glucagon) and an increased production of GLP-1 in the islets.Citation66,Citation69,Citation73-Citation77
Adaptive α-cell hyperplasia might be viewed of as a response of the islets to produce more glucagon to counteract glucagon resistance in order to increase hepatic glucose production to correct the chronic hypoglycemia, much as β-cell hyperplasia develops in response to insulin resistance in order to produce more insulin to correct the hyperglycemia. Notably, the administration of glucagon to PC2 knockout mice restores euglycemia and ameliorates α-cell hyperplasia.Citation63
The mechanism(s) responsible for α-cell hyperplasia in conditions of impaired glucagon signaling are unknown. A speculative possible mechanism is a loss of inhibition of α-cells by insulin.Citation57-Citation59,Citation61 Mice with impaired glucagon signaling have modestly decreased β-cell mass and/or pancreas insulin contentCitation7,Citation62,Citation70 and plasma insulin levels tend to be lower compared with wild-type mice.Citation67,Citation69,Citation72,Citation73,Citation78 These mutant mice manifest increased whole-body insulin sensitivity,Citation73,Citation79 decreased β-cell mass, and impaired glucose-stimulated insulin secretion.Citation79 These findings are consistent with a lesser requirement for insulin in the absence of the insulin-counter-regulatory actions of glucagon. In support of this model of compensatory adaptation of insulin demands is the report that mice lacking the glucagon receptor remain euglycemic and insulin sensitive in conditions of near complete absence of insulin.Citation7 A lower requirement for insulin might result in a decreased intra-islet paracrine inhibitory signaling to α-cells. An argument against a lack of suppressive insulin signaling on α-cell growth, per se, is the finding that targeted ablation of the insulin receptor on α-cells did not result in a phenotype of α-cell hyperplasia.Citation61
Another possible mechanism for the development of α-cell hyperplasia in the absence of effective glucagon signaling is the loss of autocrine negative feedback inhibition of α-cells. Glucagon is predicted to inhibit glucagon secretion by α-cells.Citation80 Further, as mentioned earlier, glucagon inhibits the expansion of Ngn3-expressing progenitors in the pancreas of mice overexpressing the transcription factor Pax4.Citation41 Since a small subpopulation (9%) of adult α-cells express the glucagon receptor,Citation81 and an additional small population (3%) express the endocrine stem cell transcription factor Pdx-1,Citation82 it is tempting to speculate on the possibility that a subpopulation of α-cells in adult islets are undifferentiated pro-α-cells and they are the cells that expand in mice rendered defective in glucagon signaling.
Paradoxical α-cell hyperplasia, a hallmark of β-cell injury
Paradoxically, α-cell hyperplasia also occurs in conditions of insulin deficiency and hyperglycemia resulting from injury of β-cells. α-cell hyperplasia is a hallmark manifestation in mice given the β-cell toxins streptozotocinCitation7,Citation41,Citation83-Citation86 or alloxan following pancreatic duct ligation,Citation39 in baboons with inborn islet amyloidosis,Citation87 diet-induced obese mice with insulin resistance and impaired β-cell functionCitation88 or autoimmune destruction of β-cells in NOD mice.Citation89 A study of a type 2 diabetes model in mice using low dosage streptozotocin found a maintenance of α-cell mass compared with a 75% loss of β-cell mass.Citation90
Pancreases obtained from humans with autoimmunity type 1 or type 2 diabetes show an overall maintenance of α-cell mass displaying a large extent of heterogeneity among donors. An extensive study of pancreases from 47 donors with type 1 diabetes showed over all a slight reduction in total α-cell mass per pancreas with some pancreases showing either no change or a marked increase in α-cell mass compared with non-diabetic, age-matched controls.Citation91 In pancreases from type 2 diabetes donors α-cell mass appears to be maintained on average.Citation92 In another study islets composed nearly entirely of α-cells are described.Citation93 Studies of islet cell replication in pancreases from donors with recent-onset type 1 diabetes reveal rates of replication of both α- and β-cells that are 10-fold greater than control islets from non-diabetic donors.Citation94 These studies suggest that in diverse conditions of β-cell injuries in animals and humans α-cell mass is either preserved or increased in the face of severe ongoing destruction of β-cells. If, as discussed in this review, α-cells can trans-differentiate into β-cells in these conditions of β-cell injuries, it seems that in both type 1 and type 2 diabetes the mass of α-cells retained is sufficient to provide a precursor pool for the formation of new β-cells. These observations or increased proliferation of both α- and β-cells in type 1 diabetes also suggest that α-cells must be actively expanding and transforming into new β-cells.Citation94
Hyperplastic α-cells as undifferentiated pro-α-cells
The paradoxical growth of α-cells in conditions of β-cell injuries and hyperglycemia might be explained by the induction of a change in the phenotype of the α-cells associated with injuries of β-cells. Injured β-cells might produce factors, such as cytokines and chemokines that act on adjacent α-cells resulting in the change in their phenotype. Such a change in cellular phenotype might involve a de-differentiation of mature α-cells to undifferentiated pro-α-cells. Thereby the biologic functions of the α-cells switches from a mature α-cell that produces glucagon involved in glucose metabolism to an undifferentiated pro-α-cell providing local growth factors, such as GLP-1, that are involved in the regeneration of the injured β-cells.
In this context we propose that pro-α-cells represent a population of undifferentiated (immature) islet endocrine cells that express PC1/3 and the GLP-1 receptor because these properties distinguish immature α-cells during embryonic development from those of mature adult α-cells.Citation24,Citation95,Citation96 We also propose that fully mature α-cells in the adult pancreas can be transformed by de-differentiation into immature pro-α-cells in response to injuries of β-cells. During development pro-α-cells can be precursors of either the α-cell lineage or the β-cell lineage. Furthermore, pro-α-cells might transiently differentiate into β-cells, express the insulin gene, either with or without co-expression of the proglucagon gene (bi-hormonal cells), and then differentiate into β or α-cells.Citation34,Citation97 The lineage commitments of endocrine progenitor cells might involve a series of progressive steps of differentiation, de-differentiation and re-differentiation of stem/progenitor cells until a successful functional lineage is achieved.
Several lines of evidence support the notion that hyperplastic α-cells are undifferentiated pro-α-cells that express PC1/3, the GLP-1 receptor, and produce GLP-1. Mice and rats with α-cell hyperplasia arising from defective glucagon signalingCitation69,Citation72,Citation73 or β-cell injury by streptozotocinCitation84,Citation98 have markedly elevated plasma GLP-1 levels and high levels of intra-islet GLP-1.Citation66,Citation69,Citation73,Citation74,Citation84 The increased production of GLP-1 by hyperplastic α-cells requires their expression of the prohormone convertase PC1/3. Normal adult α-cells in islets express PC2 required for the production of glucagon and PC1/3 is undetectable.Citation74,Citation96 Accordingly, hyperplastic α-cells in mice with defective glucagon signaling,Citation69 mice with β-cell stress or injuries,Citation74,Citation84 and obese, diabetic gerbilsCitation99 express PC1/3. Injuries of isolated islets ex vivo induce the expression of PC1/3 and the production of GLP-1 in α-cells.Citation76,Citation77 Curiously, islets isolated from PC2 null mice with α-cell hyperplasia appear to display a defect in the processing of proglucagon to both glucagon and to GLP-1.Citation100 Levels of PC1/3 in the islets of these mice are not increased.Citation100 In contrast to isolated islets, a cell line, α TC1deltaPC2, derived from the α-cells of PC2 null mice has elevated levels of PC1/3 and produces GLP-1 from proglucagon.Citation101
The selective ectopic expression of PC1/3 in place of PC2 in α-cells of mouse islets enhances the survival of β-cells in the islets when transplanted into diabetic mouse recipients.Citation96,Citation102 Moreover, the hyperplastic α-cells that arise in the mouse with defective glucagon signaling due to disruption of the expression of the glucagon receptor express the GLP-1 receptor throughout development and in the adult islets.Citation95 Whether or not the GLP-1 receptor is expressed in α-cells of the adult islet is unclear. In one study the GLP-1 receptor is expressed in a small (20%) population of adult α-cells of normal ratsCitation103 and in other studies it was not detectable on α-cells from mouse,Citation95 human or rats.Citation104,Citation105 These findings, although disparate, suggest that diverse injuries of β-cells, or defective glucagon signaling, resulting in the development of α-cell hyperplasia are required to induce the expression of the GLP-1 receptor, PC1/3, and the processing of proglucagon to GLP-1 in α-cells. This change in phenotype of the α-cells recapitulates the phenotype of pro-α progenitor cells seen in the embryonic development of the endocrine cells.Citation31,Citation90 GLP-1/GLP-1R axis signaling might be one mechanism involved in the proliferation of α-cells in the absence of effective glucagon signaling.
The B > A > B Hypothesis: A Mechanism for How Injured β-cells might Signal to α-cells and Regenerate β-cells
Based on current evidence α-cells appear to be critically involved in both the generation of β-cells during development and in the regeneration of β-cells in the adult pancreas in response to injuries invoked by means such as autoimmunity, gluco-lipotoxicity and streptozotocin. The observations of α-cell hyperplasia in response to β-cell injuries, the production of GLP-1 by hyperplastic α-cells, and the capacity for α-cells to trans-differentiate into β-cells, led to the conception of a possible cellular mechanism that might be involved in the regeneration of β-cells. This mechanism involves paracrine and autocrine signaling among β- and α-cells and is referred to the B > A > B hypothesis: injured β-cells signal to α-cells, which in turn signal to β-cells to stimulate their regenerationCitation77 or trans-differentiate into β-cells depending on the extent of β-cell injury ().
Figure 3. Model illustrating the B > A > B hypothesis of the autocrine/paracrine actions of SDF-1 and GLP-1 in α-cell-mediated regeneration of β-cells. Stromal cell-derived factor-1 (SDF-1) produced by injured β-cells acts on adjacent α-cells stimulating their de-differentiation to pro-α-cells. SDF-1 activates Akt and Jak/STAT signaling pathways in mature, fully-differentiated α-cells resulting in the expression of PC1/3 and the production of GLP-1. De-differentiated pro-α-cells express Ngn3, a hallmark of endocrine progenitor cells and Pax4, a master determinant of the differentiation of progenitor cells to β-cells. GLP-1 produced by pro-α-cells and SDF-1 signaling promote the trans-differentiation of pro-α into β-cells. GLP-1 and SDF-1 participate in the proliferation and the survival of both pro-α-cells and newly regenerated β-cells. A deficiency of glucagon and insulin, the products of fully-differentiated α- and β-cells, respectively, facilitates the transition of mature α to pro-α to β-cells, i.e., glucagon and insulin are inhibitory to the progression of de-differentiation and trans-differentiation. Wnt signaling might be involved in the signaling mediated by both SDF-1 and GLP-1 as both hormones activate β-catenin/Tcf7l2-mediated downstream Wnt signaling.Citation35,Citation36,Citation106 The transcription factors Arx and Irx2 and Pdx1, Pax6 and MafA define the phenotypes or mature α- and β-cells, respectively.Citation107,Citation108
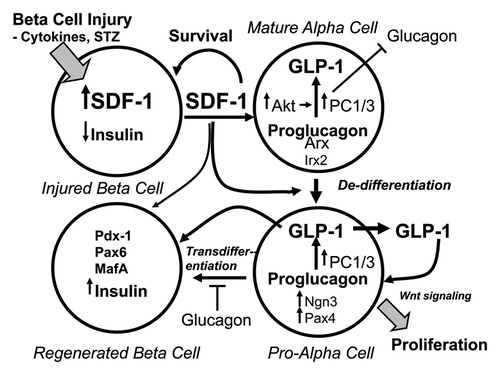
Paracrine inter-cellular communications between β- and α-cells in the islets is well recognized.Citation57,Citation109-Citation111 This cyto-architecture consisting of the juxta-positioning of β-cells next to non-β-cell endocrine cells such as α-cells is conducive to cell-cell paracrine signaling mechanisms. In particular in human islets the α-cells are admixed with the β-cells so that > 70% of β-cells are in heterotypic contact with non-β endocrine cells,Citation112,Citation113 the majority of which are α-cells.Citation57,Citation109,Citation110 Although the observations that injuries of β-cells induce the expression of PC1/3 and the production of GLP-1 cells in α-cells is established, the question remains how do injured β-cells activate α-cells?
An initial observation of cross-talk between injured β-cells and the activation of α-cells suggested the possibility that factors(s), such as stromal cell factor-1 (SDF-1), released from injured β-cells directly activate α-cells.Citation83 SDF-1 is a chemokine produced in multiple organs in response to injuries and is involved in the regeneration and renewal of damaged tissues.Citation114,Citation115 SDF-1 exerts cytoprotective actions on β-cellsCitation33,Citation71,Citation77,Citation106,Citation116 and is expressed in β-cells during development and re-expressed in adult β-cells in response to β-cell injuries.Citation77 SDF-1 receptors, known as CXCR4, are expressed at high levels on both α- and β-cells.Citation77,Citation83
The treatment of mice with streptozotocin, a selective toxin for β-cells that does not affect α-cells, resulted in the activation of the pro-survival kinase Akt in α-cells in the mouse islets 6 h after the administration of streptozotocin.Citation83 Further, in these same studies a similar dose of streptozotocin given to transgenic mice expressing the SDF-1 in β-cells, in contrast to wild-type mice, resulted in a regeneration of 50% of the β-cells after 2 weeks.Citation83 The islets of the wild-type mice two weeks after the administration of streptozotocin consisted almost entirely of α-cells.Citation83 This observation led to the conception of the B > A > B hypothesis of paracrine and autocrine cross-talk between β- and α-cells and α- and β-cells in the islets and that SDF-1 might be important in the regeneration of injured β-cellsCitation77 (). In addition to SDF-1, the cytokine Interleukin-6 (IL-6) is a potential candidate factor emanating from injured β-cells that might be involved in α-cell-mediated β-cell regeneration. IL-6 also promotes α-cell hyperplasiaCitation88 and the production of GLP-1 by α-cells.Citation117 The neutralization of IL-6 actions prevents the α-cell hyperplasia and corresponding increase in GLP-1 production that occurs in diet-induced obese mice.
Support for the B > A > B model is provided by studies demonstrating the de-differentiation of α-cells and their re-differentiation into β-cells in response to injury of β-cells by the administration of stretozotocin. The administration of streptozotocin to 4 d-old rat pups induced the expression of Ngn3 and Pax 4 in α-cells, a maintenance of α-cells, and a 60% regeneration of β-cell mass by 20 d.Citation118 Remarkably, a recent study found a complete regeneration of islets and the amelioration of diabetes in rats by four weeks following streptozotocin-induced ablation of β-cells and the transient administration to the pancreas of expression plasmids encoding GLP-1, cyclin D1 and cyclin-dependent kinase 4.Citation119 GLP-1 expression alone resulted in about a 50% improvement in glycemic control and a partial regeneration of β-cells. Most notably, the precursor pool appears to be predominantly de-differentiated α-cells, pro-α-cells, as by 1 week after the administration of streptozotocin and the expression plasmids, the rapidly proliferating cells were located on the mantle (periphery) of the islets and co-expressed glucagon, insulin, Ngn3, Sox9, Pdx-1, Beta2/NeuroD, sonic hedgehog and amylase. This gene expression profile of the precursor cells arising after severe injury of the β-cells by streptozocin mimics key features of undifferentiated islet endocrine precursors seen during embryonic development. These observations provide compelling evidence that latent β-cell precursors exist within the islets of adult rats.
The B > A > B model proposes the de-differentiation of mature α-cells to undifferentiated pro-α-cells by SDF-1-mediated signaling emanating from the injured β-cells. The de-differentiation of the α-cells by the actions of SDF-1 allows them to expand, perhaps by activation of the Wnt signaling pathway,Citation120 and renders them competent to trans-differentiate into β-cells in the presence of GLP-1 signaling and the absence of insulin and glucagon, which are proposed to suppress pro-α-cell growth and differentiation. Mature α-cells, characterized by the expression of PC2 and their production of glucagon, and the transcription factors Arx,Citation20 Pax6,Citation121 and MafB,Citation122 are induced to de-differentiate into pro-α-cells. The pro-endocrine transcription factor Ngn3 is re-induced as a consequence of the de-differentiation of mature α-cells to pro-α-cells. The pro-α-cells express PC1/3 resulting in the production of GLP-1, and the GLP-1 receptor is induced. Notably, as described earlier, pro-α-cells express the GLP-1 receptor, which is not expressed on fully differentiated α-cells in adult islets.Citation95 The undifferentiated pro-α-cells are then driven to trans-differentiate into β-cells by the activation of an autocrine GLP-1/GLP-1R axis that induces the expression of the β-cell factor Pax4.Citation13 It is proposed that in pro-α-cells Ngn3 is an activator of the expression of Pax4.Citation123 The expression of Pax4 promoted by GLP-1 signaling transforms the pro-α-cells into newly-regenerated β-cells that express the β-cell transcription factors Pdx1, MafA, Pax4, Nkx2.2 and the insulin gene.
Additional support for the notion that islet α-cells might have the capacity do de-differentiate under conditions of β-cell injuries is provided by several studies of islet-derived endocrine cells cultured in vitro showing that they de-differentiate, expand, and can be re-differentiated into insulin-producing β-like cells.Citation124-Citation126 The sequence of de-diffentiation, expansion and re-differentiation is similar to the epithelial to mesencymal transitions (EMT) and mesenchymal to epithelial transitions (MET) that occur during gastrulation and embryo development.Citation127,Citation128 These studies in rats in vivo and in islet cells in vitro lend support to the B > A > B hypothesis in which both paracrine and autocrine signaling via the SDF-1/CXCR4 and the GLP-1/GLP-1R axes alter the phenotypes of α- and β-cells in the promotion of their proliferation and survival.
Summary and Future Directions
The results of recent studies indicate that α-cells might have a new and unexpected function in the islets and that function is to serve as guardians for the repair and regeneration of injured β-cells. The production of glucagon by fully differentiated α-cells in the adult islets is an important, but not essential, function to maintain plasma glucose levels during the post-absorptive state as mice lacking glucagon signaling remain relatively healthy. This circumstance suggests that α-cells might have important functions other than glucose production such as the regeneration of β-cells. The α-cells situated adjacent to β-cells in the islets are positioned to rescue injured β-cells via paracrine mechanisms. Alpha-cells sense and respond to signals emanating from injured β-cells, such as SDF-1, by initiating the local production of GLP-1, a growth and survival factor for β-cells. In response to extreme injury of β-cells, α-cells de-differentiate to pro-α-cells, similar to embryonic endocrine progenitors and trans-differentiation into new β-cells.
Much evidence has established that α-cells increase in numbers in response to β-cell stress and injuries of various origins, as well as to the absence of glucagon actions. Additional evidence indicates that α-cells are progenitors of β-cells and under certain circumstances can trans-differentiate into β-cells. Although α-cells are plentiful in the islets of diabetic individuals and mice and rats, β-cells are reduced in numbers and secretion of insulin in response to nutrient signals is impaired.Citation90-Citation94 Given these circumstances the question arises as to why β-cell mass and function is impaired in diabetes if α-cells are progenitors of β-cells? It seems that hyperplastic α-cells are poised to become β-cells but the environmental cues required to make this happen are missing. One important avenue for future investigations is to identify the factor(s) required to covert the abundance of α-cells into functional β-cells that will make and secrete insulin in meaningful amounts and in a regulated fashion.
The α-cells remaining after the depletion of β-cells are proposed to be de-differentiated pro-α-cells that have been rendered competent to commit to β-cells. However, under normal circumstances they do not commit to β-cells. The weight of the evidence points to the transcription factors Arx and Pax4 as the master determinants of the α- and β-cell types. A loss of Arx and/or a gain of Pax4 function favor commitment of progenitor cells to a β-cell phenotype. Approaches that reduce Arx activity might be counterproductive. Loss of Arx functions decreases the formation of α-cells, and if α-cells are obligate progenitors of β-cells, the loss of Arx might reduce the pool of progenitors for β-cells. On the other hand, inhibition of Arx expression might shunt pro-a progenitors into the formation of b-cells. Increasing Pax4 function in pro-α-cells seems to be a more promising approach. The overexpression of Pax4, in the presence of other factors, such as Nkx2.2 and a phosphatidylinositol-3-kinase inhibitor, converts embryonic stem cells to insulin-producing β-like cells in vitroCitation129-Citation132 and forced expression of Pax4 in the pancreatic lineage in mice dramatically converts α- to β-cells.Citation41 The next question is how to induce the expression of Pax4 in the pro-α-cells of β-cell-depleted islets in diabetic individuals?
The promoter of Pax4 is under complex control by multiple signal transduction pathways including PI3 kinase, mitogen-activated kinases and cAMP-activated kinase, and Smad signaling by activin.Citation13 Downstream signaling activates nuclear transcription factors and epigenetic mechanisms involving the actions of hepatic nuclear factors 1α and 4α, Pdx1, Ngn3 and Beta2/NeuroD that regulate Pax4 expression.Citation123,Citation133 Therefore, based on the evidence that SDF-1/CXCR4 and GLP-1/GLP-1R axes signaling is involved in the paracrine cross-talk between α- and β-cells, it is tempting to speculate that a combination of SDF-1 and GLP-1 receptor agonism might coax the trans-differentiation of α to β-cells. The SDF-1 receptor, CXCR4, is coupled to G-protein Gi α and the GLP-1 receptor is coupled to G-protein Gs α subunit. The combined signaling of SDF-1 and GLP-1 might be sufficient to activate the expression of Pax4 in α-cells resulting in their trans-differentiation into β-cells.
Abbreviations: | ||
GLP-1 | = | glucagon-like peptide-1 |
SDF-1 | = | stromal cell-derived factor-1 |
PC | = | prohormone convertase |
Acknowledgments
We thank Vishal Saxena for careful reading and suggestions for the manuscript. The concepts and studies described were supported in part by grants from the Juvenile Diabetes Research Foundation, the American Diabetes Association and Novo Nordisk.
References
- Gromada J, Franklin I, Wollheim CB. Alpha-cells of the endocrine pancreas: 35 years of research but the enigma remains. Endocr Rev 2007; 28:84 - 116; http://dx.doi.org/10.1210/er.2006-0007; PMID: 17261637
- Lefèbvre PJ. Early milestones in glucagon research. Diabetes Obes Metab 2011; 13:Suppl 1 1 - 4; http://dx.doi.org/10.1111/j.1463-1326.2011.01437.x; PMID: 21824250
- Orci L. Macro- and micro-domains in the endocrine pancreas. Diabetes 1982; 31:538 - 65; PMID: 6759269
- Rall LB, Pictet RL, Williams RH, Rutter WJ. Early differentiation of glucagon-producing cells in embryonic pancreas: a possible developmental role for glucagon. Proc Natl Acad Sci U S A 1973; 70:3478 - 82; http://dx.doi.org/10.1073/pnas.70.12.3478; PMID: 4519640
- Hancock AS, Du A, Liu J, Miller M, May CL. Glucagon deficiency reduces hepatic glucose production and improves glucose tolerance in adult mice. Mol Endocrinol 2010; 24:1605 - 14; http://dx.doi.org/10.1210/me.2010-0120; PMID: 20592160
- Abs R, Verbist L, Moeremans M, Blockx P, De Leeuw I, Bekaert J. Hypoglycemia owing to inappropriate glucagon secretion treated with a continuous subcutaneous glucagon infusion system. Acta Endocrinol (Copenh) 1990; 122:319 - 22; PMID: 2183535
- Lee Y, Wang MY, Du XQ, Charron MJ, Unger RH. Glucagon receptor knockout prevents insulin-deficient type 1 diabetes in mice. Diabetes 2011; 60:391 - 7; http://dx.doi.org/10.2337/db10-0426; PMID: 21270251
- Kieffer TJ, Habener JF. The glucagon-like peptides. Endocr Rev 1999; 20:876 - 913; http://dx.doi.org/10.1210/er.20.6.876; PMID: 10605628
- Drucker DJ. The biology of incretin hormones. Cell Metab 2006; 3:153 - 65; http://dx.doi.org/10.1016/j.cmet.2006.01.004; PMID: 16517403
- Drucker DJ. Glucagon-like peptides: regulators of cell proliferation, differentiation, and apoptosis. Mol Endocrinol 2003; 17:161 - 71; http://dx.doi.org/10.1210/me.2002-0306; PMID: 12554744
- Buteau J. GLP-1 receptor signaling: effects on pancreatic beta-cell proliferation and survival. Diabetes Metab 2008; 34:Suppl 2 S73 - 7; http://dx.doi.org/10.1016/S1262-3636(08)73398-6; PMID: 18640589
- Dubé PE, Brubaker PL. Frontiers in glucagon-like peptide-2: multiple actions, multiple mediators. Am J Physiol Endocrinol Metab 2007; 293:E460 - 5; http://dx.doi.org/10.1152/ajpendo.00149.2007; PMID: 17652153
- Brun T, Hu He KH, Lupi R, Boehm B, Wojtusciszyn A, Sauter N, et al. The diabetes-linked transcription factor Pax4 is expressed in human pancreatic islets and is activated by mitogens and GLP-1. Hum Mol Genet 2008; 17:478 - 89; http://dx.doi.org/10.1093/hmg/ddm325; PMID: 17989064
- Xu G, Stoffers DA, Habener JF, Bonner-Weir S. Exendin-4 stimulates both beta-cell replication and neogenesis, resulting in increased beta-cell mass and improved glucose tolerance in diabetic rats. Diabetes 1999; 48:2270 - 6; http://dx.doi.org/10.2337/diabetes.48.12.2270; PMID: 10580413
- Stoffers DA, Kieffer TJ, Hussain MA, Drucker DJ, Bonner-Weir S, Habener JF, et al. Insulinotropic glucagon-like peptide 1 agonists stimulate expression of homeodomain protein IDX-1 and increase islet size in mouse pancreas. Diabetes 2000; 49:741 - 8; http://dx.doi.org/10.2337/diabetes.49.5.741; PMID: 10905482
- Bastien-Dionne PO, Valenti L, Kon N, Gu W, Buteau J. Glucagon-like peptide 1 inhibits the sirtuin deacetylase SirT1 to stimulate pancreatic β-cell mass expansion. Diabetes 2011; 60:3217 - 22; http://dx.doi.org/10.2337/db11-0101; PMID: 22013015
- Heller RS, Aponte GW. Intra-islet regulation of hormone secretion by glucagon-like peptide-1-(7--36) amide. Am J Physiol 1995; 269:G852 - 60; PMID: 8572216
- Masur K, Tibaduiza EC, Chen C, Ligon B, Beinborn M. Basal receptor activation by locally produced glucagon-like peptide-1 contributes to maintaining beta-cell function. Mol Endocrinol 2005; 19:1373 - 82; http://dx.doi.org/10.1210/me.2004-0350; PMID: 15677711
- Sangan CB, Tosh D. A new paradigm in cell therapy for diabetes: turning pancreatic α-cells into β-cells. Bioessays 2010; 32:881 - 4; http://dx.doi.org/10.1002/bies.201000074; PMID: 20803505
- Courtney M, Pfeifer A, Al-Hasani K, Gjernes E, Vieira A, Ben-Othman N, et al. In vivo conversion of adult α-cells into β-like cells: a new research avenue in the context of type 1 diabetes. Diabetes Obes Metab 2011; 13:Suppl 1 47 - 52; http://dx.doi.org/10.1111/j.1463-1326.2011.01441.x; PMID: 21824256
- Seymour PA, Sander M. Historical perspective: beginnings of the beta-cell: current perspectives in beta-cell development. Diabetes 2011; 60:364 - 76; http://dx.doi.org/10.2337/db10-1068; PMID: 21270248
- Gradwohl G, Dierich A, LeMeur M, Guillemot F. neurogenin3 is required for the development of the four endocrine cell lineages of the pancreas. Proc Natl Acad Sci U S A 2000; 97:1607 - 11; http://dx.doi.org/10.1073/pnas.97.4.1607; PMID: 10677506
- Johansson KA, Dursun U, Jordan N, Gu G, Beermann F, Gradwohl G, et al. Temporal control of neurogenin3 activity in pancreas progenitors reveals competence windows for the generation of different endocrine cell types. Dev Cell 2007; 12:457 - 65; http://dx.doi.org/10.1016/j.devcel.2007.02.010; PMID: 17336910
- Wilson ME, Kalamaras JA, German MS. Expression pattern of IAPP and prohormone convertase 1/3 reveals a distinctive set of endocrine cells in the embryonic pancreas. Mech Dev 2002; 115:171 - 6; http://dx.doi.org/10.1016/S0925-4773(02)00118-1; PMID: 12049785
- Harb G, Heremans Y, Heimberg H, Korbutt GS. Ectopic expression of neurogenin 3 in neonatal pig pancreatic precursor cells induces (trans)differentiation to functional alpha cells. Diabetologia 2006; 49:1855 - 63; http://dx.doi.org/10.1007/s00125-006-0299-z; PMID: 16736130
- Schwitzgebel VM, Scheel DW, Conners JR, Kalamaras J, Lee JE, Anderson DJ, et al. Expression of neurogenin3 reveals an islet cell precursor population in the pancreas. Development 2000; 127:3533 - 42; PMID: 10903178
- Herrera PL. Adult insulin- and glucagon-producing cells differentiate from two independent cell lineages. Development 2000; 127:2317 - 22; PMID: 10804174
- Hashimoto T, Kawano H, Daikoku S, Shima K, Taniguchi H, Baba S. Transient coappearance of glucagon and insulin in the progenitor cells of the rat pancreatic islets. Anat Embryol (Berl) 1988; 178:489 - 97; http://dx.doi.org/10.1007/BF00305036; PMID: 2464956
- De Krijger RR, Aanstoot HJ, Kranenburg G, Reinhard M, Visser WJ, Bruining GJ. The midgestational human fetal pancreas contains cells coexpressing islet hormones. Dev Biol 1992; 153:368 - 75; http://dx.doi.org/10.1016/0012-1606(92)90121-V; PMID: 1356859
- Teitelman G, Alpert S, Polak JM, Martinez A, Hanahan D. Precursor cells of mouse endocrine pancreas coexpress insulin, glucagon and the neuronal proteins tyrosine hydroxylase and neuropeptide Y, but not pancreatic polypeptide. Development 1993; 118:1031 - 9; PMID: 7903631
- Polak M, Bouchareb-Banaei L, Scharfmann R, Czernichow P. Early pattern of differentiation in the human pancreas. Diabetes 2000; 49:225 - 32; http://dx.doi.org/10.2337/diabetes.49.2.225; PMID: 10868939
- Bai L, Meredith G, Tuch BE. Glucagon-like peptide-1 enhances production of insulin in insulin-producing cells derived from mouse embryonic stem cells. J Endocrinol 2005; 186:343 - 52; http://dx.doi.org/10.1677/joe.1.06078; PMID: 16079260
- Sanz C, Blázquez E. New gene targets for glucagon-like peptide-1 during embryonic development and in undifferentiated pluripotent cells. Am J Physiol Endocrinol Metab 2011; 301:E494 - 503; http://dx.doi.org/10.1152/ajpendo.00116.2011; PMID: 21712536
- Rezania A, Riedel MJ, Wideman RD, Karanu F, Ao Z, Warnock GL, et al. Production of functional glucagon-secreting α-cells from human embryonic stem cells. Diabetes 2011; 60:239 - 47; http://dx.doi.org/10.2337/db10-0573; PMID: 20971966
- Liu Z, Habener JF. Glucagon-like peptide-1 activation of TCF7L2-dependent Wnt signaling enhances pancreatic beta cell proliferation. J Biol Chem 2008; 283:8723 - 35; http://dx.doi.org/10.1074/jbc.M706105200; PMID: 18216022
- Liu Z, Habener JF. Wnt signaling in pancreatic islets. Adv Exp Med Biol 2010; 654:391 - 419; http://dx.doi.org/10.1007/978-90-481-3271-3_17; PMID: 20217507
- Welters HJ, Kulkarni RN. Wnt signaling: relevance to beta-cell biology and diabetes. Trends Endocrinol Metab 2008; 19:349 - 55; http://dx.doi.org/10.1016/j.tem.2008.08.004; PMID: 18926717
- Thorel F, Népote V, Avril I, Kohno K, Desgraz R, Chera S, et al. Conversion of adult pancreatic alpha-cells to beta-cells after extreme beta-cell loss. Nature 2010; 464:1149 - 54; http://dx.doi.org/10.1038/nature08894; PMID: 20364121
- Chung CH, Hao E, Piran R, Keinan E, Levine F. Pancreatic β-cell neogenesis by direct conversion from mature α-cells. Stem Cells 2010; 28:1630 - 8; http://dx.doi.org/10.1002/stem.482; PMID: 20653050
- Lu J, Herrera PL, Carreira C, Bonnavion R, Seigne C, Calender A, et al. Alpha cell-specific Men1 ablation triggers the transdifferentiation of glucagon-expressing cells and insulinoma development. Gastroenterology 2010; 138:1954 - 65; http://dx.doi.org/10.1053/j.gastro.2010.01.046; PMID: 20138042
- Collombat P, Xu X, Ravassard P, Sosa-Pineda B, Dussaud S, Billestrup N, et al. The ectopic expression of Pax4 in the mouse pancreas converts progenitor cells into alpha and subsequently beta cells. Cell 2009; 138:449 - 62; http://dx.doi.org/10.1016/j.cell.2009.05.035; PMID: 19665969
- Collombat P, Hecksher-Sørensen J, Krull J, Berger J, Riedel D, Herrera PL, et al. Embryonic endocrine pancreas and mature beta cells acquire alpha and PP cell phenotypes upon Arx misexpression. J Clin Invest 2007; 117:961 - 70; http://dx.doi.org/10.1172/JCI29115; PMID: 17404619
- Dhawan S, Georgia S, Tschen SI, Fan G, Bhushan A. Pancreatic β cell identity is maintained by DNA methylation-mediated repression of Arx. Dev Cell 2011; 20:419 - 29; http://dx.doi.org/10.1016/j.devcel.2011.03.012; PMID: 21497756
- Liu Z, Habener JF. Alpha cells beget beta cells. Cell 2009; 138:424 - 6; http://dx.doi.org/10.1016/j.cell.2009.07.022; PMID: 19665963
- Sosa-Pineda B, Chowdhury K, Torres M, Oliver G, Gruss P. The Pax4 gene is essential for differentiation of insulin-producing beta cells in the mammalian pancreas. Nature 1997; 386:399 - 402; http://dx.doi.org/10.1038/386399a0; PMID: 9121556
- Collombat P, Mansouri A, Hecksher-Sorensen J, Serup P, Krull J, Gradwohl G, et al. Opposing actions of Arx and Pax4 in endocrine pancreas development. Genes Dev 2003; 17:2591 - 603; http://dx.doi.org/10.1101/gad.269003; PMID: 14561778
- Brun T, Gauthier BR. A focus on the role of Pax4 in mature pancreatic islet beta-cell expansion and survival in health and disease. J Mol Endocrinol 2008; 40:37 - 45; http://dx.doi.org/10.1677/JME-07-0134; PMID: 18234907
- Dor Y, Brown J, Martinez OI, Melton DA. Adult pancreatic beta-cells are formed by self-duplication rather than stem-cell differentiation. Nature 2004; 429:41 - 6; http://dx.doi.org/10.1038/nature02520; PMID: 15129273
- Nir T, Melton DA, Dor Y. Recovery from diabetes in mice by beta cell regeneration. J Clin Invest 2007; 117:2553 - 61; http://dx.doi.org/10.1172/JCI32959; PMID: 17786244
- Cano DA, Rulifson IC, Heiser PW, Swigart LB, Pelengaris S, German M, et al. Regulated beta-cell regeneration in the adult mouse pancreas. Diabetes 2008; 57:958 - 66; http://dx.doi.org/10.2337/db07-0913; PMID: 18083786
- Wang ZV, Mu J, Schraw TD, Gautron L, Elmquist JK, Zhang BB, et al. PANIC-ATTAC: a mouse model for inducible and reversible beta-cell ablation. Diabetes 2008; 57:2137 - 48; http://dx.doi.org/10.2337/db07-1631; PMID: 18469203
- Teta M, Rankin MM, Long SY, Stein GM, Kushner JA. Growth and regeneration of adult beta cells does not involve specialized progenitors. Dev Cell 2007; 12:817 - 26; http://dx.doi.org/10.1016/j.devcel.2007.04.011; PMID: 17488631
- Liu H, Guz Y, Kedees MH, Winkler J, Teitelman G. Precursor cells in mouse islets generate new beta-cells in vivo during aging and after islet injury. Endocrinology 2010; 151:520 - 8; http://dx.doi.org/10.1210/en.2009-0992; PMID: 20056825
- Smukler SR, Arntfield ME, Razavi R, Bikopoulos G, Karpowicz P, Seaberg R, et al. The adult mouse and human pancreas contain rare multipotent stem cells that express insulin. Cell Stem Cell 2011; 8:281 - 93; http://dx.doi.org/10.1016/j.stem.2011.01.015; PMID: 21362568
- Xu X, D’Hoker J, Stangé G, Bonné S, De Leu N, Xiao X, et al. Beta cells can be generated from endogenous progenitors in injured adult mouse pancreas. Cell 2008; 132:197 - 207; http://dx.doi.org/10.1016/j.cell.2007.12.015; PMID: 18243096
- Inada A, Nienaber C, Katsuta H, Fujitani Y, Levine J, Morita R, et al. Carbonic anhydrase II-positive pancreatic cells are progenitors for both endocrine and exocrine pancreas after birth. Proc Natl Acad Sci U S A 2008; 105:19915 - 9; http://dx.doi.org/10.1073/pnas.0805803105; PMID: 19052237
- Samols E, Stagner JI, Ewart RB, Marks V. The order of islet microvascular cellular perfusion is B----A----D in the perfused rat pancreas. J Clin Invest 1988; 82:350 - 3; http://dx.doi.org/10.1172/JCI113593; PMID: 2455737
- Franklin I, Gromada J, Gjinovci A, Theander S, Wollheim CB. Beta-cell secretory products activate alpha-cell ATP-dependent potassium channels to inhibit glucagon release. Diabetes 2005; 54:1808 - 15; http://dx.doi.org/10.2337/diabetes.54.6.1808; PMID: 15919803
- Menge BA, Grüber L, Jørgensen SM, Deacon CF, Schmidt WE, Veldhuis JD, et al. Loss of inverse relationship between pulsatile insulin and glucagon secretion in patients with type 2 diabetes. Diabetes 2011; 60:2160 - 8; http://dx.doi.org/10.2337/db11-0251; PMID: 21677283
- Kawamori D, Kurpad AJ, Hu J, Liew CW, Shih JL, Ford EL, et al. Insulin signaling in alpha cells modulates glucagon secretion in vivo. Cell Metab 2009; 9:350 - 61; http://dx.doi.org/10.1016/j.cmet.2009.02.007; PMID: 19356716
- Kawamori D, Akiyama M, Hu J, Hambro B, Kulkarni RN. Growth factor signalling in the regulation of α-cell fate. Diabetes Obes Metab 2011; 13:Suppl 1 21 - 30; http://dx.doi.org/10.1111/j.1463-1326.2011.01442.x; PMID: 21824253
- Furuta M, Yano H, Zhou A, Rouillé Y, Holst JJ, Carroll R, et al. Defective prohormone processing and altered pancreatic islet morphology in mice lacking active SPC2. Proc Natl Acad Sci U S A 1997; 94:6646 - 51; http://dx.doi.org/10.1073/pnas.94.13.6646; PMID: 9192619
- Webb GC, Akbar MS, Zhao C, Swift HH, Steiner DF. Glucagon replacement via micro-osmotic pump corrects hypoglycemia and alpha-cell hyperplasia in prohormone convertase 2 knockout mice. Diabetes 2002; 51:398 - 405; http://dx.doi.org/10.2337/diabetes.51.2.398; PMID: 11812747
- Vincent M, Guz Y, Rozenberg M, Webb G, Furuta M, Steiner D, et al. Abrogation of protein convertase 2 activity results in delayed islet cell differentiation and maturation, increased alpha-cell proliferation, and islet neogenesis. Endocrinology 2003; 144:4061 - 9; http://dx.doi.org/10.1210/en.2003-0088; PMID: 12933680
- Hayashi Y, Yamamoto M, Mizoguchi H, Watanabe C, Ito R, Yamamoto S, et al. Mice deficient for glucagon gene-derived peptides display normoglycemia and hyperplasia of islet alpha-cells but not of intestinal L-cells. Mol Endocrinol 2009; 23:1990 - 9; http://dx.doi.org/10.1210/me.2009-0296; PMID: 19819987
- Sloop KW, Cao JX, Siesky AM, Zhang HY, Bodenmiller DM, Cox AL, et al. Hepatic and glucagon-like peptide-1-mediated reversal of diabetes by glucagon receptor antisense oligonucleotide inhibitors. J Clin Invest 2004; 113:1571 - 81; PMID: 15173883
- Gu W, Yan H, Winters KA, Komorowski R, Vonderfecht S, Atangan L, et al. Long-term inhibition of the glucagon receptor with a monoclonal antibody in mice causes sustained improvement in glycemic control, with reversible alpha-cell hyperplasia and hyperglucagonemia. J Pharmacol Exp Ther 2009; 331:871 - 81; http://dx.doi.org/10.1124/jpet.109.157685; PMID: 19720878
- Winzell MS, Brand CL, Wierup N, Sidelmann UG, Sundler F, Nishimura E, et al. Glucagon receptor antagonism improves islet function in mice with insulin resistance induced by a high-fat diet. Diabetologia 2007; 50:1453 - 62; http://dx.doi.org/10.1007/s00125-007-0675-3; PMID: 17479245
- Gelling RW, Du XQ, Dichmann DS, Romer J, Huang H, Cui L, et al. Lower blood glucose, hyperglucagonemia, and pancreatic alpha cell hyperplasia in glucagon receptor knockout mice. Proc Natl Acad Sci U S A 2003; 100:1438 - 43; http://dx.doi.org/10.1073/pnas.0237106100; PMID: 12552113
- Vuguin PM, Kedees MH, Cui L, Guz Y, Gelling RW, Nejathaim M, et al. Ablation of the glucagon receptor gene increases fetal lethality and produces alterations in islet development and maturation. Endocrinology 2006; 147:3995 - 4006; http://dx.doi.org/10.1210/en.2005-1410; PMID: 16627579
- Kedees MH, Guz Y, Vuguin PM, Vargas C, Cui L, Steiner DF, et al. Nestin expression in pancreatic endocrine and exocrine cells of mice lacking glucagon signaling. Dev Dyn 2007; 236:1126 - 33; http://dx.doi.org/10.1002/dvdy.21112; PMID: 17366624
- Conarello SL, Jiang G, Mu J, Li Z, Woods J, Zycband E, et al. Glucagon receptor knockout mice are resistant to diet-induced obesity and streptozotocin-mediated beta cell loss and hyperglycaemia. Diabetologia 2007; 50:142 - 50; http://dx.doi.org/10.1007/s00125-006-0481-3; PMID: 17131145
- Chen M, Gavrilova O, Zhao WQ, Nguyen A, Lorenzo J, Shen L, et al. Increased glucose tolerance and reduced adiposity in the absence of fasting hypoglycemia in mice with liver-specific Gs alpha deficiency. J Clin Invest 2005; 115:3217 - 27; http://dx.doi.org/10.1172/JCI24196; PMID: 16239968
- Kilimnik G, Kim A, Steiner DF, Friedman TC, Hara M. Intraislet production of GLP-1 by activation of prohormone convertase 1/3 in pancreatic α-cells in mouse models of ß-cell regeneration. Islets 2010; 2:149 - 55; http://dx.doi.org/10.4161/isl.2.3.11396; PMID: 20657753
- Gu W, Winters KA, Motani AS, Komorowski R, Zhang Y, Liu Q, et al. Glucagon receptor antagonist-mediated improvements in glycemic control are dependent on functional pancreatic GLP-1 receptor. Am J Physiol Endocrinol Metab 2010; 299:E624 - 32; http://dx.doi.org/10.1152/ajpendo.00102.2010; PMID: 20647556
- Whalley NM, Pritchard LE, Smith DM, White A. Processing of proglucagon to GLP-1 in pancreatic α-cells: is this a paracrine mechanism enabling GLP-1 to act on β-cells?. J Endocrinol 2011; 211:99 - 106; http://dx.doi.org/10.1530/JOE-11-0094; PMID: 21795304
- Liu Z, Stanojevic V, Avadhani S, Yano T, Habener JF. Stromal cell-derived factor-1 (SDF-1)/chemokine (C-X-C motif) receptor 4 (CXCR4) axis activation induces intra-islet glucagon-like peptide-1 (GLP-1) production and enhances beta cell survival. Diabetologia 2011; 54:2067 - 76; http://dx.doi.org/10.1007/s00125-011-2181-x; PMID: 21567300
- Gu W, Lloyd DJ, Chinookswong N, Komorowski R, Sivits G Jr., Graham M, et al. Pharmacological targeting of glucagon and glucagon-like peptide 1 receptors has different effects on energy state and glucose homeostasis in diet-induced obese mice. J Pharmacol Exp Ther 2011; 338:70 - 81; http://dx.doi.org/10.1124/jpet.111.179986; PMID: 21471191
- Sørensen H, Winzell MS, Brand CL, Fosgerau K, Gelling RW, Nishimura E, et al. Glucagon receptor knockout mice display increased insulin sensitivity and impaired beta-cell function. Diabetes 2006; 55:3463 - 9; http://dx.doi.org/10.2337/db06-0307; PMID: 17130493
- Ma X, Zhang Y, Gromada J, Sewing S, Berggren PO, Buschard K, et al. Glucagon stimulates exocytosis in mouse and rat pancreatic alpha-cells by binding to glucagon receptors. Mol Endocrinol 2005; 19:198 - 212; http://dx.doi.org/10.1210/me.2004-0059; PMID: 15459251
- Kieffer TJ, Heller RS, Unson CG, Weir GC, Habener JF. Distribution of glucagon receptors on hormone-specific endocrine cells of rat pancreatic islets. Endocrinology 1996; 137:5119 - 25; http://dx.doi.org/10.1210/en.137.11.5119; PMID: 8895386
- Peshavaria M, Gamer L, Henderson E, Teitelman G, Wright CV, Stein R. XIHbox 8, an endoderm-specific Xenopus homeodomain protein, is closely related to a mammalian insulin gene transcription factor. Mol Endocrinol 1994; 8:806 - 16; http://dx.doi.org/10.1210/me.8.6.806; PMID: 7935494
- Yano T, Liu Z, Donovan J, Thomas MK, Habener JF. Stromal cell derived factor-1 (SDF-1)/CXCL12 attenuates diabetes in mice and promotes pancreatic beta-cell survival by activation of the prosurvival kinase Akt. Diabetes 2007; 56:2946 - 57; http://dx.doi.org/10.2337/db07-0291; PMID: 17878289
- Nie Y, Nakashima M, Brubaker PL, Li QL, Perfetti R, Jansen E, et al. Regulation of pancreatic PC1 and PC2 associated with increased glucagon-like peptide 1 in diabetic rats. J Clin Invest 2000; 105:955 - 65; http://dx.doi.org/10.1172/JCI7456; PMID: 10749575
- Jones CW, Reynolds WA, Hoganson GE. Streptozotocin diabetes in the monkey: plasma levels of glucose, insulin, glucagon, and somatostatin, with corresponding morphometric analysis of islet endocrine cells. Diabetes 1980; 29:536 - 46; http://dx.doi.org/10.2337/diabetes.29.7.536; PMID: 6103856
- Li Z, Karlsson FA, Sandler S. Islet loss and alpha cell expansion in type 1 diabetes induced by multiple low-dose streptozotocin administration in mice. J Endocrinol 2000; 165:93 - 9; http://dx.doi.org/10.1677/joe.0.1650093; PMID: 10750039
- Guardado-Mendoza R, Davalli AM, Chavez AO, Hubbard GB, Dick EJ, Majluf-Cruz A, et al. Pancreatic islet amyloidosis, beta-cell apoptosis, and alpha-cell proliferation are determinants of islet remodeling in type-2 diabetic baboons. Proc Natl Acad Sci U S A 2009; 106:13992 - 7; http://dx.doi.org/10.1073/pnas.0906471106; PMID: 19666551
- Ellingsgaard H, Ehses JA, Hammar EB, Van Lommel L, Quintens R, Martens G, et al. Interleukin-6 regulates pancreatic alpha-cell mass expansion. Proc Natl Acad Sci U S A 2008; 105:13163 - 8; http://dx.doi.org/10.1073/pnas.0801059105; PMID: 18719127
- Ogawa N, List JF, Habener JF, Maki T. Cure of overt diabetes in NOD mice by transient treatment with anti-lymphocyte serum and exendin-4. Diabetes 2004; 53:1700 - 5; http://dx.doi.org/10.2337/diabetes.53.7.1700; PMID: 15220193
- Meier JJ, Ueberberg S, Korbas S, Schneider S. Diminished glucagon suppression after β-cell reduction is due to impaired α-cell function rather than an expansion of α-cell mass. Am J Physiol Endocrinol Metab 2011; 300:E717 - 23; http://dx.doi.org/10.1152/ajpendo.00315.2010; PMID: 21285404
- Waguri M, Hanafusa T, Itoh N, Miyagawa J, Imagawa A, Kuwajima M, et al. Histopathologic study of the pancreas shows a characteristic lymphocytic infiltration in Japanese patients with IDDM. Endocr J 1997; 44:23 - 33; http://dx.doi.org/10.1507/endocrj.44.23; PMID: 9152611
- Henquin JC, Rahier J. Pancreatic alpha cell mass in European subjects with type 2 diabetes. Diabetologia 2011; 54:1720 - 5; http://dx.doi.org/10.1007/s00125-011-2118-4; PMID: 21465328
- Deng S, Vatamaniuk M, Huang X, Doliba N, Lian MM, Frank A, et al. Structural and functional abnormalities in the islets isolated from type 2 diabetic subjects. Diabetes 2004; 53:624 - 32; http://dx.doi.org/10.2337/diabetes.53.3.624; PMID: 14988246
- Willcox A, Richardson SJ, Bone AJ, Foulis AK, Morgan NG. Evidence of increased islet cell proliferation in patients with recent-onset type 1 diabetes. Diabetologia 2010; 53:2020 - 8; http://dx.doi.org/10.1007/s00125-010-1817-6; PMID: 20532863
- Kedees MH, Grigoryan M, Guz Y, Teitelman G. Differential expression of glucagon and glucagon-like peptide 1 receptors in mouse pancreatic alpha and beta cells in two models of alpha cell hyperplasia. Mol Cell Endocrinol 2009; 311:69 - 76; http://dx.doi.org/10.1016/j.mce.2009.07.024; PMID: 19647035
- Wideman RD, Covey SD, Webb GC, Drucker DJ, Kieffer TJ. A switch from prohormone convertase (PC)-2 to PC1/3 expression in transplanted alpha-cells is accompanied by differential processing of proglucagon and improved glucose homeostasis in mice. Diabetes 2007; 56:2744 - 52; http://dx.doi.org/10.2337/db07-0563; PMID: 17698597
- Riedel MJ, Asadi A, Wang R, Ao Z, Warnock GL, Kieffer TJ. Immunohistochemical characterisation of cells co-producing insulin and glucagon in the developing human pancreas. Diabetologia 2012; 55:372 - 81; http://dx.doi.org/10.1007/s00125-011-2344-9; PMID: 22038519
- Thyssen S, Arany E, Hill DJ. Ontogeny of regeneration of beta-cells in the neonatal rat after treatment with streptozotocin. Endocrinology 2006; 147:2346 - 56; http://dx.doi.org/10.1210/en.2005-0396; PMID: 16484329
- Hansen AM, Bödvarsdottir TB, Nordestgaard DN, Heller RS, Gotfredsen CF, Maedler K, et al. Upregulation of alpha cell glucagon-like peptide 1 (GLP-1) in Psammomys obesus--an adaptive response to hyperglycaemia?. Diabetologia 2011; 54:1379 - 87; http://dx.doi.org/10.1007/s00125-011-2080-1; PMID: 21347622
- Furuta M, Zhou A, Webb G, Carroll R, Ravazzola M, Orci L, et al. Severe defect in proglucagon processing in islet A-cells of prohormone convertase 2 null mice. J Biol Chem 2001; 276:27197 - 202; http://dx.doi.org/10.1074/jbc.M103362200; PMID: 11356850
- Webb GC, Dey A, Wang J, Stein J, Milewski M, Steiner DF. Altered proglucagon processing in an alpha-cell line derived from prohormone convertase 2 null mouse islets. J Biol Chem 2004; 279:31068 - 75; http://dx.doi.org/10.1074/jbc.M404110200; PMID: 15143067
- Wideman RD, Gray SL, Covey SD, Webb GC, Kieffer TJ. Transplantation of PC1/3-Expressing alpha-cells improves glucose handling and cold tolerance in leptin-resistant mice. Mol Ther 2009; 17:191 - 8; http://dx.doi.org/10.1038/mt.2008.219; PMID: 18941442
- Heller RS, Kieffer TJ, Habener JF. Insulinotropic glucagon-like peptide I receptor expression in glucagon-producing alpha-cells of the rat endocrine pancreas. Diabetes 1997; 46:785 - 91; http://dx.doi.org/10.2337/diabetes.46.5.785; PMID: 9133545
- Moens K, Heimberg H, Flamez D, Huypens P, Quartier E, Ling Z, et al. Expression and functional activity of glucagon, glucagon-like peptide I, and glucose-dependent insulinotropic peptide receptors in rat pancreatic islet cells. Diabetes 1996; 45:257 - 61; http://dx.doi.org/10.2337/diabetes.45.2.257; PMID: 8549871
- Tornehave D, Kristensen P, Rømer J, Knudsen LB, Heller RS. Expression of the GLP-1 receptor in mouse, rat, and human pancreas. J Histochem Cytochem 2008; 56:841 - 51; http://dx.doi.org/10.1369/jhc.2008.951319; PMID: 18541709
- Kayali AG, Van Gunst K, Campbell IL, Stotland A, Kritzik M, Liu G, et al. The stromal cell-derived factor-1alpha/CXCR4 ligand-receptor axis is critical for progenitor survival and migration in the pancreas. J Cell Biol 2003; 163:859 - 69; http://dx.doi.org/10.1083/jcb.200304153; PMID: 14638861
- Dorrell C, Schug J, Lin CF, Canaday PS, Fox AJ, Smirnova O, et al. Transcriptomes of the major human pancreatic cell types. Diabetologia 2011; 54:2832 - 44; http://dx.doi.org/10.1007/s00125-011-2283-5; PMID: 21882062
- Petri A, Ahnfelt-Rønne J, Frederiksen KS, Edwards DG, Madsen D, Serup P, et al. The effect of neurogenin3 deficiency on pancreatic gene expression in embryonic mice. J Mol Endocrinol 2006; 37:301 - 16; http://dx.doi.org/10.1677/jme.1.02096; PMID: 17032746
- Brunicardi FC, Stagner J, Bonner-Weir S, Wayland H, Kleinman R, Livingston E, et al, Long Beach Veterans Administration Regional Medical Education Center Symposium. Microcirculation of the islets of Langerhans. Diabetes 1996; 45:385 - 92; PMID: 8603757
- Jain R, Lammert E. Cell-cell interactions in the endocrine pancreas. Diabetes Obes Metab 2009; 11:Suppl 4 159 - 67; http://dx.doi.org/10.1111/j.1463-1326.2009.01102.x; PMID: 19817798
- Unger RH, Orci L. Paracrinology of islets and the paracrinopathy of diabetes. Proc Natl Acad Sci U S A 2010; 107:16009 - 12; http://dx.doi.org/10.1073/pnas.1006639107; PMID: 20798346
- Cabrera O, Berman DM, Kenyon NS, Ricordi C, Berggren PO, Caicedo A. The unique cytoarchitecture of human pancreatic islets has implications for islet cell function. Proc Natl Acad Sci U S A 2006; 103:2334 - 9; http://dx.doi.org/10.1073/pnas.0510790103; PMID: 16461897
- Steiner DJ, Kim A, Miller K, Hara M. Pancreatic islet plasticity: interspecies comparison of islet architecture and composition. Islets 2010; 2:135 - 45; http://dx.doi.org/10.4161/isl.2.3.11815; PMID: 20657742
- Lau TT, Wang DA. Stromal cell-derived factor-1 (SDF-1): homing factor for engineered regenerative medicine. Expert Opin Biol Ther 2011; 11:189 - 97; http://dx.doi.org/10.1517/14712598.2011.546338; PMID: 21219236
- Ratajczak MZ, Zuba-Surma E, Kucia M, Reca R, Wojakowski W, Ratajczak J. The pleiotropic effects of the SDF-1-CXCR4 axis in organogenesis, regeneration and tumorigenesis. Leukemia 2006; 20:1915 - 24; http://dx.doi.org/10.1038/sj.leu.2404357; PMID: 16900209
- Liu Z, Habener JF. Stromal cell-derived factor-1 promotes survival of pancreatic beta cells by the stabilisation of beta-catenin and activation of transcription factor 7-like 2 (TCF7L2). Diabetologia 2009; 52:1589 - 98; http://dx.doi.org/10.1007/s00125-009-1384-x; PMID: 19468708
- Ellingsgaard H, Hauselmann I, Schuler B, Habib AM, Baggio LL, Meier DT, et al. Interleukin-6 enhances insulin secretion by increasing glucagon-like peptide-1 secretion from L cells and alpha cells. Nat Med 2011; 17:1481 - 9; http://dx.doi.org/10.1038/nm.2513; PMID: 22037645
- Liang XD, Guo YY, Sun M, Ding Y, Wang N, Yuan L, et al. Streptozotocin-induced expression of Ngn3 and Pax4 in neonatal rat pancreatic α-cells. World J Gastroenterol 2011; 17:2812 - 20; PMID: 21734788
- Chen S, Shimoda M, Chen J, Matsumoto S, Grayburn PA. Transient overexpression of cyclin D2/CDK4/GLP1 genes induces proliferation and differentiation of adult pancreatic progenitors and mediates islet regeneration. Cell Cycle 2012; 11:695 - 705; http://dx.doi.org/10.4161/cc.11.4.19120; PMID: 22373529
- Wideman RD, Yu IL, Webber TD, Verchere CB, Johnson JD, Cheung AT, et al. Improving function and survival of pancreatic islets by endogenous production of glucagon-like peptide 1 (GLP-1). Proc Natl Acad Sci U S A 2006; 103:13468 - 73; http://dx.doi.org/10.1073/pnas.0600655103; PMID: 16938896
- Gosmain Y, Cheyssac C, Heddad Masson M, Dibner C, Philippe J. Glucagon gene expression in the endocrine pancreas: the role of the transcription factor Pax6 in α-cell differentiation, glucagon biosynthesis and secretion. Diabetes Obes Metab 2011; 13:Suppl 1 31 - 8; http://dx.doi.org/10.1111/j.1463-1326.2011.01445.x; PMID: 21824254
- Artner I, Le Lay J, Hang Y, Elghazi L, Schisler JC, Henderson E, et al. MafB: an activator of the glucagon gene expressed in developing islet alpha- and beta-cells. Diabetes 2006; 55:297 - 304; http://dx.doi.org/10.2337/diabetes.55.02.06.db05-0946; PMID: 16443760
- Smith SB, Gasa R, Watada H, Wang J, Griffen SC, German MS. Neurogenin3 and hepatic nuclear factor 1 cooperate in activating pancreatic expression of Pax4. J Biol Chem 2003; 278:38254 - 9; http://dx.doi.org/10.1074/jbc.M302229200; PMID: 12837760
- Russ HA, Bar Y, Ravassard P, Efrat S. In vitro proliferation of cells derived from adult human beta-cells revealed by cell-lineage tracing. Diabetes 2008; 57:1575 - 83; http://dx.doi.org/10.2337/db07-1283; PMID: 18316362
- Russ HA, Ravassard P, Kerr-Conte J, Pattou F, Efrat S. Epithelial-mesenchymal transition in cells expanded in vitro from lineage-traced adult human pancreatic beta cells. PLoS One 2009; 4:e6417; http://dx.doi.org/10.1371/journal.pone.0006417; PMID: 19641613
- Russ HA, Sintov E, Anker-Kitai L, Friedman O, Lenz A, Toren G, et al. Insulin-producing cells generated from dedifferentiated human pancreatic beta cells expanded in vitro. PLoS One 2011; 6:e25566; http://dx.doi.org/10.1371/journal.pone.0025566; PMID: 21984932
- Hanley S, Rosenberg L. Islet-derived progenitors as a source of in vitro islet regeneration. Methods Mol Biol 2009; 482:371 - 85; http://dx.doi.org/10.1007/978-1-59745-060-7_23; PMID: 19089368
- Joglekar MV, Hardikar AA. Epithelial-to-mesenchymal transition in pancreatic islet beta cells. Cell Cycle 2010; 9:4077 - 9; http://dx.doi.org/10.4161/cc.9.20.13590; PMID: 20948307
- Blyszczuk P, Czyz J, Kania G, Wagner M, Roll U, St-Onge L, et al. Expression of Pax4 in embryonic stem cells promotes differentiation of nestin-positive progenitor and insulin-producing cells. Proc Natl Acad Sci U S A 2003; 100:998 - 1003; http://dx.doi.org/10.1073/pnas.0237371100; PMID: 12525695
- Wang J, Elghazi L, Parker SE, Kizilocak H, Asano M, Sussel L, et al. The concerted activities of Pax4 and Nkx2.2 are essential to initiate pancreatic beta-cell differentiation. Dev Biol 2004; 266:178 - 89; http://dx.doi.org/10.1016/j.ydbio.2003.10.018; PMID: 14729487
- Lin HT, Kao CL, Lee KH, Chang YL, Chiou SH, Tsai FT, et al. Enhancement of insulin-producing cell differentiation from embryonic stem cells using pax4-nucleofection method. World J Gastroenterol 2007; 13:1672 - 9; PMID: 17461469
- Liew CG, Shah NN, Briston SJ, Shepherd RM, Khoo CP, Dunne MJ, et al. PAX4 enhances beta-cell differentiation of human embryonic stem cells. PLoS One 2008; 3:e1783; http://dx.doi.org/10.1371/journal.pone.0001783; PMID: 18335054
- Brink C, Gruss P. DNA sequence motifs conserved in endocrine promoters are essential for Pax4 expression. Dev Dyn 2003; 228:617 - 22; http://dx.doi.org/10.1002/dvdy.10405; PMID: 14648838