Abstract
The function of steroid receptors is not only regulated by steroid hormones, but also by multiple cellular signaling cascades activated by membrane-bound receptors which are stimulated by growth factors or cytokines. Cross-talk between JAK and steroid receptors plays a central role in the regulation of a multitude of physiological processes and aberrant signaling is involved in the development of numerous diseases including cancer. In this review we provide a brief summary of the knowledge of interactions between JAK and the function of steroid receptors in normal cells and tissues and in diseases.
Introduction
Janus kinases (JAKs) constitute a family of cytoplasmic non-receptor tyrosine kinases of which four family members have been identified in mammals, JAK1, JAK2, JAK3, and TYK2 (tyrosine kinase 2). Whereas JAK1, JAK2, and TYK2 are ubiquitously expressed, the expression of JAK3 is limited to hematopoietic cells. JAKs associate constitutively with a variety of cytokine and hormone receptors, and ligand binding to these receptors results in activation of JAK. The activated kinase subsequently phosphorylates specific tyrosine motifs in the receptor which in turn serve as docking sites for signaling molecules such as STATs, Src-kinase, protein phosphatases, and adaptor signaling proteins such as Shc, Grb2, and Cbl.Citation1 Consequently, an intricate cross-talk between JAK and other signal transduction pathways occurs in mammalian cells.Citation2-Citation4
JAKs and the JAK-STAT pathways play essential roles in embryogenesis, development, and hematopoiesis. However, JAKs have also been found mutated and overexpressed in various malignancies and therefore have been attributed a critical role in malignant transformation of cells and in cancer progressionCitation5-Citation8 and strong efforts are being undertaken to generate selective inhibitors for the JAK subtypes to achieve therapeutic effects.Citation9,Citation10
Signaling pathways activated by cytokines consist of families of inherent signaling molecules, which are different from those activated by nuclear receptors. Nevertheless, convincing evidence has been accumulated in the last decade that cytokines, via activation of JAK and the JAK-STAT pathways, interact with signaling pathways that are triggered by nuclear receptors. Furthermore, ligands of nuclear receptors have been reported to be important regulators of cytokine production.Citation11 In this review we summarize briefly current knowledge on the interaction of JAK and JAK-related signaling pathways with steroids and steroid receptors with special emphasis on observations in tumors.
Steroid Hormones and Steroid Receptors
Steroid hormones occurring in vertebrates have been grouped into the sex steroids (estrogens, progestagens, and androgens), corticoids (glucocorticoids and mineralocorticoids), and in steroid-like compounds (vitamin D and its derivatives, retinoic acid, PPAR [peroxisome proliferator-activated receptor] agonists, and thyroid hormone). All steroids bind to specific receptors which show significant homology and form the superfamily of steroid receptors.Citation12,Citation13 Most of the natural steroid hormones are synthesized from cholesterol. The sex steroids are mainly produced in the gonads and to a small extent in the adrenal glands. Estrone and estradiol, the strongest natural estrogens, may also be produced at significant amounts in the adipose tissue.Citation14 Glucocorticoids and mineralocorticoids are products of the adrenal cortex. Conversions and catabolism of steroids occur mainly in the liver, but also in other peripheral tissues and in the target tissues. Steroid hormones help control metabolism, inflammation, immune functions, salt and water balance, development of sexual characteristics, and the ability to withstand illness and injury. Nevertheless, all classical steroid hormones, in particular the sex hormones, may be implicated in the development of diseases. For example, estrogens and androgens play a central role in the pathogenesis of breast and prostate cancer, respectively.
In the blood, steroid hormones are bound to specific carrier proteins such as sex hormone-binding globulin or corticosteroid-binding globulin. As lipophilic compounds they can pass through the cell membrane and then bind to the respective steroid hormone receptors. Most steroid receptors are members of the large superfamily of nuclear receptors and transcription factors.Citation12 This superfamily, on the one hand, has been categorized in six subfamilies according to sequence homology.Citation15 On the other hand, type I to IV receptors have been categorized according to DNA binding characteristics.Citation12,Citation16
Steroid hormone receptors may be nuclear or cytosolic or even membrane-bound.Citation17 In the inactive state cytosolic steroid receptors are bound to heat-shock proteins. Ligand binding results in the dissociation of heat shock proteins, homodimerization of the receptors, and translocation from the cytoplasm into the cell nucleus. There, they bind to specific sequences of DNA known as hormone response elements (HREs) in the promoter regions of target genes. Other proteins are recruited to the receptor/DNA complex and DNA transcription into mRNA is initiated. Four types of nuclear steroid receptors have been described depending on their mechanism of action. Type I nuclear receptors usually bind to HREs consisting ideally of two palindromic half-sites separated by a variable length of DNA, the second half-site being a sequence inverted from the first (inverted repeat). Type I nuclear receptors include the estrogen receptors, progesterone receptor, androgen receptor, mineralocorticoid receptor, and glucocorticoid receptor. Type II receptors are located in the nucleus regardless of the activation status and usually bound to direct repeat HREs in the DNA. In the unliganded state, type II nuclear receptors are usually complexed with corepressor proteins. Ligand binding causes dissociation of corepressor and recruitment of coactivator proteins. Type II nuclear receptors include the retinoic acid receptor, retinoid X receptor, and the thyroid hormone receptor.Citation18 Type III receptors are similar to type I receptors; both classes bind to DNA as homodimers. However, in contrast to type I receptors, type III receptors bind to direct repeat instead of inverted repeat HREs. The so-called “orphan nuclear receptors” have been categorized in the Type III class.Citation19 Type IV receptors can bind either as monomers or dimers. Such receptors are found in most of the nuclear receptor subfamilies. The high complexity of nuclear receptor/DNA binding has been reviewed previously.Citation20,Citation21
In contrast to the nuclear receptors which initiate genomic actions, i.e., activation or repression of gene expression, membrane-associated steroid receptors activate intracellular signaling cascades involved in rapid non-genomic actions.Citation17 Cell membrane associated steroid receptors include some estrogen receptor subtypes and G-protein-coupled receptors.Citation22 Characteristically, steroid hormone receptors undergo ligand-induced downregulation. This represents a physiologically important feedback mechanism to limit hormone action in target tissues.
JAK and Estrogen Receptor Interaction
Since the pioneering work of George Beatson in 1896,Citation23 different clinical, epidemiological, and animal studies have clearly established the cardinal role of estrogens in the pathogenesis of breast cancer. More importantly, estrogen receptor-α (ERα) has been identified as the key mediator of the tumorigenic action of estrogens in the breast and is a clinically reliable prognostic indicator of the disease.Citation24 Nevertheless, the signaling mechanisms involved in the regulation of ERα transcriptional activity are highly complex and continue to remain elusive. In the last decade it became evident that ERα activity is not only regulated by estrogens but also by ligand-independent mechanisms which involve protein kinases related to growth factor-activated signaling cascades.Citation25-Citation28 Considering the importance of protein kinases in the regulation of intracellular signal transduction pathways, our group performed a kinome-wide high-throughput siRNA screening in an ERα-positive breast cancer cell line and identified JAK2 as a novel negative regulator of ERα activity.Citation29 Investigation of the underlying molecular mechanism elucidated a role of JAK2 in promoting ubiquitin-dependent proteasomal degradation of ERα. In addition, we found an upregulation of JAK2 expression upon prolonged estradiol treatment, which suggested a potential physiological function of this kinase in governing the autoregulation of ERα expression level in hormone responsive tissues. Other JAK isoforms were not related to this mechanism. The potential role of STATs in this negative effect of JAK2 on ERα remains to be clarified. Interestingly, we found JAK2 to be a target of miR-375 (Gupta N, unpublished data), a micro-RNA which has been previously reported to be upregulated in breast cancer cells, functioning as a positive regulator of ERα.Citation30 This observation suggests an even higher complexity of JAK2/ERα interaction involving the fine-tuning by a micro-RNA ().
Figure 1. JAK2 is a negative regulator of ERα. JAK2 negatively regulates ERα level by initiating a signaling pathway that results in degradation of ERα via the proteasome. In the presence of E2, JAK2 is induced. Increasing JAK2 levels may play a role in limiting the ERα level and thereby regulate E2 responsiveness in target tissues.Citation29 In addition, JAK2 is a target for miR-375, a miRNA which positively regulates ERα.Citation30 This suggests a complex regulation of the JAK2 and ERα interaction.
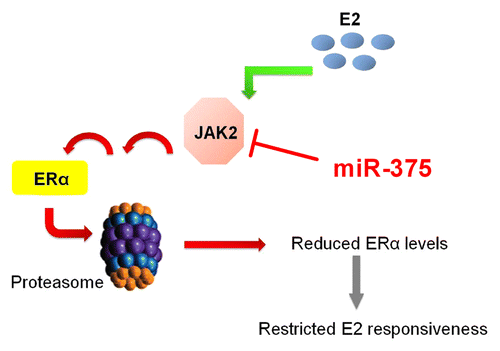
In a clinical study with infiltrating ductal breast carcinomas, Yeh et al. observed a reduction in the expression of active phosphorylated JAK1 (p-JAK1) which significantly correlated with ER positive status and increased tumor size.Citation31 Further, by using ERα-positive and negative cell lines as well as RNAi interference, these authors established the inverse relation between p-JAK1 and ERα which in turn underscored the importance of p-JAK1 in the development of infiltrating ductal carcinoma. Interestingly, in context of the mitogenic role of leptin in breast tumorigenesisCitation32,Citation33 the JAK kinase has been reported to exert a positive regulatory effect on estrogen signaling. Upon binding to its receptor on the surface of epithelial breast cancer cells, leptin induces JAK-mediated tyrosine phosphorylation and activation of the downstream targets STAT3 and MAPK. Whereas STAT3 induces the expression of aromatase and upregulates estradiol production, MAPK inhibits the proteasomal degradation of ERα.Citation34
An intricate transcriptional crosstalk between nuclear receptors and cytokine signaling is increasingly gaining prominence in the immune system. Furthermore, growth hormone (GH) regulates somatic growth and carbohydrate and lipid metabolismCitation35 through a transmembrane receptor which is a member of the cytokine receptor superfamily and is coupled to JAK-STAT signaling.Citation36 Leung et al. showed that estrogen inhibited GH-induced tyrosine phosphorylation of JAK2 and consequently, reduced STAT3 and STAT5 transcriptional activity in HEK293 cells stably expressing the GH receptor, as well as in T-47D breast cancer cells and HuH-7 hepatoma cells.Citation37 The effect was mediated by upregulation of the expression of SOCS2, a well-established negative regulator of the JAK-STAT pathway. The same group showed later that the selective estrogen receptor modulators 4-hydroxytamoxifen and raloxifen augmented GH signaling in HEK293 cells.Citation38
Similar to GH, the luteotropic hormone prolactin (PRL) which is essential for the establishment and maintenance of pregnancy exerts its biological action through a cytokine receptor.Citation39 In the ovarian corpus luteum, PRL activates the JAK2-STAT5 pathway and upregulates ERα expression, which in turn transduces the reproductive functions of estrogen. In addition, PRL also increases the level of luteinizing hormone that is necessary for endogenous production of estradiol and androgens.Citation40,Citation41 Interestingly, Zhao et al. showed that in human adipocytes, the cytokines IL-11, IL-6, LIF, and oncostatin M activate the JAK1-STAT3 pathway to induce the expression of aromatase P450Citation42 which catalyzes the last step in the biosynthesis of estrogens. Since adipose tissue is the major site for postmenopausal estrogen production, JAK1 seems to have the potential to influence hormone levels in elderly women.
Taken together, an intriguingly complex and diverse crosstalk exists between estrogens, ERα, and JAK, which is subject to species and tissue specific variation and is strongly influenced by the upstream signals.
JAK and Progesterone Interaction
Progesterone is mainly produced in the corpus luteum during the menstrual cycle and in the placenta during pregnancy in women and to a minor extent in the testis in men. It promotes proliferation and differentiation of mammary gland epithelium and has an essential contribution to the development and progression of breast cancer.Citation43 The tumorigenic effect of progesterone has been partly attributed to the multi-level intricate cross-talk of the ovarian steroid with growth factors and cytokine signaling pathways. Interestingly, treatment of T-47D breast cancer cells with synthetic progesterone induces tyrosine phosphorylation of JAK2 and an increase in STAT5 protein level. In addition, progesterone potentiates EGF signaling by upregulating the EGF receptors and activating the downstream signaling molecules in the breast cancer cells. This cross-talk between progesterone and JAK-STAT pathway/EGF signaling has been reviewed in detail by Lange et al.Citation44 In 2005, Proietti et al. further investigated the role of progesterone in the regulation of the JAK-STAT pathway in breast cancer.Citation45 The study used an elegant experimental model where the synthetic progesterone medroxyprogesterone acetate (MPA) was used to induce mammary adenocarcinoma in Balb/c mice. MPA treatment triggered tyrosine phosphorylation of JAK1 and JAK2 and activated the downstream target STAT3. Additionally, progesterone upregulated the activity and expression of STAT3 and stimulated breast cancer growth; this could be blocked by inactivation of JAKs.
Only recently, a unique role of progesterone has been reported in human spermatozoa.Citation46 Capacitation or the acquisition of sperm fertilizing ability involves the activation of the JAK-STAT pathway.Citation47,Citation48 Progesterone was found to trigger tyrosine phosphorylation of JAK1, JAK2, and STAT1 in a time-dependent manner and the phosphorylation pattern of JAK1 and JAK2 being different in different regions of the sperm, and thereby influence the normal physiological function of spermatozoa.
JAK and Androgen Receptor Interaction
Only little is known about the interaction between JAK and androgen receptor (AR). Androgens are mainly produced in the testis and to a minor extent in the adrenal gland and the prostate and they have long been implicated in the pathogenesis of human prostate cancer.Citation49 In addition, activation of the androgen receptor and increased androgen signaling play a role in the carcinogenesis in other tissues such as the liver and the pancreas. Ueda et al. discovered an interesting cross-talk between IL-6 and AR signaling in LNCaP prostate cancer cells resulting in AR activation and increased AR-driven reporter gene expression.Citation50 Interestingly, IL-6 induced the phosphorylation/activation of mitogen-activated protein kinase (MAPK) and activation of the N-terminal domain of the AR. IL-6 also activated STAT3, probably by direct phosphorylation of STAT3 by JAK. Activated STAT3 directly interacted with the AR N-terminal domain. Moreover, in hepatitis C virus positive hepatocellular carcinomaCitation51 and in pancreatic cancer,Citation52 JAK along with STAT3 has been implicated in the activation and function of the AR. In contrast, Martinez et al. suggested a negative effect of JAK2 on androgen receptor activity in LNCaP cells.Citation53 These authors performed a mass spectrometry-based quantitative proteomic analysis to identify androgen regulated protein networks that control cell proliferation, survival and differentiation in LNCaP cells. JAK2 was identified as a novel androgen-sensitive microsome-associated protein that was part of a whole network of androgen-sensitive microsome-associated proteins which also included MAPK and phosphatidylinositol 3-kinase (PI3K). JAK2 modulated the expression of prostate epithelial and neuronal markers and co-regulated AR-mediated transcription. Importantly, JAK2 inhibited the mTOR pathway and downregulated cell proliferation in an AR-dependent fashion. The contradictory results regarding the role of JAK2 in the regulation of AR activity remains elusive. The high complexity of this molecular framework may be of relevance in the regulation of attenuation or stimulation of prostate cancer growth.
JAK and Aldosterone Interaction
Aldosterone is a steroid of the adrenal cortex. Adrenal steroidogenesis is primarily regulated by the hypothalamic adrenocorticotropic hormone (ACTH) via the cAMP/PKA signaling pathway. Lefrancois-Martinez et al. uncovered an interesting novel link between PKA and STAT5-independent nuclear JAK2 signaling in rat adrenal cortical cells.Citation54 Nuclear JAK2 induced tyrosine phosphorylation and prevented the proteasomal degradation of the transcription factor cAMP response element-binding protein (CREB); leading to increased stability of CREB and expression of CREB regulated steroidogenic target genes.
As an integral part of the renin-angiotensin system aldosterone plays a central role in the regulation of hemodynamic stability.Citation55 The active octapeptide angiotensin II, produced from angiotensin I by the angiotensin converting enzyme (ACE), stimulates the production of aldosterone by inducing the rate-limiting steroidogenic acute regulatory protein (StAR). Two independent groups reported angiotensin II-induced phosphorylation and activation of JAK2 in vascular smooth muscle cellsCitation56 and in H295R human adrenocortical cells,Citation57 respectively. The latter study further demonstrated the inhibitory effect of JAK2 blockage on StAR reporter gene activity and steroid production, thereby, once more, highlighting and confirming the importance of JAK2 in adrenal steroidogenesis. Interestingly, aldosterone was found to stimulate the expression and activity of ACE through a JAK2-dependent pathway in rat aortic endothelial cells.Citation58 This indicates the presence of a positive autoregulatory loop in the renin-angiotensin-aldosterone system where JAK2 seems to play an integral role ().
Figure 2. JAK2 is a key player in the renin-angiotensin-aldosterone autoregulation. Angiotensin II, produced from angiotensin I by the angiotensin converting enzyme (ACE), stimulates the production of aldosterone by inducing the rate-limiting steroidogenic acute regulatory protein (StAR). Aldosterone stimulates ACE expression through a JAK2-dependent mechanism. Furthermore, angiotensin II induces phosphorylation and activation of JAK2 which then activates StAR expression. This indicates the presence of a positive autoregulatory loop in the renin-angiotensin-aldosterone system where JAK2 seems to play an integral role.
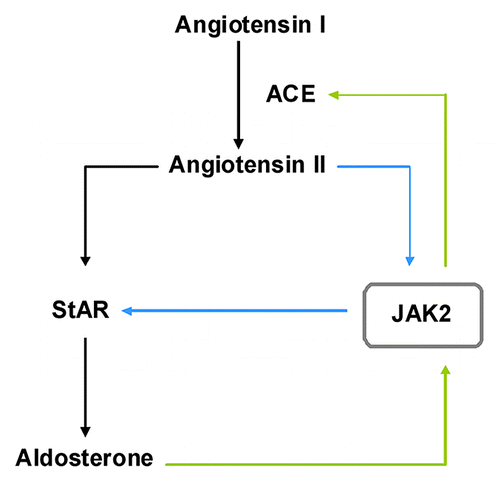
An increased level of circulatory very low density lipoproteins (VLDL) is a characteristic feature of diabetic dyslipedemia. Recently, JAK2 has been reported to be involved in VLDL-induced transcriptional regulation of aldosterone synthase (Cyp11B2).Citation59 The elevated level of the steroid may contribute to myocardial fibrosis and cardiovascular injury during diabetes mellitus.
Glucocorticoids
Glucocorticoids are produced in the adrenal cortex. They play a key role in the regulation of immunosuppressive and anti-inflammatory mechanisms in a cell type-specific manner, primarily through inhibition of cytokines.Citation60,Citation61 To uncover the role of the JAK-STAT pathway in these immunomodulatory actions of glucocorticoids, Bianchi et al. undertook a pioneering study in primary T cells.Citation62 They found that dexamethasone, a synthetic glucocorticoid, inhibited IL-2 signaling by suppression of IL-2 receptor and JAK3 kinase expression. The consequent reduction in tyrosine phosphorylation and nuclear translocation of STAT3 led to the decrease of IL-2 mediated gene activation and T-cell proliferation. In addition, due to its inhibitory effect on IL-2 receptor expression, dexamethasone had the ability to block signaling by cytokines like IL-4, IL-7, and IL-15, which use IL-2 receptor components. This in turn, indicated the potential of the steroid in suppressing T-cell response.
Haffner et al. reported an interesting crosstalk between the glucocorticoid receptor and SOCS1 (suppressor of cytokine signaling-1), a potent inhibitor of the JAK-STAT pathway.Citation63 They found that the glucocorticoid receptor and SOCS1 interact physically to form an intracellular complex and negatively influence the transcription of two glucocorticoid receptor-regulated genes. These results suggest a prominent role of SOCS1 in the early phase of cross-talk between glucocorticoid receptor and cytokine signaling. Furthermore, glucocorticoids increased the nuclear level of SOCS1. In addition to their immunosuppressive functions, glucocorticoids are potent inducers of apoptosis,Citation64,Citation65 and the development of resistance to such anti-proliferative signals in tumor cells challenges the successful implementation of current therapies. Krasil’nikov et al. uncovered the existence of an autoregulatory feedback loop consisting of JAK2, STAT3, and PI3K that resulted in enhanced resistance to dexamethasone treatment in melanoma cells.Citation66
The major signaling pathways activated by leptin, a hypothalamic hormone and central regulator of metabolic homeostasis, are the JAK-STAT pathway and the mitogen-activated protein kinase (MAPK) pathway.Citation67 Importantly, the JAK-STAT pathway is the crucial pathway in leptin-mediated energy homeostasis and metabolism.Citation67 Glucocorticoids or hypersensitivity to glucocorticoids which have a permissive effect on the development of obesity were found to antagonize leptin action in rodents.Citation68 Ishida-Takahashi et al. showed for the first time that glucocorticoids have the potential to inhibit leptin-induced tyrosine phosphorylation of JAK2 and activation of STAT3 in human hepatoma cell lines Huh7, partly via the MAPK cascade.Citation69
JAK and Retinoic Acid Receptor Interaction
Retinoic acid receptor α (RARα) is an important regulator of myeloid cell proliferation and differentiation.Citation70-Citation72 Si and Collins reported that IL-3 upregulates RARα transcriptional activity, which in turn was accompanied by commitment of multipotent immature extramedullary myeloid leukemia cells to a granulocyte/monocyte lineage.Citation73 Interestingly, the increased receptor activity was primarily driven by a JAK2-dependent pathway. Yet another cytokine, IL-6, triggered the JAK-STAT pathway to increase the expression of RARα in astrocytes.Citation74 Along with STAT, p300/CBP, and the Smad complex, RARα potentiated the transcription of glia specific marker GFAP (glial fibrillary acidic protein). Finally, the JAK-STAT pathway was found to be profoundly deregulated in vitamin A-deficient rat liver.Citation75 Nuclear phospho-JAK2 and phospho-SHP-1, a ubiquitously expressed tyrosine-specific protein phosphatase, were reduced whereas phosphorylated STAT5 tended to accumulate in the nucleus.
JAK and Vitamin D Interaction
Vitamin D has potential protective effects against prostate cancer and the most active vitamin D analog 1α,25 dihydroxyvitamin D3 (1,25(OH)2D3) has been reported to exert anti-cancer effects in cultured prostate cancer cells or animal tumor models.Citation76 In an attempt to decipher the underlying mechanism, Kovalenko et al. investigated the transcript profile of 1,25(OH)2D3-treated RWPE1 cells, an immortalized, non-tumorigenic human prostate epithelial cell line.Citation77 The vitamin was found to block cytokine production and suppressed the JAK-STAT pathway which mediates the proliferative effects of IL-6, IL-12, and INFγ. A similar inhibitory effect of 1,25(OH)2D3 on JAK signaling was observed in experimental allergic encephalomyelitis, an autoimmune disease of the central nervous system.Citation78 In contrast, in UMR106 osteoblast-like cells, 1,25(OH)2D3 repressed SOCS3 and cytokine-inducible SH2-containing protein (CIS), two negative regulators of JAK2-STAT5 signaling, and thereby potentiated GH action.Citation79
JAK Interaction with Peroxisome Proliferator-Activated Receptors
The peroxisome proliferator-activated receptors (PPARs) are a large family (PPARα, PPARβ/δ, and PPARγ)of lipid-binding nuclear receptors which play essential roles in the regulation of cellular differentiation, development, and metabolism (carbohydrate, lipid, and protein).Citation80,Citation81 PPARs heterodimerize with the retinoid X receptor and bind to PPAR response elements (PPREs) in the DNA to regulate gene expression.
Peroxisome proliferator-activated receptor α (PPARα) is essential for maintaining liver homeostasis,Citation80,Citation81 and it also participates in mediating the carcinogenic and tumor-promoting effects of a wide array of non-genotoxic agents.Citation82 Several groups independently reported an inhibitory effect of GH on induction of peroxisomal enzymes and peroxisome proliferation.Citation83-Citation86 The underlying mechanism remained unclear until Zhou et al. showed that GH-induced JAK2 and STAT5B is involved in the suppression of PPARα activity.Citation87 The PPARγ isoform is known to intervene in the production of cytokines and/or chemokines and subsequently, modulate the development of an inflammatory response. The natural PPARγ agonist 15-deoxy-Δ12,14-prostaglandin J2 (15d-PGJ2) was reported to inhibit the expression of a T-cell attracting chemokine, IP-10/CXCL10, by targeting the JAK-STAT pathway in mouse mesangial cells.Citation88
Concluding Remarks
This brief review gives an overview on the high complexity in the interplay between cytokine signaling, the JAK-STAT pathway and the expression level and function of steroid receptors in different tissues. Interaction of different JAK isoforms with a steroid receptor may have opposite effects in the same tissue and cross-talk between JAK and a certain steroid receptor may elicit different processes in different tissues. Therefore the balanced cross-talk is of high relevance for the regulation and maintenance of cell and tissue specific physiological functions and disturbances may result in pathophysiological processes and diseases including cancer. The novel observation that JAK2 is a negative regulator of ERα, a steroid receptor involved in breast cancer development and progression, suggests that more research is necessary regarding the use of JAK inhibitors in cancer therapy.
Abbreviations: | ||
ACE | = | angiotensin converting enzyme |
ACTH | = | adrenocorticotropic hormone |
AR | = | androgen receptor |
cAMP | = | cyclic adenosine monophosphate |
CREB | = | cAMP response element-binding protein |
GH | = | growth hormone |
IL | = | interleukin |
JAK | = | Janus kinase |
ERα | = | estrogen receptor-alpha |
LIF | = | leukemia inhibitory factor |
MAPK | = | mitogen-activated protein kinase |
siRNA | = | small interfering RNA |
MPA | = | medroxyprogesterone acetate |
PI3K | = | phosphatidylinositol 3-kinase |
PKA | = | protein kinase A |
PPAR | = | peroxisome proliferator-activated receptor |
PRL | = | prolactin |
RAR | = | retinoic acid receptor |
SOCS | = | suppressor of cytokine signaling |
StAR | = | steroidogenic acute regulatory protein |
STAT | = | signal transducer and activator of transcription |
TYK2 | = | tyrosine kinase 2 |
VLDL | = | very low density lipoproteins |
Disclosure of Potential Conflicts of Interest
No potential conflicts of interest were disclosed.
References
- Leonard WJ, O’Shea JJ. Jaks and STATs: biological implications. Annu Rev Immunol 1998; 16:293 - 322; http://dx.doi.org/10.1146/annurev.immunol.16.1.293; PMID: 9597132
- Yamaoka K, Saharinen P, Pesu M, Holt VE 3rd, Silvennoinen O, O’Shea JJ. The Janus kinases (Jaks). Genome Biol 2004; 5:253; http://dx.doi.org/10.1186/gb-2004-5-12-253; PMID: 15575979
- Rane SG, Reddy EP. Janus kinases: components of multiple signaling pathways. Oncogene 2000; 19:5662 - 79; http://dx.doi.org/10.1038/sj.onc.1203925; PMID: 11114747
- Rawlings JS, Rosler KM, Harrison DA. The JAK/STAT signaling pathway. J Cell Sci 2004; 117:1281 - 3; http://dx.doi.org/10.1242/jcs.00963; PMID: 15020666
- Boudny V, Kovarik J. JAK/STAT signaling pathways and cancer. Janus kinases/signal transducers and activators of transcription. Neoplasma 2002; 49:349 - 55; PMID: 12584581
- Verma A, Kambhampati S, Parmar S, Platanias LC. Jak family of kinases in cancer. Cancer Metastasis Rev 2003; 22:423 - 34; http://dx.doi.org/10.1023/A:1023805715476; PMID: 12884916
- Wagner KU, Rui H. Jak2/Stat5 signaling in mammogenesis, breast cancer initiation and progression. J Mammary Gland Biol Neoplasia 2008; 13:93 - 103; http://dx.doi.org/10.1007/s10911-008-9062-z; PMID: 18228120
- LaFave LM, Levine RL. JAK2 the future: therapeutic strategies for JAK-dependent malignancies. Trends Pharmacol Sci 2012; 33:574 - 82; http://dx.doi.org/10.1016/j.tips.2012.08.005; PMID: 22995223
- Stein BL, Crispino JD, Moliterno AR. Janus kinase inhibitors: an update on the progress and promise of targeted therapy in the myeloproliferative neoplasms. Curr Opin Oncol 2011; 23:609 - 16; PMID: 21993415
- Menet CJ, Rompaey LV, Geney R. Advances in the discovery of selective JAK inhibitors. Prog Med Chem 2013; 52:153 - 223; PMID: 23384668
- Wang L, Zhang X, Farrar WL, Yang X. Transcriptional crosstalk between nuclear receptors and cytokine signal transduction pathways in immunity. Cell Mol Immunol 2004; 1:416 - 24; PMID: 16293210
- Mangelsdorf DJ, Thummel C, Beato M, Herrlich P, Schütz G, Umesono K, et al. The nuclear receptor superfamily: the second decade. Cell 1995; 83:835 - 9; http://dx.doi.org/10.1016/0092-8674(95)90199-X; PMID: 8521507
- Zhang Z, Burch PE, Cooney AJ, Lanz RB, Pereira FA, Wu J, et al. Genomic analysis of the nuclear receptor family: new insights into structure, regulation, and evolution from the rat genome. Genome Res 2004; 14:580 - 90; http://dx.doi.org/10.1101/gr.2160004; PMID: 15059999
- Cleary MP, Grossmann ME. Minireview: Obesity and breast cancer: the estrogen connection. Endocrinology 2009; 150:2537 - 42; http://dx.doi.org/10.1210/en.2009-0070; PMID: 19372199
- Nuclear Receptors Nomenclature Committee. A unified nomenclature system for the nuclear receptor superfamily. Cell 1999; 97:161 - 3; http://dx.doi.org/10.1016/S0092-8674(00)80726-6; PMID: 10219237
- Novac N, Heinzel T. Nuclear receptors: overview and classification. Curr Drug Targets Inflamm Allergy 2004; 3:335 - 46; http://dx.doi.org/10.2174/1568010042634541; PMID: 15584884
- Levin ER. Minireview: Extranuclear steroid receptors: roles in modulation of cell functions. Mol Endocrinol 2011; 25:377 - 84; http://dx.doi.org/10.1210/me.2010-0284; PMID: 20861220
- McKenna NJ, O’Malley BW. Combinatorial control of gene expression by nuclear receptors and coregulators. Cell 2002; 108:465 - 74; http://dx.doi.org/10.1016/S0092-8674(02)00641-4; PMID: 11909518
- Mangelsdorf DJ, Evans RM. The RXR heterodimers and orphan receptors. Cell 1995; 83:841 - 50; http://dx.doi.org/10.1016/0092-8674(95)90200-7; PMID: 8521508
- Kininis M, Kraus WL. A global view of transcriptional regulation by nuclear receptors: gene expression, factor localization, and DNA sequence analysis. Nucl Recept Signal 2008; 6:e005; PMID: 18301785
- Geserick C, Meyer HA, Haendler B. The role of DNA response elements as allosteric modulators of steroid receptor function. Mol Cell Endocrinol 2005; 236:1 - 7; http://dx.doi.org/10.1016/j.mce.2005.03.007; PMID: 15876478
- Maggiolini M, Picard D. The unfolding stories of GPR30, a new membrane-bound estrogen receptor. J Endocrinol 2010; 204:105 - 14; http://dx.doi.org/10.1677/JOE-09-0242; PMID: 19767412
- Beatson GT. On the treatment of inoperable cases of carcinoma of the mamma: suggestions for a new method of treatment with illustrative cases. Lancet 1896; 2:104 - 7; http://dx.doi.org/10.1016/S0140-6736(01)72307-0
- Platet N, Cathiard AM, Gleizes M, Garcia M. Estrogens and their receptors in breast cancer progression: a dual role in cancer proliferation and invasion. Crit Rev Oncol Hematol 2004; 51:55 - 67; http://dx.doi.org/10.1016/j.critrevonc.2004.02.001; PMID: 15207254
- Weigel NL, Zhang Y. Ligand-independent activation of steroid hormone receptors. J Mol Med (Berl) 1998; 76:469 - 79; http://dx.doi.org/10.1007/s001090050241; PMID: 9660165
- Coleman KM, Smith CL. Intracellular signaling pathways: nongenomic actions of estrogens and ligand-independent activation of estrogen receptors. Front Biosci 2001; 6:D1379 - 91; http://dx.doi.org/10.2741/Coleman; PMID: 11578956
- Weigel NL, Moore NL. Steroid receptor phosphorylation: a key modulator of multiple receptor functions. Mol Endocrinol 2007; 21:2311 - 9; http://dx.doi.org/10.1210/me.2007-0101; PMID: 17536004
- Song RX, Chen Y, Zhang Z, Bao Y, Yue W, Wang JP, et al. Estrogen utilization of IGF-1-R and EGF-R to signal in breast cancer cells. J Steroid Biochem Mol Biol 2010; 118:219 - 30; http://dx.doi.org/10.1016/j.jsbmb.2009.09.018; PMID: 19815064
- Gupta N, Grebhardt S, Mayer D. Janus kinase 2--a novel negative regulator of estrogen receptor α function. Cell Signal 2012; 24:151 - 61; http://dx.doi.org/10.1016/j.cellsig.2011.08.016; PMID: 21907792
- de Souza Rocha Simonini P, Breiling A, Gupta N, Malekpour M, Youns M, Omranipour R, et al. Epigenetically deregulated microRNA-375 is involved in a positive feedback loop with estrogen receptor alpha in breast cancer cells. Cancer Res 2010; 70:9175 - 84; http://dx.doi.org/10.1158/0008-5472.CAN-10-1318; PMID: 20978187
- Yeh YT, Ou-Yang F, Chen IF, Yang SF, Su JH, Hou MF, et al. Altered p-JAK1 expression is associated with estrogen receptor status in breast infiltrating ductal carcinoma. Oncol Rep 2007; 17:35 - 9; PMID: 17143475
- Garofalo C, Surmacz E. Leptin and cancer. J Cell Physiol 2006; 207:12 - 22; http://dx.doi.org/10.1002/jcp.20472; PMID: 16110483
- Cirillo D, Rachiglio AM, la Montagna R, Giordano A, Normanno N. Leptin signaling in breast cancer: an overview. J Cell Biochem 2008; 105:956 - 64; http://dx.doi.org/10.1002/jcb.21911; PMID: 18821585
- Andò S, Catalano S. The multifactorial role of leptin in driving the breast cancer microenvironment. Nat Rev Endocrinol 2012; 8:263 - 75; http://dx.doi.org/10.1038/nrendo.2011.184; PMID: 22083089
- Davidson MB. Effect of growth hormone on carbohydrate and lipid metabolism. Endocr Rev 1987; 8:115 - 31; http://dx.doi.org/10.1210/edrv-8-2-115; PMID: 3301316
- Goffin V, Kelly PA. The prolactin/growth hormone receptor family: structure/function relationships. J Mammary Gland Biol Neoplasia 1997; 2:7 - 17; http://dx.doi.org/10.1023/A:1026313211704; PMID: 10887515
- Leung KC, Doyle N, Ballesteros M, Sjogren K, Watts CK, Low TH, et al. Estrogen inhibits GH signaling by suppressing GH-induced JAK2 phosphorylation, an effect mediated by SOCS2. Proc Natl Acad Sci U S A 2003; 100:1016 - 21; http://dx.doi.org/10.1073/pnas.0337600100; PMID: 12552091
- Leung KC, Brce J, Doyle N, Lee HJ, Leong GM, Sjögren K, et al. Regulation of growth hormone signaling by selective estrogen receptor modulators occurs through suppression of protein tyrosine phosphatases. Endocrinology 2007; 148:2417 - 23; http://dx.doi.org/10.1210/en.2006-1305; PMID: 17272397
- Bachelot A, Binart N. Reproductive role of prolactin. Reproduction 2007; 133:361 - 9; http://dx.doi.org/10.1530/REP-06-0299; PMID: 17307904
- Frasor J, Gibori G. Prolactin regulation of estrogen receptor expression. Trends Endocrinol Metab 2003; 14:118 - 23; http://dx.doi.org/10.1016/S1043-2760(03)00030-4; PMID: 12670737
- Frasor J, Barkai U, Zhong L, Fazleabas AT, Gibori G. PRL-induced ERalpha gene expression is mediated by Janus kinase 2 (Jak2) while signal transducer and activator of transcription 5b (Stat5b) phosphorylation involves Jak2 and a second tyrosine kinase. Mol Endocrinol 2001; 15:1941 - 52; http://dx.doi.org/10.1210/me.15.11.1941; PMID: 11682625
- Zhao Y, Nichols JE, Bulun SE, Mendelson CR, Simpson ER. Aromatase P450 gene expression in human adipose tissue. Role of a Jak/STAT pathway in regulation of the adipose-specific promoter. J Biol Chem 1995; 270:16449 - 57; http://dx.doi.org/10.1074/jbc.270.27.16449; PMID: 7608217
- Lange CA. Integration of progesterone receptor action with rapid signaling events in breast cancer models. J Steroid Biochem Mol Biol 2008; 108:203 - 12; http://dx.doi.org/10.1016/j.jsbmb.2007.09.019; PMID: 17964138
- Lange CA, Richer JK, Horwitz KB. Hypothesis: Progesterone primes breast cancer cells for cross-talk with proliferative or antiproliferative signals. Mol Endocrinol 1999; 13:829 - 36; http://dx.doi.org/10.1210/me.13.6.829; PMID: 10379882
- Proietti C, Salatino M, Rosemblit C, Carnevale R, Pecci A, Kornblihtt AR, et al. Progestins induce transcriptional activation of signal transducer and activator of transcription 3 (Stat3) via a Jak- and Src-dependent mechanism in breast cancer cells. Mol Cell Biol 2005; 25:4826 - 40; http://dx.doi.org/10.1128/MCB.25.12.4826-4840.2005; PMID: 15923602
- Sagare-Patil V, Modi D. Progesterone activates Janus Kinase 1/2 and activators of transcription 1 (JAK1-2/STAT1) pathway in human spermatozoa. Andrologia 2013; 45:178 - 86; http://dx.doi.org/10.1111/j.1439-0272.2012.01332.x; PMID: 22748021
- Laflamme J, Akoum A, Leclerc P. Induction of human sperm capacitation and protein tyrosine phosphorylation by endometrial cells and interleukin-6. Mol Hum Reprod 2005; 11:141 - 50; http://dx.doi.org/10.1093/molehr/gah142; PMID: 15665187
- Lachance C, Leclerc P. Mediators of the Jak/STAT signaling pathway in human spermatozoa. Biol Reprod 2011; 85:1222 - 31; http://dx.doi.org/10.1095/biolreprod.111.092379; PMID: 21880948
- Gelmann EP. Molecular biology of the androgen receptor. J Clin Oncol 2002; 20:3001 - 15; http://dx.doi.org/10.1200/JCO.2002.10.018; PMID: 12089231
- Ueda T, Bruchovsky N, Sadar MD. Activation of the androgen receptor N-terminal domain by interleukin-6 via MAPK and STAT3 signal transduction pathways. J Biol Chem 2002; 277:7076 - 85; http://dx.doi.org/10.1074/jbc.M108255200; PMID: 11751884
- Kanda T, Steele R, Ray R, Ray RB. Hepatitis C virus core protein augments androgen receptor-mediated signaling. J Virol 2008; 82:11066 - 72; http://dx.doi.org/10.1128/JVI.01300-08; PMID: 18768969
- Okitsu K, Kanda T, Imazeki F, Yonemitsu Y, Ray RB, Chang C, et al. Involvement of interleukin-6 and androgen receptor signaling in pancreatic cancer. Genes Cancer 2010; 1:859 - 67; http://dx.doi.org/10.1177/1947601910383417; PMID: 21779469
- Martinez HD, Hsiao JJ, Jasavala RJ, Hinkson IV, Eng JK, Wright ME. Androgen-sensitive microsomal signaling networks coupled to the proliferation and differentiation of human prostate cancer cells. Genes Cancer 2011; 2:956 - 78; http://dx.doi.org/10.1177/1947601912436422; PMID: 22701762
- Lefrancois-Martinez AM, Blondet-Trichard A, Binart N, Val P, Chambon C, Sahut-Barnola I, et al. Transcriptional control of adrenal steroidogenesis: novel connection between Janus kinase (JAK) 2 protein and protein kinase A (PKA) through stabilization of cAMP response element-binding protein (CREB) transcription factor. J Biol Chem 2011; 286:32976 - 85; http://dx.doi.org/10.1074/jbc.M111.218016; PMID: 21808064
- Johnston CI. Franz Volhard Lecture. Renin-angiotensin system: a dual tissue and hormonal system for cardiovascular control. J Hypertens Suppl 1992; 10:S13 - 26; PMID: 1337911
- Marrero MB, Venema VJ, Ju H, Eaton DC, Venema RC. Regulation of angiotensin II-induced JAK2 tyrosine phosphorylation: roles of SHP-1 and SHP-2. Am J Physiol 1998; 275:C1216 - 23; PMID: 9814969
- Li J, Feltzer RE, Dawson KL, Hudson EA, Clark BJ. Janus kinase 2 and calcium are required for angiotensin II-dependent activation of steroidogenic acute regulatory protein transcription in H295R human adrenocortical cells. J Biol Chem 2003; 278:52355 - 62; http://dx.doi.org/10.1074/jbc.M305232200; PMID: 14565954
- Sugiyama T, Yoshimoto T, Tsuchiya K, Gochou N, Hirono Y, Tateno T, et al. Aldosterone induces angiotensin converting enzyme gene expression via a JAK2-dependent pathway in rat endothelial cells. Endocrinology 2005; 146:3900 - 6; http://dx.doi.org/10.1210/en.2004-1674; PMID: 15932931
- Saha S, Bornstein SR, Graessler J, Kopprasch S. Very-low-density lipoprotein mediates transcriptional regulation of aldosterone synthase in human adrenocortical cells through multiple signaling pathways. Cell Tissue Res 2012; 348:71 - 80; http://dx.doi.org/10.1007/s00441-012-1346-3; PMID: 22331364
- Almawi WY, Beyhum HN, Rahme AA, Rieder MJ. Regulation of cytokine and cytokine receptor expression by glucocorticoids. J Leukoc Biol 1996; 60:563 - 72; PMID: 8929546
- Necela BM, Cidlowski JA. Mechanisms of glucocorticoid receptor action in noninflammatory and inflammatory cells. Proc Am Thorac Soc 2004; 1:239 - 46; http://dx.doi.org/10.1513/pats.200402-005MS; PMID: 16113441
- Bianchi M, Meng C, Ivashkiv LB. Inhibition of IL-2-induced Jak-STAT signaling by glucocorticoids. Proc Natl Acad Sci U S A 2000; 97:9573 - 8; http://dx.doi.org/10.1073/pnas.160099797; PMID: 10920190
- Haffner MC, Jurgeit A, Berlato C, Geley S, Parajuli N, Yoshimura A, et al. Interaction and functional interference of glucocorticoid receptor and SOCS1. J Biol Chem 2008; 283:22089 - 96; http://dx.doi.org/10.1074/jbc.M801041200; PMID: 18524780
- Greenstein S, Ghias K, Krett NL, Rosen ST. Mechanisms of glucocorticoid-mediated apoptosis in hematological malignancies. Clin Cancer Res 2002; 8:1681 - 94; PMID: 12060604
- Almawi WY, Melemedjian OK. Molecular mechanisms of glucocorticoid antiproliferative effects: antagonism of transcription factor activity by glucocorticoid receptor. J Leukoc Biol 2002; 71:9 - 15; PMID: 11781376
- Krasil’nikov M, Shatskaya V. Signal transducer and activator of transcription-3 and phosphatidylinositol-3 kinase as coordinate regulators of melanoma cell response to glucocorticoid hormones. J Steroid Biochem Mol Biol 2002; 82:369 - 76; http://dx.doi.org/10.1016/S0960-0760(02)00223-6; PMID: 12589944
- Yang R, Barouch LA. Leptin signaling and obesity: cardiovascular consequences. Circ Res 2007; 101:545 - 59; http://dx.doi.org/10.1161/CIRCRESAHA.107.156596; PMID: 17872473
- Zakrzewska KE, Cusin I, Sainsbury A, Rohner-Jeanrenaud F, Jeanrenaud B. Glucocorticoids as counterregulatory hormones of leptin: toward an understanding of leptin resistance. Diabetes 1997; 46:717 - 9; http://dx.doi.org/10.2337/diabetes.46.4.717; PMID: 9075817
- Ishida-Takahashi R, Uotani S, Abe T, Degawa-Yamauchi M, Fukushima T, Fujita N, et al. Rapid inhibition of leptin signaling by glucocorticoids in vitro and in vivo. J Biol Chem 2004; 279:19658 - 64; http://dx.doi.org/10.1074/jbc.M310864200; PMID: 14993217
- Douer D, Ramezani L, Parker J, Levine AM. All-trans-retinoic acid effects the growth, differentiation and apoptosis of normal human myeloid progenitors derived from purified CD34+ bone marrow cells. Leukemia 2000; 14:874 - 81; http://dx.doi.org/10.1038/sj.leu.2401772; PMID: 10803520
- Labrecque J, Allan D, Chambon P, Iscove NN, Lohnes D, Hoang T. Impaired granulocytic differentiation in vitro in hematopoietic cells lacking retinoic acid receptors alpha1 and gamma. Blood 1998; 92:607 - 15; PMID: 9657762
- Collins SJ. The role of retinoids and retinoic acid receptors in normal hematopoiesis. Leukemia 2002; 16:1896 - 905; http://dx.doi.org/10.1038/sj.leu.2402718; PMID: 12357341
- Si J, Collins SJ. IL-3-induced enhancement of retinoic acid receptor activity is mediated through Stat5, which physically associates with retinoic acid receptors in an IL-3-dependent manner. Blood 2002; 100:4401 - 9; http://dx.doi.org/10.1182/blood-2001-12-0374; PMID: 12393611
- Herrera F, Chen Q, Schubert D. Synergistic effect of retinoic acid and cytokines on the regulation of glial fibrillary acidic protein expression. J Biol Chem 2010; 285:38915 - 22; http://dx.doi.org/10.1074/jbc.M110.170274; PMID: 20876578
- Murray M, Butler AM, Fiala-Beer E, Su GM. Phospho-STAT5 accumulation in nuclear fractions from vitamin A-deficient rat liver. FEBS Lett 2005; 579:3669 - 73; http://dx.doi.org/10.1016/j.febslet.2005.05.052; PMID: 15963505
- Fleet JC. Molecular actions of vitamin D contributing to cancer prevention. Mol Aspects Med 2008; 29:388 - 96; http://dx.doi.org/10.1016/j.mam.2008.07.003; PMID: 18755215
- Kovalenko PL, Zhang Z, Cui M, Clinton SK, Fleet JC. 1,25 dihydroxyvitamin D-mediated orchestration of anticancer, transcript-level effects in the immortalized, non-transformed prostate epithelial cell line, RWPE1. BMC Genomics 2010; 11:26; http://dx.doi.org/10.1186/1471-2164-11-26; PMID: 20070897
- Muthian G, Raikwar HP, Rajasingh J, Bright JJ. 1,25 Dihydroxyvitamin-D3 modulates JAK-STAT pathway in IL-12/IFNgamma axis leading to Th1 response in experimental allergic encephalomyelitis. J Neurosci Res 2006; 83:1299 - 309; http://dx.doi.org/10.1002/jnr.20826; PMID: 16547967
- Morales O, Faulds MH, Lindgren UJ, Haldosén LA. 1Alpha,25-dihydroxyvitamin D3 inhibits GH-induced expression of SOCS3 and CIS and prolongs growth hormone signaling via the Janus kinase (JAK2)/signal transducers and activators of transcription (STAT5) system in osteoblast-like cells. J Biol Chem 2002; 277:34879 - 84; http://dx.doi.org/10.1074/jbc.M204819200; PMID: 12107179
- Wahli W, Braissant O, Desvergne B. Peroxisome proliferator activated receptors: transcriptional regulators of adipogenesis, lipid metabolism and more... Chem Biol 1995; 2:261 - 6; http://dx.doi.org/10.1016/1074-5521(95)90045-4; PMID: 9383428
- Feige JN, Gelman L, Michalik L, Desvergne B, Wahli W. From molecular action to physiological outputs: peroxisome proliferator-activated receptors are nuclear receptors at the crossroads of key cellular functions. Prog Lipid Res 2006; 45:120 - 59; http://dx.doi.org/10.1016/j.plipres.2005.12.002; PMID: 16476485
- Peters JM, Cattley RC, Gonzalez FJ. Role of PPAR alpha in the mechanism of action of the nongenotoxic carcinogen and peroxisome proliferator Wy-14,643. Carcinogenesis 1997; 18:2029 - 33; http://dx.doi.org/10.1093/carcin/18.11.2029; PMID: 9395198
- Lee SS, Pineau T, Drago J, Lee EJ, Owens JW, Kroetz DL, et al. Targeted disruption of the alpha isoform of the peroxisome proliferator-activated receptor gene in mice results in abolishment of the pleiotropic effects of peroxisome proliferators. Mol Cell Biol 1995; 15:3012 - 22; PMID: 7539101
- Sugiyama H, Yamada J, Suga T. Effects of testosterone, hypophysectomy and growth hormone treatment on clofibrate induction of peroxisomal beta-oxidation in female rat liver. Biochem Pharmacol 1994; 47:918 - 21; http://dx.doi.org/10.1016/0006-2952(94)90494-4; PMID: 8135869
- Sundseth SS, Waxman DJ. Sex-dependent expression and clofibrate inducibility of cytochrome P450 4A fatty acid omega-hydroxylases. Male specificity of liver and kidney CYP4A2 mRNA and tissue-specific regulation by growth hormone and testosterone. J Biol Chem 1992; 267:3915 - 21; PMID: 1740439
- Yamada J, Sugiyama H, Tamura H, Suga T. Hormonal modulation of peroxisomal enzyme induction caused by peroxisome proliferators: suppression by growth and thyroid hormones in cultured rat hepatocytes. Arch Biochem Biophys 1994; 315:555 - 7; http://dx.doi.org/10.1006/abbi.1994.1536; PMID: 7986103
- Zhou YC, Waxman DJ. Cross-talk between janus kinase-signal transducer and activator of transcription (JAK-STAT) and peroxisome proliferator-activated receptor-alpha (PPARalpha) signaling pathways. Growth hormone inhibition of pparalpha transcriptional activity mediated by stat5b. J Biol Chem 1999; 274:2672 - 81; http://dx.doi.org/10.1074/jbc.274.5.2672; PMID: 9915797
- Panzer U, Zahner G, Wienberg U, Steinmetz OM, Peters A, Turner JE, et al. 15-deoxy-Delta12,14-prostaglandin J2 inhibits INF-gamma-induced JAK/STAT1 signalling pathway activation and IP-10/CXCL10 expression in mesangial cells. Nephrol Dial Transplant 2008; 23:3776 - 85; http://dx.doi.org/10.1093/ndt/gfn361; PMID: 18596134