Abstract
Organophosphates are potent poisoning agents that cause severe cholinergic toxicity. Current treatment has been reported to be unsatisfactory and novel antidotes are needed. In this study, we used a single-chain variable fragment (scFv) library to select a recombinant antibody fragment (WZ1–14.2.1) with butyrylcholinesterase-like catalytic activity by using an innovative method integrating genetic selection and the bait-and-switch strategy. Ellman assay demonstrated that WZ1–14.2.1 has Michaelis-Menten kinetics in the hydrolysis of all the three substrates used, acetylthiocholine, propionylthiocholine and butyrylthiocholine. Notably, the catalytic activity was resistant to the following acetylcholinesterase inhibitors: neostigmine, iso-OMPA, chlorpyrifos oxon, dichlorvos, and paraoxon ethyl. Otherwise, the enzymatic activity of WZ1–14.2.1 was inhibited by the selective butyrylcholinesterase inhibitor, ethopropazine, and by the Ser-blocking agent phenylmethanesuphonyl fluoride. A hypothetical 3D structure of the WZ1–14.2.1 catalytic site, compatible with functional results, is proposed on the basis of a molecular modeling analysis.
Introduction
Every year, all around the world, hundreds of thousands deaths are caused by the irreversible inhibition of acetylcholinesterase (AChE; EC 3.1.1.7) due to accidental exposure, suicide, or homicide. In addition, the enzyme is the target of nerve agents, chemical weapons used for mass injury, as was recently observed in the sarin attack on Syrian civilians. These chemicals are ester or amide derivatives of phosphonic acid, structurally related to organophosphate insecticides such as malathion. The organophosphates block the enzyme by covalently binding the Ser oxygen located at the active site;Citation1 inhibition is initially reversible, until the inhibited enzyme undergoes spontaneous dealkylation of the Ser-bound organophosphate (aging), resulting in an irreversibly phosphorylated enzyme.Citation2 The inability of inhibited AchE to hydrolyse acetylcholine (ACh) causes transmitter accumulation in synapses of both the peripheral and central nervous systems and in neuromuscular junctions. This results in generalized over-stimulation of cholinergic receptors, followed by breakdown of ganglionic and neuromuscular transmission. While atropine and other anticholinergic drugs competitively counteract muscarinic symptoms, they do not restore the impaired neuromuscular transmission and are ineffective in preventing respiratory muscle failure. On the other hand, oximes, such as HI-6 (2-hydroxyiminomethyl-4’-carbamoyl-pyridinium-1’-methylether dichloride monohydrate), pralidoxime, obidoxime and others, can reverse the AChE-organophosphate complex by reacting as nucleophiles against the phosphate group and restore the AChE activity. However, these agents are rather ineffective against some nerve agents, including soman and sarinCitation3 and fully ineffective against the aged phosphorylated enzyme.
The search for novel chemical antidotes is hampered by substantial differences in poison-antidote kinetics and activity that are observed among animal speciesCitation4 and by the occurrence of spontaneous dephosphylation of the phosphyl-AChE-complex. This led to efforts toward novel therapeutic strategies, such as poison scavengers that act in the internal systemic or external artificial blood circulation.
To date, two basic strategies have been proposed. The first one is based on the administration of natural or engineered enzymes (catalytic bioscavengers) of the organophosphate breakdown pathway such as paraoxonase and other esterases.Citation5-Citation9 Special micro and nano-delivery systems have also been proposed to stabilize the enzyme persistence in serum and to minimize its potential for inducing a host antibody response.Citation10,Citation11 The second one is based on natural or engineered forms of AChE/butyrylcholinesterase (BChE) as suicide enzymes.Citation12-Citation14 A transgenic-derived recombinant BChE in a pegylated form (ProtexiaTM) is the only such agent entered in a Phase 1 clinical study,Citation15 which ended in 2009 (NCT00744146).
Although both catalytic and neutralizing bioscavengers have been proven to be effective, they also have inherent limitations. Enhanced poison removal (through catalysis or linkage) does not accelerate recovery of already inhibited endogenous AChE. This is the reason why bioscavengers are mainly intended for prophylaxis against a chemical attack.Citation5 Although a recent publication suggests a possible utility of BchE also in the post-exposure period,Citation16 the authors themselves pointed out that the BchE administration was performed at a time when the signs of systemic poisoning induced by the organophosphate were not yet evident, consistently with a bioscavenger efficacy proved in the early stage of intoxication. In addition, the use of suicide enzymes is poorly effective, unless stoichiometry with the amount of toxic that entered the body is obtained; this amount may be in the order of grams. Moreover, with regards to organophosphate toxicokinetics, it should be noted that part of the absorbed dose undergoes redistribution after storing in fat tissue, making it very difficult to predict blood concentrations.
These notions prompted us to develop a new potential therapeutic agent against intoxication produced by AChE inhibitors. In particular, here we report the successful isolation of a human single-chain variable fragment (scFv) with BChE-like activity, resistant to organophosphates and carbamates.
The abzyme fragment has been selected with the aim to replace the endogenous poisoned AChE with a small isozyme having a low immunogenic potential, able to diffuse in synapses and neuromuscular junctions due to its molecular weight and resistant to poisons due to the geometry of its active site.
Results
Clone selection and scFv identification
HB2151 E.coli cells transformed with Tomlinson I+J phage library were grown in the agar selective medium, containing succinylcholine chloride () whose metabolite succinic acid represented the sole source of reduced carbon in the medium. After 10 d incubation, only a few colonies (<50/ about 1.4 × 108 seeded) of HB2151 cells transformed with the Tomlinson I + J phage library grew under the selective culture conditions used in our experimental setting (), while no untransformed HB2151 cells survived in the selective medium. The most quickly growing colonies from the first round of selection were submitted to a second round of selection in the same culture medium to minimize the possibility of false positive clonal selection, coming from parasitic cells devoid of the desired genotype, and to rule out gene instability as a cause of cell loss.
Figure 1. (A) Molecular formula of the agent (succinylcholine) used as substrate to select the HB2151 E. coli cells able to grow in the selective minimum medium; (B) petri dish containing colonies of HB2151 cells from the first round of selection able to growth in the selective minimum medium.
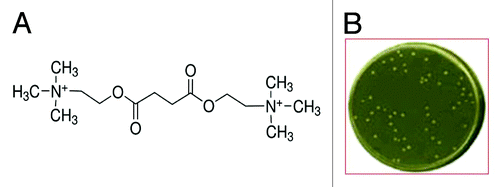
Five isolated colonies, among those already detectable after 2–3 d incubation in aerobiosis condition at 37 °C were subjected to phagemid sequencing, revealing a >90% homology among the scFv inserts. Purification procedure of scFv gave a unique band of about 24 kDa weight in the SDS-PAGE analysis (). shows the primary structure of the catalytic scFv named WZ1–14.2.1, confirmed by MALDI-TOF analysis and deposited in the GenBank database under the accession number KF914159. The protein is an unconjugated polypeptide of 249 residues, with 26.268 kDa molecular weight, a theoretical pI of 9.02 and an average extinction coefficient (calculated from that obtained assuming all pairs of Cys residues forming cysteines and that assuming all Cys residues reduced) of 40.005 M−1 cm−1, at 280 nm. WZ1–14.2.1 is a recombinant chimera with the myc antigenic determinant and the 6xHis domain for Ni-resin purification.
Catalytic activity of the scFv WZ1–14.2.1
WZ1–14.2.1 catalysis showed a Michaelis-Menten kinetics for all the three substrates evaluated in the Ellman assay ().Their kinetic parameters are reported in .
Figure 3. General formula of the thio-substrates, used in the modified Ellman assay (37 °C, pH 7.4) and kinetic curves for the hydrolysis respectively of the three substrates, acetylthiocholine, propionylthiocholine and butyrylthiocholine, by WZ-14.2.1 (10−7 M) in the modified Ellman assay.
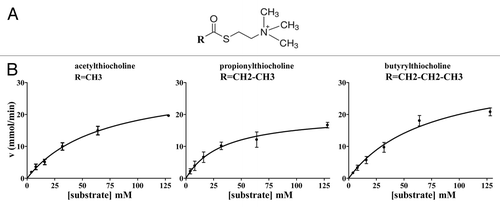
Table 1. Kinetic parameters obtained for the three substrates hydrolysis by WZ-14.2.1 (10−7 M) in the modified Ellman assay (37 °C; pH 7.4)
Of note, the carbamate AChE inhibitor neostigmine and the organophosphates paraoxon ethyl, iso-OMPA, chlorpyrifos oxon and dichlorvos (), tested in the range from 10−6 mM to 2 mM, did not significantly (P > 0.05) inhibit the enzymatic hydrolysis of acetylthiocholine by WZ1–14.2.1. In the same experimental conditions, both paraoxon ethyl and neostigmine showed an inhibitory action on the commercial Electrophorus Electricus AChE with IC50 values in the nanomolar range (60.5 ± 13.3 nM for paraoxon ethyl and 20.8 ± 5.4 nM for neostigmine), according to the literature.Citation17,Citation18
On the other hand, the selective BChE inhibitor ethopropazine, at the highest concentrations tested (1 and 2 mM) was able to significantly (P < 0.01) decrease the WZ1–14.2.1 enzymatic activity (). Moreover, also the Ser blocking agent PMSF, evaluated at 1 and 5 mM, inhibited the ability of WZ1–14.2.1 to hydrolyse acetylthiocholine (), suggesting a role of Ser in the catalytic mechanism of the scFv.
Figure 5. Concentration-dependent inhibition by the BChE inhibitor ethopropazine on the hydrolysis by WZ-14.2.1 (10−7 M) of the substrate acetylthiocholine verified through the Ellman modified assay (37 °C, pH 7.4). Data are means ± SEM from three separate experiments. *P < 0.05; **P < 0.01
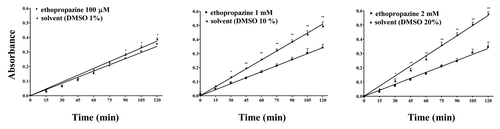
Figure 6. Concentration-dependent inhibition by the Ser blocking agent phenylmethanesuphonyl fluoride (PMSF) on the hydrolysis by WZ-14.2.1 (10−7 M) of the substrate acetylthiocholine verified through the Ellman modified assay (37 °C, pH 7.4). Data are means ± SEM from three separate experiments. *P < 0.05; **P < 0.01
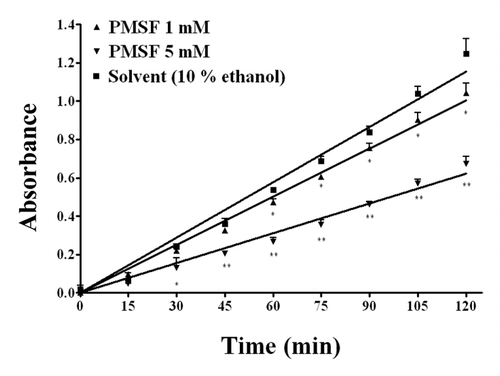
Finally, the fluorimetric ACh/AChE assay clearly demonstrated that WZ1–14.2.1 has also esterase activity against the physiologic mediator Ach ().
Figure 7. Kinetic profile obtained in the presence and in the absence (control) of WZ-14.2.1 (10−7 M) using the Amplex Red ® fluorimetric acetylcholinesterase/acetylcholine assay; *P < 0.05; **P < 0.01
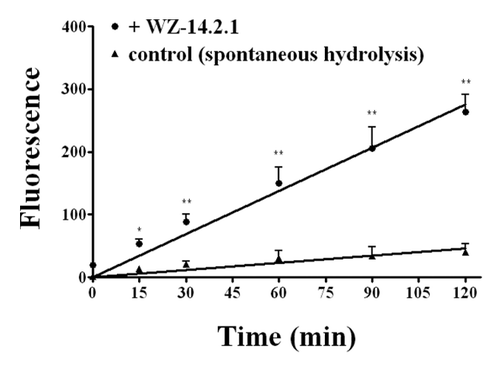
Molecular modeling analysis
The 3D structure of WZ-14.2.1 was built by homology modeling, exploiting several steps also used by other authors.Citation19
Multiple sequence alignment and secondary structure prediction
The amino acid sequences of WZ-14.2.1 and its closest homologs, with the PDBCitation20 codes 2GHW, 4CAU, 1F3R, 2GKI, and 1DZB, were aligned with the CLUSTALWCitation21 algorithm. On the obtained alignment (), we highlighted the complementarity-determining regions (CDRs) zone as predicted by the Rosetta antibody server.Citation22 It can be seen that all the light chain (L1, L2, and L3) and the H1 and H2 heavy chain CDRs are of equal length (except for 2GKI). The CDRs of H3 heavy chains instead show some differences, mainly for the 1F3R structure. Moreover in 4CAU and 2GKI structures, the H3 domain is unrecognized. H3 of WZ-14.2.1 is similar to that showed by 2GHW.
Figure 8. (A) CLUSTALW alignment between WZ-14.2.1 (query) and its closest homologous (identified by BLAST) with the PDB codes 2GHW, 4CAU, 1F3R, 2GKI and 1DZB; (B) 3D model of WZ-14.2.1 obtained by homology building on 2GHW template. The CDR zones (L1, L2, L3, H1, H2, and H3) are colored (in yellow, pink, green, red, dark green, and gray, respectively), the putative binding site is circled; (C) The best conformation of acetylthiocholine in the catalytic antibody obtained with the molecular docking analysis.
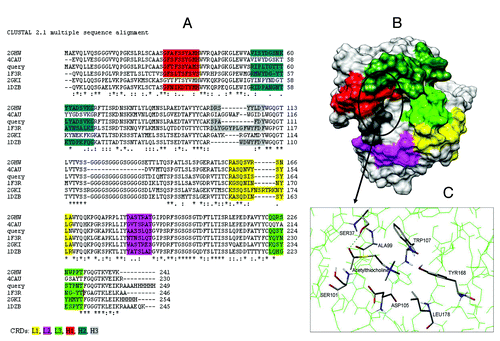
Given their amino acid sequences, secondary structures of WZ-14.2.1 and its homologs were also predicted and analyzed by the Jpred 3 program.Citation23 The secondary structure of WZ-14.2.1 showed a close similarity to the whole structures of the prototype scFv antibodies, which consisted mainly of β strands. 2GHW was selected as the final template (among the first 5 best homologs) due to its overall % of sequence identity with the target scFv (~75%), its good similarity in CDRs length (see ) and for its quite good resolution (2.30 Å).
Loading of the WZ1–14.2.1 row sequence in the SwissPdb-Model server,Citation24 together with the 3D structure of the 2GHW protein and their sequence alignment, enabled automatic building of a theoretical 3D model for the esterase scFv, which was then refined on the SwissPdb-Model server.
A protein structure comparison between our modeled and 2GHW template structure using the secondary structure matching analysis toolCitation25 confirmed that the B chain of 2GHW structure is a very good template for model building, with a Q score (quality function of Cα alignment) between the modeled query and 2GHW (chain B) of 0.873. Similarly, very high values of the P score (44.93) and Z score (20.6), which measures the statistical significance of a match in terms of Gaussian statistics, were found.
Molecular docking
Swiss dock server was used to find the possible pockets able to allocate the ligands of interest on the entire surface of the hypothesized 3D model. Swiss dock identified mainly four sites for our ligands. Among these four regions just one is localized on the CDRs zone supposed to be responsible for the scFv activity.
Moreover, putative catalytic sites potentially able to explain its cholinesterase activity were searched. In particular, a domain comprising residues relevant for cholinesterase activity, as that formed by Ser, His and Glu (known as “catalytic triad” from the literatureCitation26) in Chase enzymes, and residues able to engage a π-cation interaction with the cationic head of the cholinic compounds were searched.
Intramolecular H-bonds between triads and dyads of amino acids enhances the nucleophilicity of certain side chains responsible for enzymatic catalysis. Examples are the hydroxyl side chains of Ser, Thr, and Tyr residues activated by spatially neighboring general bases contributed by His, Lys, Arg, Tyr, Glu, and Asp residues.Citation27
We screened the hypothesized WZ-14.2.1 model for side chain hydroxyls located within 4 Å of atoms that can serve as general bases. A few of possible triads and dyads fulfilling this requirement were found. Among these, an interesting site containing Ser residues spatially close to general bases, comprises a couple of Ser (Ser37 and Ser101), an Asp (Asp105), an Arg (Arg52) and two Trp residues (Trp49 and Trp107 that probably are capable to engage π-cation interaction with the cationic head of the cholinic compounds).
This putative site (), localized within the same region identified also by blind docking runs, was further investigated with Autodock programCitation28 by evaluating its capability to interact with some cholinomimetic and Achase/Bchase inhibitor compounds in order to compare theoretical and functional results. From the molecular docking protocol, the best orientation and conformation of acetylthiocholine within the putative catalytic site were obtained and reported in . For all the three Ellmann substrates, acetylcholine and the Chase inhibitors used, the values derived from docking calculations are reported in .
Table 2. The measured quantities for each ligand after molecular docking calculation
Discussion
As early as the 1940s, Linus PaulingCitation29 proposed antibodies as molecules endowed with catalytic activity and, nearly 40 y later, this hypothesis was proven to be correct.Citation30,Citation31 According to Pauling, if the antigen binding site structure comes from random molecular events, the problem of isolating an antibody with desired ligand or catalytic properties reduces to the way its copy number can be increased.
First attempts to obtain catalytic antibodies were made by exposing the immune system to molecules mimicking the reaction transition state. This strategy was largely successful and has been used to obtain many antibodies accelerating a large number of reactions.Citation32 In spite of this success, the catalytic efficiency of the elicited abzymes was generally poor, their accelerating properties being about 10 000 times less than that observed for natural enzymes. An abzyme selection based on the investigation of the binding activity against transition state analogs of the natural substrate, even if successful, might lead to an artificial enzyme with reaction mechanism, active site geometry and sensitivity to inhibitors, very close to those of the natural enzyme. This may also occur when abzymes are obtained as idiotypic or anti-idiotypic antibodies against the enzyme, as observed for AChE-like antibodies.Citation33-Citation36
These were the reasons why we changed the experimental approach to the isolation of an AchE-like scFv, integrating the screening by genetic selectionCitation37,Citation38 with the bait-and-switch strategyCitation39,Citation40 for the elicitation of antibody catalysts. Phage libraries represent an excellent tool for antibody selection as demonstrated by the drugs already produced on the market.Citation41 Selection from these libraries is generally realized by exposing phages to the target, while WZ1–14.2.1 was obtained from the amber codon non-suppressor E. coli strain HB2151, infected with the Tomlinson I + J library, after exposure to culture conditions enabling the contemporaneous genetic and bait-and-switch selection. Libraries of scFv are large repertoires of randomly shuffled genes and they perform as chaotic systems when exposed to an attractive condition (i.e., a selective source of reduced carbon), enabling genetic selection. This leads the randomness of the recombining sequences to give rise to a deterministic effect, e.g., the selection of a single gene or a few genes with the expected property, i.e., substrate catalysis. On the other hand, succinylcholine was chosen as the substrate for the selection of the expected enzymatic activity due to its covalent structure. The bait-and-switch methodology involves the use of a charged hapten that selects for a complementary charged residue in the antibody-combining site. If the hapten is positively charged, the selected anion residue may act as general base in catalysis; however, anion haptens or molecules bearing a localized electron cloud may also be excluded from the binding site. This is the case of organophosphates that, through their phosphorus atom, can act as a reactive electrophile against the primary alcohol of the active site Ser of the enzyme, establishing covalent binding to electronegative elements such as O, S, and F. In this respect, succinylcholine can be regarded as acetylcholine at the mirror, due to symmetry around its center. As a consequence, its binding to a candidate active site will imply the accommodation of a quaternary ammonium cation, irrespective of the conformation or direction the molecule presents itself for binding. This strategy was used in the bait-and-switch methodological approach to obtain our second aim, i.e., resistance to inhibitors.
The experimental data obtained by testing the WZ1–14.2.1 enzymatic activity against acetylthiocholine, propionylthiocholine and butyrylthiocholine in the Ellmann assay demonstrated that the scFv has an abzyme activity with a Michaelis–Menten kinetics. This finding is in agreement to evidence previously reported for other AChE-like antibodies,Citation33-Citation35 but in contrast with other known abzymes deriving from the selection against transition state analogs; for these latter, the Michaelis–Menten kinetics does not apply due to the affinity the abzymes still manifest against the reaction product.
Although the Km values of WZ1–14.2.1 are high, the concentration levels are in line with those reported for ACh in the neuromuscular junction space during a single quantal event (i.e., 1–10 mM).Citation42 No data on the ACh concentrations in the synaptic cleft after AChE inhibitor exposure has been reported in the literature; however, a meta-analysis evaluating results obtained in rat brain studies indicates that this value can increase up to 350-fold over baseline.Citation43 Moreover, the catalytic efficiency of WZ1–14.2.1 is good, accelerating the spontaneous hydrolysis of about 107 times.
The enzymatic specificity of WZ1–14.2.1 appears to be more similar to that of BChE than AChE. Indeed, AChE does not catalyze the hydrolysis of choline esters, having an acyl chain more bulky than ACh.Citation44 Moreover, WZ1–14.2.1 is not a pure thioesterase because it can also hydrolyse Ach.
Within our 3D proposed model, docking analysis identified a probable catalytic site similar to that occurring in cholinesterase enzymes and other hydrolases. Evaluating the distances between the residues in the putative active site of WZ1–14.2.1 and the ester group of the ligands, the Ser37 appeared to have a distance compatible with an effective interaction. Site-directed mutagenesis and crystallization studies, however, are needed to confirm the hypothetical identification from 3D modeling of amino acid residues involved in catalysis.
One of the most important findings of the current study is that the BChE-like activity of WZ1–14.2.1 is resistant to different AChE inhibitors. Indeed, neostigmine and all tested organophosphates (i.e., chlorpyrifos oxon, dichlorvos, iso-OMPA, and paraoxon ethyl) did not inhibit, over a large range of concentrations, the acetylthiocholine hydrolysis catalyzed by WZ1–14.2.1. None of them was then able to bind to or react with the active site of the recombinant enzyme, in line with results obtained by challenging them against the hypothesized 3D model of WZ1–14.2.1.
According to the BChE-like activity of WZ1–14.2.1, a significant inhibition was instead observed with the selective BChE inhibitor ethopropazine. This is a bulky molecule able to bind to the anionic site of BChE, but not to that of AChE because of a steric occlusion in the acyl-binding pocket.Citation26 This phenothiazine compound shows interaction energy better than the tested Ellman substrates and it may engage π-cation interactions with Trp107 and salt bridges with Asp105 in our 3D model.
No evidence that WZ1–14.2.1 is active at the neuromuscular junction in vivo has been obtained in the current study; however, the low molecular size could facilitate the WZ1–14.2.1 tropism from circulation to the motor synapse. In line with this notion, patients who develop myasthenia gravis have circulating 150 kDa autoantibodies that reach the neuromuscular junction, bind to the acetylcholine receptor, and block cholinergic transmission.Citation45-Citation47 Finally, our experiments demonstrated that WZ1–14.2.1 may function in physiologic conditions (pH 7.4, 37 °C) we reproduced in the modified Ellmann assay. On the other hand, the mass/activity ratio (i.e., the specific activity of WZ1–14.2.1) is probably inappropriate for its clinical use, due to the need of administering the active principle in the order of grams/dose, been the abzyme more than one-thousand weaker in terms of kcat than acetylcholinesterase. Future site-directed mutagenesis experiments could suggest amino acid substitutions to circumvent this inherent limitation.
Overall, in the present study we used a new strategy for selection of a BChE-like abzyme resistant to AChE inhibitors. Our results may open the way to exploitation of alternate geometries in the active sites of AChE-like catalytic proteins, and reveal new approaches for improved treatment of poisoning by AChE inhibitors.
Materials and Methods
Chemicals and reagents
The following reagents and chemicals were obtained from Sigma: LB powder, Electrophorus Electricus AchE, acetylthiocholine chloride, butyrylthiocholine chloride, propionylthiocholine chloride, chlorpyrifos oxon, ethopropazine chloride (10-[2-diethylaminopropyl]phenothiazine), neostigmine bromide, iso-OMPA (tetraisopropyl pyrophosphoramide), paraoxon ethyl, dichlorvos, phenylmethylsulphonyl fluoride (PMSF), ampicillin, chloramphenicol, IPTG (isopropylthio-β-galactoside); succinylcholine chloride, succinic acid, DTNB (5,5′-dithiobis[2-nitrobenzoic] acid), glycerol, imidazole; chaps ([3-{3-cholamidopropyl}dimethyl ammonium]-1-propansulphonate).
Library and culture conditions
The Tomlinson I + J libraries, obtained from MRC gene service (Cambridge, UK) were used for selection. The Escherichia coli strain TG1 (K12 [lac-proAB] supE thi hadD5/F’ traD36 proA + B lacIq lacZM15), the strain HB2151(K12 ara [lac-proAB] thi/F’proA + B lacIq lacZM15) and the helper phage KM13 were part of the libraries;Citation48 both libraries, constructed in the ampicillin resistant phagemid vector pIT2, are based on a single human framework for VH and VL and their variety is represented by about 1.4 × 108 different scFv fragments, each one comprising a single polypeptide with the VH and VL domains linked to one another by a flexible Gly-Ser sequence.
The growth of Escherichia coli cells and expansion of phage K13 were according to the protocol obtained by MRC (http://www.lifesciences.sourcebioscience.com/media/143421/tomlinsonij.pdfhttp://www.lifesciences.sourcebioscience.com/media/143421/tomlinsonij.pdf).
After recovery of the scFv repertoire from the TG1 libraries with the helper phage KM13, the Su-E. Coli strain HB2151 were infected for expression of soluble scFv fragments avoiding any panning procedure. Transformed HB2151 cell library was then grown in an agar selective medium, containing 100 μg/mL ampicillin, 1 mM IPTG for induction, and succinylcholine chloride (100 μg/mL; ) as the sole source of reduced carbon. Indeed, both colonies obtained following two rounds of selection and the transformed HB2151 whole library were unable to survive when succinylcholine chloride addition was omitted or choline was added instead of succinylcholine. On the other hand, non-transformed HB2151 E. coli cells do not survive in the selective medium, revealing themselves to be unable to survive at the non-catalyzed rate of spontaneous hydrolysis of succinylcholine in water and to be devoid of any esterase activity providing succinate from succinylcholine. The scFv insert was amplified by colony PCR and sequenced.
Insertion of scFv gene construct in a new vector and cell hosts
Isolation of the pIT2 phagemid vector was performed using the QIAGEN Plasmid Isolation Kit (QIAGEN). The target gene was then recombined into the pET-22b(+) vector (Novagen Cat. No. 69744–3), which carries an N-terminal pelB signal sequence for potential periplasmic localization plus an optional C-terminal His•Tag® sequence. The new vector was used to transform the non-expression host BL21(DE3) (Novagen Cat. No. 69387) for gene internalization and, following gene sequencing and assessment of the appropriate reading frame, the expression host Rosetta™(DE3)pLysS (Novagen Cat. No. 70956) for protein production.
ScFv purification
Protein was extracted from 1L LB medium after the Rosetta culture reached OD600 1.6–2. Cells were collected, frozen at -20 °C, thawed and sonicated in 50 mM TRIS-HCl pH 7.4, 0.1 mM NaCl, 2 mM EDTA buffer, on ice to minimise heat denaturation. HisTrap™ FF 1 mL column (GE Healthcare Cat. No. 17–5319–01) was used to purify the His-tagged recombinant protein, according to the manufacturer instruction. The high imidazole concentration used to elute the tagged protein was completely removed by using repeatedly HiTrapTM Desalting 5 mL columns (GE Healthcare Cat No. 17–1408–01) at 5 mL/min flow rate following the manufacturer protocol. The purified protein was eluted with 100 mM pH 7.4 phosphate buffer. The purification process was monitored by SDS-PAGE and activity assays; MALDI-TOF analysis of the purified protein was performed by an external service.
Cholinesterase activity assay
Cholinesterase-like activity was tested in 96 well plates according to Ellman assay,Citation49 slightly modified (T 37 °C; pH 7.4). The enzyme kinetics was evaluated by progress curves using as substrates not only acetylthiocholine but also propionylthiocholine and butyrylthiocholine in order to test the enzyme specificity. Enzyme activity vs substrate concentration assays were plotted, measuring the formation of the yellow product nitrobenzoate anion through its 412 nM absorbance by Infinite® 200 PRO NanoQuant instrument (Tecan).
A further AChE assay, the Amplex®Red acetylcholine/acetylcholinesterase assay kit (Life Technologies Cat. No. A12217), was performed to confirm the acetylcholine esterase activity by using the real physiologic substrate acetylcholine, according to the manufacturer instruction. Excitation at 530 nm and absorption at 590 nm were obtained with VictorTMX3 plate reader Instrument (Perkin Elmer).
The Kcat and Km were determined by fitting progress data to a Michaelis-Menten equation with the program software GraphPad®Prism 4.0, each data point deriving from three separate experiments. Lineweaver-Burk plots of data were performed to assess the linearity of the function (through the Fisher-Z statistics), i.e., applicability of the Michaelis-Menten model to the experimental data. When the inhibitors were used, they were added to the enzyme mix at 37 °C, 30 min prior substrate addition. V0 was calculated using the DTNB extinction coefficient of 1.38x10−4 M−1 cm−1 at 37 °C.Citation50
The background hydrolysis (Kuncat) was determined by initial rate analysis and extrapolated to zero-buffer concentration. When inhibitors were soluble in solvent different from phosphate buffer, blank kinetics with solvent instead drug solution were added in the experimental protocol.
Control Ellman experiments confirming the activity of AchE inhibitors vs a commercially AchE were performed as internal controls.
Data were plotted as mean ± SEM from three separate experiments. Statistical comparisons were done by Student t test for paired data and a P < 0.05 was taken to be significant.
Molecular modeling studies
Template selection and homology modeling
Possible homologies between WZ-14.2.1 and other scFv deposited sequences were investigated by BLAST runs on the NCBI website (http://blast.ncbi.nlm.nih.gov/Blast.cgi), using the default threshold E-value of 10 and inclusion threshold value of 0.005, against Protein Data Bank (PDB) (http://www.rcsb.org/pdb/home/home.do), Swissprot (http://www.uniprot.org/) and non-redundant sequence databases. The first five best results predicted by BLAST were analyzed.
Multiple sequence alignment and secondary structure prediction
Multiple sequence alignment was performed to check the positions of the CDRs in the templates and in the query. Multiple sequence alignment of WZ-14.2.1 and its selected homologous was performed using CLUSTALW.Citation21 Automatic alignments were critically analyzed and compared with each other. Since scFv antibodies are known to have β structures, in order to confirm whether our query sequence also possessed this type of secondary structure, its secondary structure was predicted by the Jpred 3 program.Citation23
Blind docking and docking
When the location of the binding site is unknown, is often useful to examine the possible sites through “blind docking,” which explores the entire surface of the protein and localizes the probable cavities allowed for ligand binding. Swiss dock serverCitation51 was used to find the possible cavities able to allocate the ligands of interest on the entire surface of the WZ-14.2.1 3D model. Within the identified possible cavities, triads and dyads capable of explaining catalytic activity were searched. Once the region most probable for ligand binding was selected, more detailed molecular docking runs were performed with Autodock 4.0,Citation28 focusing on the search of the best orientation and conformation of substrates and inhibitors within the selected putative binding site obtained in the previous step.
The polar hydrogens and united atom Kollman charges were assigned to the enzymes during the preparation of the protein input files, containing fragmental volume and solvation parameters. For the ligands, partial atomic charges were determined by the Gasteiger methodCitation52 with modification, to ensure unit charge on each residue. Moreover, rotatable bonds were assigned with AutoDockTools, an accessory program that allows the user to interact with AutoDock from a graphic user interface. Prior to the AutoDock, AutoGrid was exploited for the preparation of the grid map using a grid box with a npts (number of points in xyz) of 50–60–50 Å, which defines the simulation space. The box spacing was 0.375 Å and the grid was set to cover the entire space of the binding site. A distance-dependent function of the dielectric constant was used for the calculation of the energetic maps. AutoDock was run using the maximum number of energy evaluations retries and generations, of 10 000 and 27 000, respectively. The Lamarckian genetic algorithm (LGA) with the pseudo-Solis and Wets modification (LGA/pSW) method was used with default parameters for calculation of the docking possibilities.Citation53
Abbreviations: | ||
Ach | = | acetylcholine |
AChE | = | acetylcholinesterase |
ALA | = | alanine |
ASP | = | asparagine, BChE, butyrylcholinesterase |
CDRs | = | complementarity-determining regions |
chaps | = | 3-(3-cholamidopropyl)dimethyl ammonium]-1-propansulphonate |
Chase | = | cholinesterase |
Cys | = | cysteine |
DTNB | = | 5,5′-dithiobis(2-nitrobenzoic) acid |
EDTA | = | ethylenediaminetetraacetic acid |
GLU | = | glutamate |
HI-6 | = | 2-hydroxyiminomethyl-4’-carbamoyl-piridinium-1’-methylether dichloride monohydrate |
His | = | Histidine |
iso-OMPA | = | tetraisopropyl pyrophosphoramide |
IC50 | = | inhibitory concentration by 50% |
IPTG | = | isopropylthio-β-galactoside |
LB medium | = | Luria Broth medium |
LGA/pSW | = | Lamarckian genetic algorithm/ pseudo-Solis and Wets modification |
Leu | = | leucine |
pI | = | isoelectric point |
MALDI/TOF | = | matrix-assisted laser desorption/ionization/time of flight |
Ni | = | Nickel |
OD | = | optical density, PMSF, phenylmethanesuphonyl fluoride |
scFv | = | single chain variable fragment |
SDS-PAGE | = | sodium dodecyl sulfate - polyacrylamide gel electrophoresis |
Ser | = | serine |
Tyr | = | tyrosine |
Trp | = | tryptophan |
Potential Conflicts of interest
No potential conflicts of interest were disclosed.
Acknowledgments
A grant from Tuscany Region (Italy) allowed the characterization of the protein after its isolation.
References
- Taylor P, Radic Z, Hosea NA, Camp S, Marchot P, Berman HA. Structural bases for the specificity of cholinesterase catalysis and inhibition. Toxicol Lett 1995; 82-83:453 - 8; http://dx.doi.org/10.1016/0378-4274(95)03575-3; PMID: 8597093
- Shafferman A, Ordentlich A, Barak D, Stein D, Ariel N, Velan B. Aging of phosphylated human acetylcholinesterase: catalytic processes mediated by aromatic and polar residues of the active centre. Biochem J 1996; 318:833 - 40; PMID: 8836126
- Aurbek N, Herkert NM, Koller M, Thiermann H, Worek F. Kinetic analysis of interactions of different sarin and tabun analogues with human acetylcholinesterase and oximes: is there a structure-activity relationship?. Chem Biol Interact 2010; 187:215 - 9; http://dx.doi.org/10.1016/j.cbi.2010.01.035; PMID: 20105433
- Worek F, Reiter G, Eyer P, Szinicz L. Reactivation kinetics of acetylcholinesterase from different species inhibited by highly toxic organophosphates. Arch Toxicol 2002; 76:523 - 9; http://dx.doi.org/10.1007/s00204-002-0375-1; PMID: 12242610
- Bird SB, Dawson A, Ollis D. Enzymes and bioscavengers for prophylaxis and treatment of organophosphate poisoning. [Schol Ed] Front Biosci (Schol Ed) 2010; 2:209 - 20; http://dx.doi.org/10.2741/s58; PMID: 20036941
- Trovaslet-Leroy M, Musilova L, Renault F, Brazzolotto X, Misik J, Novotny L, Froment MT, Gillon E, Loiodice M, Verdier L, et al. Organophosphate hydrolases as catalytic bioscavengers of organophosphorus nerve agents. Toxicol Lett 2011; 206:14 - 23; http://dx.doi.org/10.1016/j.toxlet.2011.05.1041; PMID: 21683774
- Kurkova IN, Smirnov IV, Belogurov AA Jr., Ponomarenko NA, Gabibov AG. Creation of catalytic antibodies metabolizing organophosphate compounds. Biochemistry (Mosc) 2012; 77:1139 - 46; http://dx.doi.org/10.1134/S0006297912100069; PMID: 23157294
- Otto TC, Scott JR, Kauffman MA, Hodgins SM, Ditargiani RC, Hughes JH, Sarricks EP, Saturday GA, Hamilton TA, Cerasoli DM. Identification and characterization of novel catalytic bioscavengers of organophosphorus nerve agents. Chem Biol Interact 2013; 203:186 - 90; http://dx.doi.org/10.1016/j.cbi.2012.09.009; PMID: 23041042
- Smirnov I, Belogurov A Jr., Friboulet A, Masson P, Gabibov A, Renard PY. Strategies for the selection of catalytic antibodies against organophosphorus nerve agents. Chem Biol Interact 2013; 203:196 - 201; http://dx.doi.org/10.1016/j.cbi.2012.10.011; PMID: 23123255
- Petrikovics I, McGuinn WD, Sylvester D, Yuzapavik P, Jiang J, Way JL, Papahadjopoulos D, Hong K, Yin R, Cheng TC, et al. In vitro studies on sterically stabilized liposomes (SL) as enzyme carriers in organophosphorus (OP) antagonism. Drug Deliv 2000; 7:83 - 9; http://dx.doi.org/10.1080/107175400266641; PMID: 10892408
- Szilasi M, Budai M, Budai L, Petrikovics I. Nanoencapsulated and microencapsulated enzymes in drug antidotal therapy. Toxicol Ind Health 2012; 28:522 - 31; http://dx.doi.org/10.1177/0748233711416946; PMID: 21986886
- Cohen O, Kronman C, Raveh L, Mazor O, Ordentlich A, Shafferman A. Comparison of polyethylene glycol-conjugated recombinant human acetylcholinesterase and serum human butyrylcholinesterase as bioscavengers of organophosphate compounds. Mol Pharmacol 2006; 70:1121 - 31; http://dx.doi.org/10.1124/mol.106.026179; PMID: 16801396
- Masson P, Lockridge O. Butyrylcholinesterase for protection from organophosphorus poisons: catalytic complexities and hysteretic behavior. Arch Biochem Biophys 2010; 494:107 - 20; http://dx.doi.org/10.1016/j.abb.2009.12.005; PMID: 20004171
- Mumford H, Docx CJ, Price ME, Green AC, Tattersall JE, Armstrong SJ. Human plasma-derived BuChE as a stoichiometric bioscavenger for treatment of nerve agent poisoning. Chem Biol Interact 2013; 203:160 - 6; http://dx.doi.org/10.1016/j.cbi.2012.08.018; PMID: 22981459
- Cerasoli DM, Griffiths EM, Doctor BP, Saxena A, Fedorko JM, Greig NH, Yu QS, Huang Y, Wilgus H, Karatzas CN, et al. In vitro and in vivo characterization of recombinant human butyrylcholinesterase (Protexia) as a potential nerve agent bioscavenger. Chem Biol Interact 2005; 157-158:363 - 5; http://dx.doi.org/10.1016/j.cbi.2005.10.052; PMID: 16429486
- Mumford H, Troyer JK. Post-exposure therapy with recombinant human BuChE following percutaneous VX challenge in guinea-pigs. Toxicol Lett 2011; 206:29 - 34; http://dx.doi.org/10.1016/j.toxlet.2011.05.1016; PMID: 21620937
- Petroianu GA, Arafat K, Nurulain SM, Kuca K, Kassa J. In vitro oxime reactivation of red blood cell acetylcholinesterase inhibited by methyl-paraoxon. J Appl Toxicol 2007; 27:168 - 75; http://dx.doi.org/10.1002/jat.1189; PMID: 17265452
- Prasad SB, Kumar YC, Kumar CS, Sadashiva CT, Vinaya K, Rangappa KS. Synthesis of Novel 3-Aryl-N-Methyl-1,2,5,6-Tetrahydropyridine Derivatives by Suzuki coupling: As Acetyl Cholinesterase Inhibitors. Open Med Chem J 2007; 1:4 - 10; http://dx.doi.org/10.2174/1874104500701010004; PMID: 19662135
- Malik A, Firoz A, Jha V, Sunderasan E, Ahmad S. Modeling the three-dimensional structures of an unbound single-chain variable fragment (scFv) and its hypothetical complex with a Corynespora cassiicola toxin, cassiicolin. J Mol Model 2010; 16:1883 - 93; http://dx.doi.org/10.1007/s00894-010-0680-1; PMID: 20232097
- Berman HM, Battistuz T, Bhat TN, Bluhm WF, Bourne PE, Burkhardt K, Feng Z, Gilliland GL, Iype L, Jain S, et al. The protein data bank. Acta Crystallogr D Biol Crystallogr 2002; 58:899 - 907; http://dx.doi.org/10.1107/S0907444902003451; PMID: 12037327
- Thompson JD, Higgins DG, Gibson TJ. CLUSTAL W: improving the sensitivity of progressive multiple sequence alignment through sequence weighting, position-specific gap penalties and weight matrix choice. Nucleic Acids Res 1994; 22:4673 - 80; http://dx.doi.org/10.1093/nar/22.22.4673; PMID: 7984417
- Sivasubramanian A, Sircar A, Chaudhury S, Gray JJ. Toward high-resolution homology modeling of antibody Fv regions and application to antibody-antigen docking. Proteins 2009; 74:497 - 514; http://dx.doi.org/10.1002/prot.22309; PMID: 19062174
- Cole C, Barber JD, Barton GJ. The Jpred 3 secondary structure prediction server. Nucleic Acids Res 2008; 36:W197 201; http://dx.doi.org/10.1093/nar/gkn238; PMID: 18463136
- Arnold K, Bordoli L, Kopp J, Schwede T. The SWISS-MODEL workspace: a web-based environment for protein structure homology modelling. Bioinformatics 2006; 22:195 - 201; http://dx.doi.org/10.1093/bioinformatics/bti770; PMID: 16301204
- Krissinel E, Henrick K. Secondary-structure matching (SSM), a new tool for fast protein structure alignment in three dimensions. Acta Crystallogr D Biol Crystallogr 2004; 60:2256 - 68; http://dx.doi.org/10.1107/S0907444904026460; PMID: 15572779
- Ordentlich A, Barak D, Kronman C, Flashner Y, Leitner M, Segall Y, Ariel N, Cohen S, Velan B, Shafferman A. Dissection of the human acetylcholinesterase active center determinants of substrate specificity. Identification of residues constituting the anionic site, the hydrophobic site, and the acyl pocket. J Biol Chem 1993; 268:17083 - 95; PMID: 8349597
- Taguchi H, Planque S, Sapparapu G, Boivin S, Hara M, Nishiyama Y, Paul S. Exceptional amyloid beta peptide hydrolyzing activity of nonphysiological immunoglobulin variable domain scaffolds. J Biol Chem 2008; 283:36724 - 33; http://dx.doi.org/10.1074/jbc.M806766200; PMID: 18974093
- Morris GM, Goodsell DS, Halliday RS, Huey R, Hart WE, Belew RK, Olson AJ. Automated Docking Using a Lamarckian Genetic Algorithm and Empirical Binding Free Energy Function. J Comput Chem 1998; 19:1639 - 62; http://dx.doi.org/10.1002/(SICI)1096-987X(19981115)19:14<1639::AID-JCC10>3.0.CO;2-B
- Pauling L. Antibodies and specific biological forces. Endeavour 1948; 7:43 - 53; PMID: 18916790
- Pollack SJ, Jacobs JW, Schultz PG. Selective chemical catalysis by an antibody. Science 1986; 234:1570 - 3; http://dx.doi.org/10.1126/science.3787262; PMID: 3787262
- Tramontano A, Janda KD, Lerner RA. Catalytic antibodies. Science 1986; 234:1566 - 70; http://dx.doi.org/10.1126/science.3787261; PMID: 3787261
- Nieri P, Donadio E, Rossi S, Adinolfi B, Podestà A. Antibodies for therapeutic uses and the evolution of biotechniques. Curr Med Chem 2009; 16:753 - 79; http://dx.doi.org/10.2174/092986709787458380; PMID: 19199935
- Izadyar L, Friboulet A, Remy MH, Roseto A, Thomas D. Monoclonal anti-idiotypic antibodies as functional internal images of enzyme active sites: production of a catalytic antibody with a cholinesterase activity. Proc Natl Acad Sci U S A 1993; 90:8876 - 80; http://dx.doi.org/10.1073/pnas.90.19.8876; PMID: 8415624
- Johnson G, Moore SW. Catalytic antibodies with acetylcholinesterase activity. J Immunol Methods 2002; a 269:13 - 28; http://dx.doi.org/10.1016/S0022-1759(02)00222-3; PMID: 12379349
- Johnson G, Moore SW. Idiotypic mimicry of a catalytic antibody active site. Mol Immunol 2002; b 39:273 - 88; http://dx.doi.org/10.1016/S0161-5890(02)00113-X; PMID: 12220886
- Franqueville E, Stamatis H, Loutrari H, Friboulet A, Kolisis F. Studies on the catalytic behaviour of a cholinesterase-like abzyme in an AOT microemulsion system. J Biotechnol 2002; 97:177 - 82; http://dx.doi.org/10.1016/S0168-1656(02)00061-5; PMID: 12067523
- Wahler D, Reymond JL. Novel methods for biocatalyst screening. Curr Opin Chem Biol 2001; 5:152 - 8; http://dx.doi.org/10.1016/S1367-5931(00)00184-8; PMID: 11282341
- Soumillion P, Fastrez J. Novel concepts for selection of catalytic activity. Curr Opin Biotechnol 2001; 12:387 - 94; http://dx.doi.org/10.1016/S0958-1669(00)00232-9; PMID: 11551468
- Shokat KM, Schultz PG. Catalytic antibodies. Methods Enzymol 1991; 203:327 - 51; http://dx.doi.org/10.1016/0076-6879(91)03019-D; PMID: 1762563
- Janda KD. New strategies for the design of catalytic antibodies. Biotechnol Prog 1990; 6:178 - 81; http://dx.doi.org/10.1021/bp00003a002; PMID: 1366610
- Nixon AE, Sexton DJ, Ladner RC. Drugs derived from phage display: From candidate identification to clinical practice. MAbs 2013; 6; Forthcoming PMID: 24262785
- Smart JL, McCammon JA. Analysis of synaptic transmission in the neuromuscular junction using a continuum finite element model. Biophys J 1998; 75:1679 - 88; http://dx.doi.org/10.1016/S0006-3495(98)77610-6; PMID: 9746510
- Noori HR, Fliegel S, Brand I, Spanagel R. The impact of acetylcholinesterase inhibitors on the extracellular acetylcholine concentrations in the adult rat brain: a meta-analysis. Synapse 2012; 66:893 - 901; http://dx.doi.org/10.1002/syn.21581; PMID: 22733599
- Saxena A, Redman AM, Jiang X, Lockridge O, Doctor BP. Differences in active-site gorge dimensions of cholinesterases revealed by binding of inhibitors to human butyrylcholinesterase. Chem Biol Interact 1999; 119-120:61 - 9; http://dx.doi.org/10.1016/S0009-2797(99)00014-9; PMID: 10421439
- Newsom-Davis J, Pinching AJ, Vincent A, Wilson SG. Function of circulating antibody to acetylcholine receptor in myasthenia gravis: investigation by plasma exchange. Neurology 1978; 28:266 - 72; http://dx.doi.org/10.1212/WNL.28.3.266; PMID: 564482
- De Baets M, Stassen MH. The role of antibodies in myasthenia gravis. J Neurol Sci 2002; 202:5 - 11; http://dx.doi.org/10.1016/S0022-510X(02)00200-9; PMID: 12220686
- Hughes BW, Moro De Casillas ML, Kaminski HJ. Pathophysiology of myasthenia gravis. Semin Neurol 2004; 24:21 - 30; http://dx.doi.org/10.1055/s-2004-829585; PMID: 15229789
- de Wildt RM, Mundy CR, Gorick BD, Tomlinson IM. Antibody arrays for high-throughput screening of antibody-antigen interactions. Nat Biotechnol 2000; 18:989 - 94; http://dx.doi.org/10.1038/79494; PMID: 10973222
- Ellman GL, Courtney KD, Andres V Jr., Feather-Stone RM. A new and rapid colorimetric determination of acetylcholinesterase activity. Biochem Pharmacol 1961; 7:88 - 95; http://dx.doi.org/10.1016/0006-2952(61)90145-9; PMID: 13726518
- Eyer P, Worek F, Kiderlen D, Sinko G, Stuglin A, Simeon-Rudolf V, Reiner E. Molar absorption coefficients for the reduced Ellman reagent: reassessment. Anal Biochem 2003; 312:224 - 7; http://dx.doi.org/10.1016/S0003-2697(02)00506-7; PMID: 12531209
- Grosdidier A, Zoete V, Michielin O. SwissDock, a protein-small molecule docking web service based on EADock DSS. Nucleic Acids Res 2011; 39:W270 7; http://dx.doi.org/10.1093/nar/gkr366; PMID: 21624888
- Gasteiger J, Marsili M. Iterative Partial Equalization of Orbital Electronegativity - A Rapid Access to Atomic Charges. Tetrahedron 1980; 36:3219 - 28; http://dx.doi.org/10.1016/0040-4020(80)80168-2
- Solis FJ, Wets RJB. Minimization by random search techniques. Math Operat Res 1981; 6:19 30; http://dx.doi.org/10.1287/moor.6.1.19