Abstract
The first full length IgG produced in Pichia pastoris was reported in late 1980. However, use of a wild-type Pichia expression system to produce IgGs with human-like N-linked glycans was not possible until recently. Advances in glycoengineering have enabled organisms such as Pichia to mimic human N-glycan biosynthesis and produce IgGs with human glycans on an industrial scale. Since there are only a few reports of the analytical characterization of Pichia-produced IgG, we summarize the results known in this field, and provide additional characterization data generated in our laboratories. The data suggest that Pichia-produced IgG has the same stability as that produced in Chinese hamster ovary (CHO) cells. It has similar aggregation profiles, charge variant distribution and oxidation levels as those for a CHO IgG. It contains human N-linked glycans and O-linked single mannose. Because of the comparable biophysical and biochemical characteristics, glycoengineered Pichia pastoris is an attractive expression system for therapeutic IgG productions.
Acknowledgments
We gratefully thank Assunta Ng for providing SEC aggregation data, Anna Mach for CE-SDS data, Feng Wang for CEX data, Brian Peklansky for cIEF data, Yi Du for ESI-TOF data, Bei Wang for DSC data, Yunsong (Frank) Li for CD data and Sarita Mittal for chemical degradation data. We also would like to thank our colleagues from GlycoFi for providing technical assistance and colleagues from BPRD for providing all IgG materials.
Figures and Tables
Figure 1 The schematic outline of N-glycan biosynthesis pathway in glycoengineered Pichia pastoris that mimics human N-glycan synthesis. MnsI: α-1,2-mannosidase; GnTI: β-1,2-N-acetylglucosaminyltransferase I; MnsII: mannosidase II; GnTII: β-1,2-N-acetylglucosaminyltransferase II; GalT: β-1,4-galactosyltransferase. ○: GlcNAc; □: Man; ◊: Gal. To simplify nomenclature, the two GlcNAc sugars at the reducing end of all glycans are omitted.
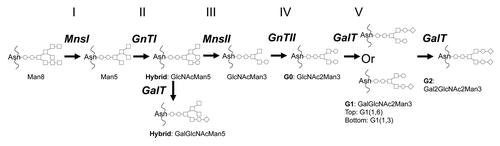
Figure 2 Size exclusion chromatography (SEC) profiles of IgG1 produced from CHO cell lines (upper trace) and from Pichia (lower trace). Insert is a zoomed view of the SEC profiles. Peak eluting at 16 min represents the IgG monomer, while peak at 13.5 min represents the aggregate and peak at 20 min represents the fragment. In both cases, the percentage of the monomer is above 99%, and the aggregate and fragment are below 0.5% individually. SEC was performed using a TSK-gelG3000SW column with UV detection at 280 nm and flow rate of 0.5 mL/min. The mobile phase contained 25 mM sodium phosphate, 300 mM sodium chloride and 0.05% sodium azide at pH 6.8.
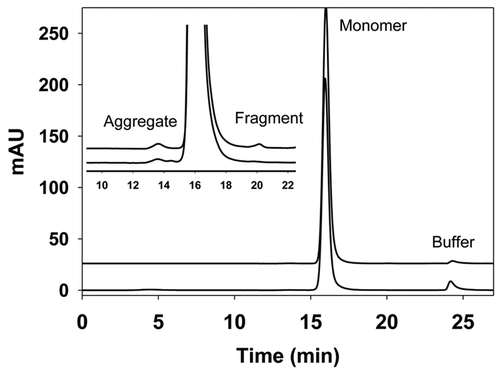
Figure 3 (A) Charge heterogeneity of IgG1 revealed by cation exchange chromatography. In this assay, the Pichia-produced IgG1 (lower trace) has 15% acidic variants, 84% main fraction and 1% basic variants. The CHO produced IgG1 (upper trace) has 23%, 61% and 16% acidic, main and basic fractions, respectively. The CEX separation was performed using Dionex ProPac WCX-10 column (4 × 250 mm) with a salt gradient elution and UV detection at 280 nm wavelength. The mobile phase A contained 25 mM sodium phosphate at pH 6.5 and the mobile phase B contained 25 mM sodium phosphate, 300 mM sodium chloride and 0.05% sodium azide at pH 6.5. The salt gradient elution was 4%–22% mobile phase B in 28 min at 1 mL/min flow rate. (B) Charge heterogeneity of IgG1 revealed by imaged capillary isoelectric focusing. The acidic, main and basic fractions assessed by this assay are 24, 75, 1% for Pichia-produced IgG1 (lower trace) and 33, 62, 5% for CHO-produced IgG1 (upper trace), respectively. Imaged capillary isoelectric focusing was carried out using Convergent Bioscience iCE280 analyzer. Samples were diluted to 0.25 mg/mL in a solution containing 0.35% methylcellulose, a mixture of pH 3–10 and 8–10 carrier ampholytes, and two pI markers of 7.6 and 9.5. The prepared samples were focused in a 5 cm long, 100 µm ID × 200 µm OD silica capillary and detected at 280 nm. Focusing time was 1 min at 1.5 kV then 8 min at 3 kV.
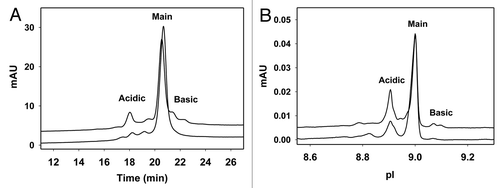
Figure 4 (A) CE-SDS gel electropherograms of IgG1 produced in CHO (lower trace) and Pichia (upper trace) under reducing condition. Both have typical IgG pattern with LC, HC and NGHC. This particular Pichia-produced IgG1 contains about 10% NGHC compared to 1% of NGHC in CHO IgG1. Samples (1 mg/mL) were reduced in beta mercaptoethanol (2-ME) containing 0.5% SDS and heated at 70°C for 10 min. CE-SDS gel was performed using Beckman PA800 CE system (220 nm detection) in bare-fused silica capillary. Sample was injected at anode with reverse polarity using -5 kV for 25 s. The separation was performed at -15 kV reverse polarity with 20 psi at both ends of capillary for 30 min. (B) CE-SDS gel electropherograms of IgG1 produced in CHO (lower trace) and Pichia (upper trace) under non-reducing condition. Both have >96% intact IgG with no covalently linked aggregates. Non-reducing samples (1 mg/mL) were treated with 25 mM iodoacetamide in the presence of 0.5% SDS and heated at 70°C for 10 min. CE separation was the same as described in reducing samples above.
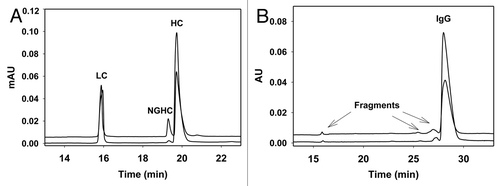
Figure 5 ESI-TOF spectra of the heavy chain in (A) CHO- and (B) Pichia-produced IgG1. As expected, Pichia-produced IgG1 has no core fucose and contains predominantly G0 glycan. Samples were reduced in the presence of 1 mM DTT at 75°C for 15 minutes, then separated on a PS-DVB column (0.5 mm × 50 mm) at 80°C with a gradient elution of mobile A and B and a flow rate of 20 µL/min. The mobile phase A contained water with 0.1% formic acid, and the mobile phase B contained acetonitrile with 0.1% formic acid. The liquid chromatography separation was coupled to an Agilent MSD-TOF MS and the mass was determined using TOF Protein Confirmation software.
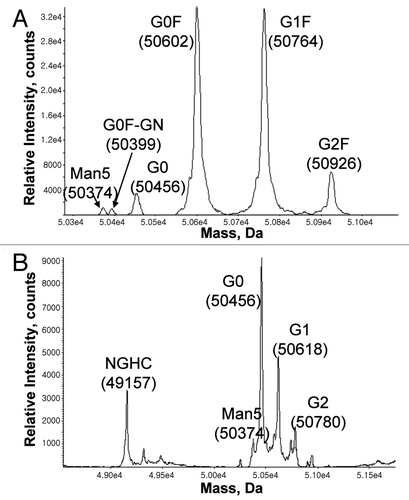
Figure 6 Far-UV (A) and near-UV (B) circular dichroism (CD) spectra of CHO- and Pichia-produced IgG1. The two antibodies have similar spectra, indicating that Pichia-produced IgG1 has similar secondary and tertiary structures compared to the CHO-produced counterpart. The CD measurements were performed on a Jasco J-810 Spectropolarimeter. Samples were dialyzed into PBS buffer and their concentrations were measured by UV. CD measurements were performed using a 1 cm quartz cuvette. A total of five spectral scans were collected at ambient temperature and signals were averaged. The buffer spectrum was collected under the same condition and was subtracted from the sample spectrum prior to the mean residue ellipticity calculation.
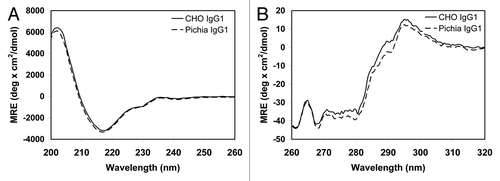
Figure 7 Temperature-induced unfolding of IgG1 produced from CHO and Pichia pastoris. CHO- and Pichia-produced IgG1 have identical Tm1 and Tm2 melting temperatures. DSC was conducted on a VP-DSC Capillary Cell Microcalorimeter with a scan rate of 1°C/min from 25°C to 95°C. The thermograms were processed using the Origin 7.0 software and normalized to the molar concentration of the IgG sample.
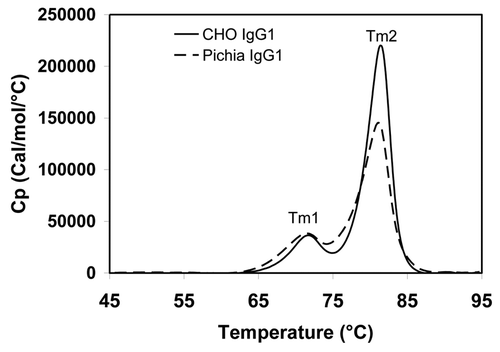