Abstract
γδ T cells contribute to the front line of lymphoid antitumor surveillance and bridge the gap between innate and adaptive immunity. They can be readily expanded to high numbers in vivo and in vitro, starting from the blood of cancer patients, and a number of Phase I trials have demonstrated that these cells can be employed in cancer immunotherapy. Sufficient patients have received γδ T cell-based immunotherapies in the context of clinical trials to evaluate their utility, and to inform the direction of new trials. A systematic approach was used to identify Phase I, Phase II, and feasibility studies testing γδ T cell-based immunotherapy in cancer patients. Studies were excluded from further analysis if they did not provide patient-specific data. Data were compiled to evaluate efficacy, with stratification by treatment approach. When possible, comparisons were made with the efficacy of second-line conventional therapeutic approaches for the same malignancy. Twelve eligible studies were identified, providing information on 157 patients who had received γδ T cell-based immunotherapy. The comparison of objective response data suggests that γδ T cell-based immunotherapy is superior to current second-line therapies for advanced renal cell carcinoma and prostate cancer, but not for non-small cell lung carcinoma. An evaluation of pooled data from 132 published in vitro experiments shows a consistent improvement in the cytotoxicity of γδ T cells in the presence of antitumor antibodies. Immunotherapy using γδ T cells alone shows promising clinical activity, but there is a strong preclinical rationale for combining this treatment modality with cancer-targeting antibodies to augment its efficacy.
Introduction
γδ T cells recognize pathogens and transformed cells in a HLA-unrestricted manner. These lymphocytes respond to markers of cellular stress including phosphoantigens, which are released by transformed cells as by-products of the mevalonate biosynthetic pathway.Citation1 Furthermore, γδ T cells share characteristics of both the innate and adaptive immune system, displaying both innate cytotoxic functions and antigen-presenting capability,Citation2,Citation3 particularly in the presence of antibody-opsonized target cells.Citation4 This dual capacity makes them an exciting candidate for cancer immunotherapy.
The most abundant subset of circulating γδ T cells, Vγ9Vδ2 cells, can be activated and expanded in vitro following a single treatment with the phosphoantigen isopentenyl pyrophosphate (IPP), with an EC50 of 3 μM.Citation5 Naturally occurring or synthetic non-peptide prenyl pyrophosphate analogs of IPP can serve as ligands of the Vγ9Vδ2 T-cell receptor (TCR), including the synthetic analog bromohydrin pyrophosphate (BrHPP, EC50 0.15 μM, from Innate Pharma, France),Citation5 which has been evaluated in Phase I and II clinical trials.Citation6,Citation7 Inhibitors of farnesyl pyrophosphate synthase (FPPS) such as the 3rd generation aminobisphosphonates zoledronate and pamidronate lead to IPP accumulation. Originally intended as inhibitors of osteoclast-mediated bone resorption for the treatment of osteoporosis and hypercalcemia, aminobisphosphonates potentially provide secondary benefit as part of γδ T cell-based immunotherapy and have been shown to be very well tolerated in combination with chemotherapy by cancer patients of all age ranges.
Similar to natural killer (NK) cells, the activation of γδ T cells is regulated by a balance between stimulatory and inhibitory signals. They can be activated by γδ TCR ligands such as phosphoantigens, or by MHC-associated ligands of the activatory receptor killer cell lectin-like receptor subfamily K, member 1 (KLRK1, best known as NKG2D, such as MHC class I polypeptide-related sequence A (MICA), MICB, and various members of the UL16-binding protein (ULBP) family. γδ T cells also express killer-cell immunoglobulin-like receptors (KIRs), which can be either activatory or inhibitory, including killer cell immunoglobulin-like receptor, 2 domains, long cytoplasmic tail, 1 (KIR2DL1)Citation8 and killer cell immunoglobulin-like receptor, 3 domains, long cytoplasmic tail, 1 (KIR3DL1).Citation9 Tumors possess the ability to manipulate this balance to stimulate tolerance by inhibitory signals, including soluble NKG2D ligands, transforming growth factor β1 (TGFβ1), galectin 3 and prostaglandin E2 (PGE2)Citation10,Citation11,Citation12,Citation13 Elevated circulating levels of sMICA, sMICB, and sULBP1 might be particularly active against effector γδ T cells, as the latter express high amounts of NKG2D. Of interest, the NKG2D ligand ULBP4 may bind to the TCR of some γδ T-cell subsets, and this may exacerbate their inhibition by neuroblastoma cells.Citation14
The balance between inhibitory and activatory signals can be tilted toward tumor control by boosting tumor-specific cytotoxic functions. With γδ T cells, this is achieved upon activation by phosphoantigens such as IPP, an effect that is exacerbated if target cells are opsonized by an appropriate antibody,Citation3,Citation4,Citation15-Citation18 making the combination of antitumor antibodies with γδ T cell-based immunotherapy an attractive therapeutic prospect.
Treatment Approaches—In Vivo Expansion Vs. Adoptive Transfer
Following the recognition that γδ T cells can be expanded to form potent antitumor effectors in vitro and in vivo, numerous clinical trials have attempted to capitalize on these properties for cancer immunotherapy. Adoptive transfer—a process that requires the expansion (and activation) of autologous T cells ex vivo and their reinfusion into patients, is becoming a popular paradigm of cellular immunotherapy. The potential to expand γδ T cells in vivo using combinations of aminobisphosphonates and cytokine offers a comparatively cheaper and more straightforward delivery alternative.
The expansion of γδ T cells ex vivo allows for the optimization and control of the effector cell population. Strategies that are currently under investigation in this sense include the use of naturally occurring and genetically-modified tumor-specific effectors. The benefits of controlling the effector cell population for adoptive transfer are significant, but must be balanced against the cost of preparing and administering the treatment. γδT cells from cancer patients can be reproducibly expanded ex vivo to large numbers using phosphoantigens,Citation19 aminobisphosphonates,Citation20 or immobilized anti-γδ TCR antibodies.Citation21
Treating patients with aminobisphosphonates or synthetic phosphoantigens leads to an increase in circulating Vγ9Vδ2 T cells that are able to kill autologous tumor cells in vitro.Citation22 The 3rd generation aminobisphosphonate zoledronate has been the most commonly used agent for the activation/expansion of γδ T cells in clinical trials, as it has been administered to 61/80 (72%) of patients. The EC50 of zoledronate for human γδ T-cell activation is favorable (0.003 μM)Citation5 and is well within the concentrations achievable with a standard dose of 4 mg. Zoledronate has been shown to improve the survival of multiple myeloma patients and to reduce the progression of skeletal-related events.Citation23 Zoledronate inhibits FPPS, resulting in the compensatory upregulation of non-prenylated small GTPases such as RAP1ACitation24 and the accumulation of IPP. These effects not only activate Vγ9Vδ2 T cells, they also inhibit the growth of cancer cells by suppressing protein prenylation.Citation24,Citation25 Zoledronate is rapidly cleared from the plasma following intravenous infusion, most likely due to sequestration into the bone. Following the administration of 4 mg zoledronate in cancer patients with normal renal function, mean peak plasma concentration was 1.13 µM.Citation26 A pharmacokinetic study of zoledronate infusions in patients with renal impairment showed that plasma concentrations 24 h upon infusion were < 1% of peak concentrations, but were still sufficient to elicit consistent γδ T-cell activation in vitro. The pharmacokinetics of zoledronate in children aged 3–17 are similar to those in adults when a comparable dose (mg/kg) is used (source: European Medicines Agency data, EMEA/H/C/000336 -A20/0026). Hence, at well-tolerated doses, zoledronate achieves plasma levels that are capable of activating Vγ9Vδ2 T cells.
Interleukin (IL)-2 is required to expand γδ T cells in vitro, has modest clinical activity as a standalone therapeutic agent in renal cell carcinoma (RCC) and melanoma patients,Citation27-Citation29 and has been shown to reduce the incidence of relapse among patients with hematological malignancies who underwent bone marrow transplantation.Citation30 The antineoplastic effects of IL-2 are indirect, following the activation and expansion of immune effectors. The toxicity of IL-2 at high doses is problematic, leading to hyperpyrexia, capillary leak, and hypotension. Low doses of IL-2 are well tolerated but might have undesirable effects for cancer immunotherapy. For example, while low-dose IL-2 drives T cells toward the effector memory (TEM) phenotype upon TCR stimulation, it also increases the number of circulating regulatory T cells (Treg), resulting in a robust immunosuppressive effect.Citation31 For this reason, the precise dose and schedule for IL-2 administration appears to be critical for the elicitation of optimal antitumor responses.
Search Methods
The NCBI PubMed database was queried using the MeSH terms outlined in Table S1. In addition, the bibliographies of review articles listing γδ T lymphocytes in the title and published in the last yearCitation32-Citation36 were searched for references to clinical studies.
Articles were included if the study pertained to cancer immunotherapy in humans, measured clinical outcomes and contained a clear treatment protocol that could be linked to each patient included in the study. Clinical outcome data either in the form of Response Evaluation Criteria In Solid Tumors (RECIST) assessment, progression-free survival or overall survival for each patient were also required. Full texts that only provided summarized statistics of patient data or no patient-specific information regarding cancer type or response were excluded. In these cases, the corresponding authors were contacted and asked if unpublished data were available on the patient characteristics, treatment received and clinical outcome.
Results
Patient demographics and diagnoses
Fifty-five studies were identified from the initial literature review, of which 15 were found to be suitable for screening. Three studies of 15 were excluded because of insufficient clinical data. Data were therefore available for 12 clinical studies, involving a total of 157 patients. Seventy-seven of these 157 patients had received infusions of γδ T cell-enriched populations, and 80 had received drugs to expand and activate endogenous γδ T cells. Of these, 68/77 patients subjected to adoptive γδ T cell transfer and 62/80 patients receiving γδ T cell-expanding drugs had RECIST data available. A PRISMA flow sheet of the screening process is shown in Figure S1. Patients with solid tumors were most often treated with adoptive T-cell transfer (71) as compared with γδ T cell-expanding drugs (47), whereas patients affected by hematological malignancies were more often treated with drugs (33) than with γδ T cells expanded ex vivo (6) (). The mean age of patients enrolled in adoptive T-cell transfer trials was 60 y (range 18–85 y, n = 67, 10 missing values), as opposed to 63 y (range 29–83 y, n = 58, 22 missing values) for trials testing γδ T cell-expanding drugs. There was no significant difference in the age of patients in each group.
Figure 1. Diagnosis and previous treatments of patients enrolled in clinical trials testing γδ T cell-based immunotherapy. AML, acute myeloid leukemia; CA, carcinoma; CLL, chronic lymphocytic leukemia; NSCLC, non-small cell lung carcinoma; PBMC, peripheral blood mononuclear cell; PBSCT, peripheral blood stem cell transplantation; RCC, renal cell carcinoma.
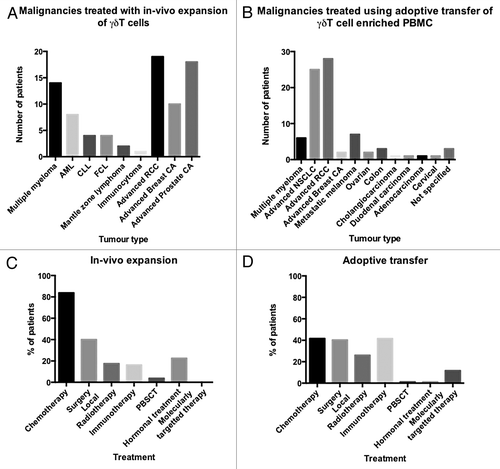
Prior therapies
As the studies reviewed were either Phase I, Phase II, or feasibility studies, participants had already received extensive treatment for their primary disease. 83.8% of patients enrolled into trials testing in vivo γδ T-cell expansion had previously received myelosuppressive chemotherapy, as compared with only 41.6% of patients allocated to γδ T-cell transfer. Conversely, 41.6% of the participants in adoptive γδ T-cell transfer trial had previously received some form of immunotherapy, as compared with only 16.3% of patients receiving γδ T cell-expanding drugs (). Prior treatments reflect the predominant diseases in each trial type. For example, a high proportion of patients previously treated with immunotherapy had melanoma or RCC.
Trials testing γδ T cell-stimulatory drugs
These trials used aminobisphosphonates +/− IL-2 to stimulate γδ T cells in vivo.Citation37-Citation41 Two studies involved standard Phase I dose-escalation protocols and 3 studies were Phase II clinical trials. In all but one case, the dose of IL-2 administered to participants within each trial was kept consistent, though there were variations between studies, as shown in .
Table 1. Treatment protocols aimed at expanding γδ T cells in vivo using zoledronate and IL-2
In an intra-patient dose-escalation study by Wilhelm and coworkers,Citation38 gradually increasing doses of IL-2 were administered to 19 patients with hematological malignancies to determine the effects of IL-2 dose and administration route. Following disappointing results in patients receiving continuous subcutaneous infusions on day (D)3–8 of each treatment cycle, the protocol was altered to 6 h intravenous bolus infusions on D1–6. It is not possible to compare the overall efficacy of these IL-2 delivery techniques from this study as patients were enrolled in the cohort treated under the altered protocol only if they had a significant expansion of γδ T cells in vitro (> 20% proliferation at D8 of culture with pamidronate and IL-2) (Table S1).
Adoptive transfer of enriched γδ T-cell populations
Clinical trials testing the adoptive transfer of γδ T cells were more homogenous in their protocol design than those investigating γδ T cell- stimulatory drugs. Indeed, the former mainly varied relative to the stimulus used to expand γδ T cells ex vivo, and the number and timing of the γδ T-cell infusions. Adoptive transfer protocols involve obtaining lymphocytes from the patient and then culturing them in conditions that selectively promote γδ T-cell proliferation. After a period of proliferation (usually 14 d), γδ T cells are re-infused into the patient, along with further immunostimulatory agents in some cases. Ex vivo expansion requires specialized laboratories and expertise in handling cellular therapy products.
The majority (78%) of patients enrolled in adoptive transfer studies had solid tumors. The major variable across protocols lies in ex vivo expansion methods. Additional variables include the cell source (leukopheresis, n = 2, or the peripheral blood, n = 5), and the length of time between subsequent γδ T-cell infusions. In addition, 2 studies administered IL-2, 1 zoledronate and 1 both agents alongside adoptively transferred cellsCitation6,Citation42-Citation44 (). Expansion protocols varied substantially, and in some studies they involved a very high concentration of IL-2 (1000 U/mL).Citation45-Citation47 The expansion of γδ T cells from the peripheral blood is clearly feasible, while studies involving leukopheresis did not achieve significantly higher numbers of γδ T cells than those based on γδ T-cell expansion from the whole blood (leukopheresis, mean 11.3 x 109 cells, 95% CI 5.8–16.9 x 109 cells: whole blood, mean 16.2 × 109 cells; 95% CI 12.5–19.9 × 109 cells). This suggests that leukopheresis is not required to generate satisfactory γδ T-cell products.
Table 2. Comparison of clinical trials using adoptively transferred γδ T cells
Clinical responses to γδ T-cell immunotherapy as compared with conventional second-line treatments
To compare the clinical response to γδ T-cell immunotherapy with standard-of-care second-line treatment approaches, we selected 3 cancer types for which national guidelines for second-line treatment exist in the UK (from the National Institute for Clinical Excellence, NICE) or US (from the National Comprehensive Cancer Network, NCCN), namely, renal cell carcinoma (RCC), non-small cell lung carcinoma (NSCLC), and prostate cancer. Outcomes in terms of clinical responses were compared. These 3 cancers also represented the commonest types of tumor in patients enrolled in γδ T-cell immunotherapy trials.
The only second-line regimen currently recommended by the NICE for the treatment of refractory/relapsed advanced prostate cancer is the combination of docetaxel and prednisolone.Citation48 Disease outcome data regarding this combination is available from numerous sources.Citation49-Citation51 There is currently no NICE recommended second-line treatment for advanced/metastatic RCC, but the NCCN recommends everolimus, much of the evidence in support of this option coming from the RECORD-1 trial.Citation52 Docetaxel or erlotinib are recommended for second-line chemotherapy in patients with Stage III-IV NSCLC.Citation53 A recent comparator studyCitation54 demonstrated a slight superiority for erlotinib over docetaxel for the second-line treatment of advanced NSCLC. Both these therapeutic options are permissible under current NICE guidelines.
Data on disease outcome from large studies that were used in the formulation of the treatment guidelines for these 3 tumor types are shown in , alongside comparisons with disease outcome from corresponding γδ T-cell immunotherapy trials. A more extensive breakdown of the results from γδ T-cell immunotherapy trials is shown in Table S2. Although direct statistical comparisons are not possible, the proportion of objective responses among patients enrolled in clinical trials testing γδ T cell-based immunotherapy is superior to that achieved with established second-line therapy in patients with advanced prostate cancer (33.3% with γδ T cells vs. 25.2% with prednisolone + docetaxel) and advanced RCC (4.8% with γδ T cells vs. 1.8% with everolimus), but not advanced NSCLC patients (7.6% with erlotinib, 12.2% with docetaxel, 0% with γδ T cells). While this could be explained through patient selection, all individuals analyzed had relapsed or recurrent disease and so are broadly comparable in terms of prognosis.
Table 3. Clinical outcomes of commonly used second-line anticancer agents as compared with γδ T-cell immunotherapy.*
Variation in γδ T-cell expansion capacity between patients
Tumor immune evasion can be facilitated by host cells. Regulatory T cells (Tregs) are an important immunosuppressive cell population that prevent autoimmune responses and excessive reactions against self entities. Tumors that recruit high levels of CD4+CD25+FOXP3+ Tregs among tumor-infiltrating lymphocytes (TILs) are associated with invasive disease.Citation55-Citation57 γδ T cells from healthy individuals and cancer patients can be expanded to clinically useful numbers, even if patients have previously received chemotherapy,Citation19,Citation58 but there is a high degree of inter-individual variation in expansion capacity. In one study, γδ T cells from 88% (14/16) healthy donors expanded in vitro in response to IL-2 + pamidronate, whereas γδ T cells from only 49% (20/41) cancer patients (including multiple myeloma, MM, non-Hodgkin’s lymphoma, NHL and, B-cell chronic lymphocytic leukemia (B-CLL) expanded following the same stimuli.Citation38 There is an inverse correlation between the frequency of circulating Tregs and the ability of γδ T cells from cancer patients to proliferate upon phosphoantigen stimulation in vitro. However, Tregs do not directly suppress the cytotoxic activity of γδ T cells or their ability to produce cytokines,Citation59 a factor that is in favor of the adoptive transfer of γδ T cells over the use of γδ T cell-stimulatory agents, as the cells can be expanded in optimized conditions.
The variability in γδ T-cell proliferation was documented in a number of trials in which patients were screened before enrolment to determine whether their γδ T cells would expand in vitro. Thirty-five out of 77 patients treated with adoptively transferred γδ T cells and 31/80 patients treated with γδ T cell-stimulating drugs were stratified based on in vitro γδ T-cell expansion rate in response to the same stimulus used in the trial. While the overall response of these “positive responders” is better than that of unscreened patients receiving γδ T cell-stimulating drugs (overall response rate 16.2% vs. 8.1%) these populations are too small and heterogeneous for firm conclusions (Table S3).
Antibody-dependent γδ T cell-mediated cytotoxicity
The initial evaluation of γδ T cell-based immunotherapy shows some promise but there is large room for improvement. Overall, conventional response rates are poor, with only 14/130 (10.8%) objective responses documented across all of the trials assessed. However, 51 (39.2%) patients achieved disease stabilization, a successful outcome of immunotherapy, indicating that clinical benefits can be achieved by a high proportion of patients subjected to γδ T cell-based immunotherapy. The capacity of Vγ9Vδ2 γδ T cells to kill malignant cells in vitro is well documented, and means of augmenting the cytotoxic activity of γδ T cells should be investigated. A number of studies have demonstrated the potential benefit of combining γδ T cells with therapeutic tumor-targeting antibodies.Citation32,Citation34 To evaluate the evidence in support of this notion we reviewed papers that reported the cytotoxicity of γδ T cells in vitro and in vivo, in the presence or in the absence of tumor-targeting antibodies. The addition of an appropriate tumor-targeting antibody improved the cytotoxic activity of γδ T cells in 132 separate experiments, reported in 6 different publications, based on 12 different cell lines or 5 primary tumor tissues, providing a statistically significant improvement in 94/132 (71.2%) experimental settings.Citation3,Citation4,Citation15-Citation18 These effect was observed in both hematological and solid tumor models, including CD20+ hematological malignancies, CD52+ lymphomas and v-erb-b2 avian erythroblastic leukemia viral oncogene homolog 2 (ERBB2)+ breast cancers. The addition of the anti-CD20 antibody rituximab significantly prolonged the clearance of malignant B cells from the circulation of cynomolgus macaques when combined with the γδ T cell-stimulator BrHPP.Citation3 In a mouse model of ERBB2+ breast carcinoma, the addition of the anti-ERBB2 antibody trastuzumab (Herceptin®) significantly increased tumor infiltration by Vγ9+ γδ T cells. In this study, mice treated with adoptively transferred γδ T cells and trastuzumab achieved a superior control of tumor growth, as compared with animals receiving γδ T cells alone, trastuzumab alone or vehicle.Citation17
Discussion
γδ T cells are a potential alternative to αβ T cells for cellular immunotherapy. They have a number of advantages that could be exploited, not least the fact that they can be easily expanded in vivo upon the administration drugs with well established safety records in adults and children. The sequential nature of the lymphoid immune response is governed by the time required to expand sufficient effector numbers to generate antimicrobial or antitumor reactivity.Citation60 The activation of γδ T cells in response to a range of stress signals such as NKG2D ligands, endogenous phosphoantigens, or TLR agonists is independent of HLA molecules. The kinetics of the γδ T-cell response in vivo is faster than that of the αβ T-cell response, as the former requires neither priming by dendritic cells in lymph nodes, nor clonal expansion. In an immunodeficient mouse model, adoptively transferred human Vγ9Vδ2 cells mounted almost immediate anti-bacterial responses following administration.Citation61 γδ T cells also acquire professional antigen-presenting function upon activation, implying that they may have a value as cellular vaccines that goes beyond their ability to exert cytotoxic functions.Citation2,Citation4,Citation62
Adoptive transfer of T cells
The possibility to enhance antitumor immune responses using tumor-specific αβ T cells expanded ex vivo was first demonstrated in melanomaCitation63 and RCC patients,Citation64 from whom tumor-infiltrating lymphocytes (TILs) can be readily obtained. The isolation of TILs has indeed proved problematic in patients affected by most other tumor types, and no data are available on tumor-infiltrating γδ T cells. Without prior lymphodepletion, adoptively transferred TILs are short-lived and clinical benefits are transient. Lymphodepletion significantly improves the clinical benefit of this immunotherapeutic regimen. In a cohort of melanoma patients, lymphodepletion followed by adoptive T-cell transfer resulted in a response rate of 56% and many patients still remain disease-free at follow up (4–10 y).Citation65 Interestingly, autologous γδ T cells expanded ex vivo have been shown to persist in the circulation of cancer patients receiving IL-2 but no prior lymphodepletion for over 12 wk.Citation45,Citation66
TILs are unavailable for a majority of patients affected tumors other than melanoma and RCC, implying that T cells must be expanded or engineered ex vivo to generate a bulk population of tumor-reactive cells for adoptive transfer. The efficacy of adoptively transferred tumor-reactive αβ T cells can decrease upon the loss of antigen expression by malignant cells, which occurs frequently in response to the selective pressure of therapy itself. γδ T cells, which recognize a broad range of stress signals emitted by malignant cells, are not subjected to this limitation. Moreover, as the cytotoxic potential of γδ T cells is independent of HLA molecules, limits the need for engineering in this sense. Nonetheless, the adoptive transfer of γδ T cells could be combined with T-cell engineering to enhance functions other than cytotoxicity.
γδ T cells as vaccines
A further advantage of γδ T cells over αβ T cells is that the former acquire professional antigen-presenting capacity upon stimulation, expressing increased levels of co-stimulatory molecules such as CD80 and CD86, as well as of molecules associated with the homing to lymph nodes.Citation2-Citation4,Citation62,Citation67. γδ T cells also share some properties with NK cells and cytokine-induced killer (CIK) cells, such as the innate cytotoxic potential and the ability to mediate antibody-dependent cell-mediated cytotoxicity. The antigen-presenting capacity of dendritic cells has already been harnessed in clinical trials that have been running for over ten years.Citation68 Adoptively transferred NK cells showed efficacy in metastatic RCC,Citation69 breast cancer,Citation70 and malignant glioma patients.Citation71 The combination of innate cytotoxic and antigen-presenting functions raises the intriguing possibility that γδ T cells could be used as a cellular vaccine that would kill malignant cells in situ and cross-present tumor-associated antigens to αβ T cells, hence generating a potent and long-lasting immune response. γδ T cells can be expanded in vitro and in vivo and their role as antigen-presenting cells, alone or combined with antibodies that enhance their effector functions, can be evaluated in clinical trials.
Overcoming inhibitory signals
The immunosuppressive nature of the tumor microenvironment is one of the biggest obstacles against successful immunotherapy. Strategies for unpicking these barriers are continuously progressing, the discovery that blocking the PD-1/PD-L1 interaction significantly reduces immune evasion and provides objective clinical benefits perhaps being the most recent example.Citation72 Inhibiting the immunosuppressive activity of CTLA4 with the anti-CTLA4 antibody ipilumumab is also highly effective in metastatic melanoma patients,Citation73 and is likely to provide clinical benefits to patients affected by other malignancies, such as prostate cancer.Citation74 In line with their central role in innate immunity, the activation of γδ T cells is controlled by a balance of activatory and inhibitory signals.Citation10 Tumors are known to produce several mediators that inhibit γδ T and NK-cell functions including soluble NKG2D ligands as well as TGFβ1 and PGE2.Citation10,Citation13,Citation75 However, compelling in vitro and in vivo evidence indicate that γδ T cells and antitumor antibodies can be successfully combined for the treatment of both hematological and solid malignancies,Citation3,Citation4,Citation15-Citation18 indicating that tumor-elicited immunosuppression can be overcome. The combination of γδ T cell-expanding agents and tumor-targeting antibodies could tip a failing immune response dominated by inhibitory cells such as Tregs, myeloid-derived suppressor cells, and inhibitory/tolerizing dendritic cellsCitation76 and the activation of immune checkpoints mediated by CTLA4 and the B7 protein family,Citation77 toward a robust cytotoxic T-cell response. Although malignant cells accumulate higher amounts of phosphoantigens than healthy cells,Citation1 an effect that is magnified by the administration of aminobisphosphonates,Citation78 this appears to be insufficient to fully overcome tumor-elicited immunosuppression. While TIL-derived γδ T cells derived will selectively kill transformed cells and spare healthy bystanders,Citation79 tumor-targeting antibodies may be required for achieving optimal cytotoxic γδ T-cell responses. Thus, combining γδ T cell-based immunotherapy with tumor-specific antibodies might spare healthy cells expressing tumor-associated antigens if the engagement of the γδ TCR is also required for optimal effector functions. Moreover, this approach might result in full-blown activation of professional antigen-presenting cells at the tumor site. The combination of γδ T cells with immunomodulatory and/or cytolytic antibodies is an attractive prospect for future clinical trials.
Conclusions
Successful immunotherapy relies on the control of the balance between antitumor cytotoxicity and immunological tolerance. In this context, adoptively transferred αβ T-cell populations potently attack specific targets but are limited by their specificity, dendritic cells lack cytotoxic functions of their own and NK cells have given inconsistent results in clinical trials.Citation69-Citation71 γδ T cells in combination with tumor-targeting antibodies might provide a direct but not antigen-exclusive response, potentially mediating not only antitumor cytotoxic effects but also long-lasting protection upon antigen presentation. Results from Phase I and II clinical trials indicate that the efficacy of γδ T cell-based immunotherapy is comparable to that of conventional second-line therapies. Combining agents that promote γδ T-cell expansion and activation with cytolytic tumor-specific antibodies is a feasible and logical approach with an (expectedly) favorable toxicity profile.
Abbreviations: | ||
BrHPP | = | bromohydrin pyrophosphate |
Additional material
Download Zip (166 KB)Acknowledgments
The authors would like to acknowledge Dr Darren Hargrave for his input regarding comparison of phase I and II trials. Dr Fisher is funded by the Wellcome Trust, Sparks and the Dubois Child Cancer Fund. John Anderson holds a Great Ormond Street Hospital Charity Leadership award. MY is funded by a project grant from Leukaemia Lymphoma Research (UK).
Supplemental materials may be found here:
http://www.landesbioscience.com/journals/oncoimmunology/article/27572/
Citation: Fisher J, Heuijerjens J, Yan M, Gustafsson K, Anderson J. γδT cells for cancer immunotherapy - a systematic review of clinical trials. OncoImmunology 2013; 2:e27572; 10.4161/onci.27572
References
- Gober HJ, Kistowska M, Angman L, Jenö P, Mori L, De Libero G. Human T cell receptor gammadelta cells recognize endogenous mevalonate metabolites in tumor cells. J Exp Med 2003; 197:163 - 8; http://dx.doi.org/10.1084/jem.20021500; PMID: 12538656
- Brandes M, Willimann K, Moser B. Professional antigen-presentation function by human gammadelta T Cells. Science 2005; 309:264 - 8; http://dx.doi.org/10.1126/science.1110267; PMID: 15933162
- Gertner-Dardenne J, Bonnafous C, Bezombes C, Capietto AH, Scaglione V, Ingoure S, Cendron D, Gross E, Lepage JF, Quillet-Mary A, et al. Bromohydrin pyrophosphate enhances antibody-dependent cell-mediated cytotoxicity induced by therapeutic antibodies. Blood 2009; 113:4875 - 84; http://dx.doi.org/10.1182/blood-2008-08-172296; PMID: 19278954
- Himoudi N, Morgenstern DA, Yan M, Vernay B, Saraiva L, Wu Y, Cohen CJ, Gustafsson K, Anderson J. Human γδ T lymphocytes are licensed for professional antigen presentation by interaction with opsonized target cells. J Immunol 2012; 188:1708 - 16; http://dx.doi.org/10.4049/jimmunol.1102654; PMID: 22250090
- Belmant C, Decise D, Fournié J-J. Phosphoantigens and aminobisphosphonates: New leads targeting γδ T lymphocytes for cancer immunotherapy. Drug Discov Today Ther Strateg 2006; 3:17 - 23; http://dx.doi.org/10.1016/j.ddstr.2006.02.001
- Bennouna J, Bompas E, Neidhardt EM, Rolland F, Philip I, Galéa C, Salot S, Saïagh S, Audrain M, Rimbert M, et al. Phase-I study of Innacell gammadelta, an autologous cell-therapy product highly enriched in gamma9delta2 T lymphocytes, in combination with IL-2, in patients with metastatic renal cell carcinoma. Cancer Immunol Immunother 2008; 57:1599 - 609; http://dx.doi.org/10.1007/s00262-008-0491-8; PMID: 18301889
- Bennouna J, Levy V, Sicard H, Senellart H, Audrain M, Hiret S, Rolland F, Bruzzoni-Giovanelli H, Rimbert M, Galéa C, et al. Phase I study of bromohydrin pyrophosphate (BrHPP, IPH 1101), a Vgamma9Vdelta2 T lymphocyte agonist in patients with solid tumors. Cancer Immunol Immunother 2010; 59:1521 - 30; http://dx.doi.org/10.1007/s00262-010-0879-0; PMID: 20563721
- D’Ombrain MC, Hansen DS, Simpson KM, Schofield L. gammadelta-T cells expressing NK receptors predominate over NK cells and conventional T cells in the innate IFN-γ response to Plasmodium falciparum malaria. Eur J Immunol 2007; 37:1864 - 73; http://dx.doi.org/10.1002/eji.200636889; PMID: 17557374
- Hermes M, Albrecht C, Schrod A, Brameier M, Walter L. Expression patterns of killer cell immunoglobulin-like receptors (KIR) of NK-cell and T-cell subsets in Old World monkeys. PLoS One 2013; 8:e64936; http://dx.doi.org/10.1371/journal.pone.0064936; PMID: 23717676
- Martinet L, Poupot R, Fournié J-J. Pitfalls on the roadmap to gammadelta T cell-based cancer immunotherapies. Immunol Lett 2009; 124:1 - 8; http://dx.doi.org/10.1016/j.imlet.2009.03.011; PMID: 19465238
- Raffaghello L, Marimpietri D, Pagnan G, Pastorino F, Cosimo E, Brignole C, Ponzoni M, Montaldo PG. Anti-GD2 monoclonal antibody immunotherapy: a promising strategy in the prevention of neuroblastoma relapse. Cancer Lett 2003; 197:205 - 9; http://dx.doi.org/10.1016/S0304-3835(03)00100-9; PMID: 12880983
- Park YP, Choi S-C, Kiesler P, Gil-Krzewska A, Borrego F, Weck J, Krzewski K, Coligan JE. Complex regulation of human NKG2D-DAP10 cell surface expression: opposing roles of the γc cytokines and TGF-β1. Blood 2011; 118:3019 - 27; http://dx.doi.org/10.1182/blood-2011-04-346825; PMID: 21816829
- Raffaghello L, Prigione I, Airoldi I, Camoriano M, Morandi F, Bocca P, Gambini C, Ferrone S, Pistoia V. Mechanisms of immune evasion of human neuroblastoma. Cancer Lett 2005; 228:155 - 61; http://dx.doi.org/10.1016/j.canlet.2004.11.064; PMID: 15923080
- Kong Y, Cao W, Xi X, Ma C, Cui L, He W. The NKG2D ligand ULBP4 binds to TCR γ 9/δ2 and induces cytotoxicity to tumor cells through both TCR and NKG2D. Blood 2009; 114:310 - 7; http://dx.doi.org/10.1182/blood-2008-12-196287; PMID: 19436053
- Tokuyama H, Hagi T, Mattarollo SR, Morley J, Wang Q, So HF, Moriyasu F, Nieda M, Nicol AJ. V γ 9 V δ 2 T cell cytotoxicity against tumor cells is enhanced by monoclonal antibody drugs--rituximab and trastuzumab. Int J Cancer 2008; 122:2526 - 34; http://dx.doi.org/10.1002/ijc.23365; PMID: 18307255
- Braza MS, Klein B, Fiol G, Rossi JF. γδ T-cell killing of primary follicular lymphoma cells is dramatically potentiated by GA101, a type II glycoengineered anti-CD20 monoclonal antibody. Haematologica 2011; 96:400 - 7; http://dx.doi.org/10.3324/haematol.2010.029520; PMID: 21109686
- Capietto AH, Martinet L, Fournié JJ. Stimulated γδ T cells increase the in vivo efficacy of trastuzumab in HER-2+ breast cancer. J Immunol 2011; 187:1031 - 8; http://dx.doi.org/10.4049/jimmunol.1100681; PMID: 21670311
- Chen Z, Freedman MS. CD16+ gammadelta T cells mediate antibody dependent cellular cytotoxicity: potential mechanism in the pathogenesis of multiple sclerosis. Clin Immunol 2008; 128:219 - 27; http://dx.doi.org/10.1016/j.clim.2008.03.513; PMID: 18501678
- Chargui J, Combaret V, Scaglione V, Iacono I, Péri V, Valteau-Couanet D, Dubrel M, Angevin E, Puisieux A, Romagné F, et al. Bromohydrin pyrophosphate-stimulated Vgamma9delta2 T cells expanded ex vivo from patients with poor-prognosis neuroblastoma lyse autologous primary tumor cells. J Immunother 2010; 33:591 - 8; http://dx.doi.org/10.1097/CJI.0b013e3181dda207; PMID: 20551838
- Kondo M, Sakuta K, Noguchi A, Ariyoshi N, Sato K, Sato S, Sato K, Hosoi A, Nakajima J, Yoshida Y, et al. Zoledronate facilitates large-scale ex vivo expansion of functional gammadelta T cells from cancer patients for use in adoptive immunotherapy. Cytotherapy 2008; 10:842 - 56; http://dx.doi.org/10.1080/14653240802419328; PMID: 19016372
- Zhou J, Kang N, Cui L, Ba D, He W. Anti-γδ TCR antibody-expanded γδ T cells: a better choice for the adoptive immunotherapy of lymphoid malignancies. Cell Mol Immunol 2012; 9:34 - 44; http://dx.doi.org/10.1038/cmi.2011.16; PMID: 21666706
- Kunzmann V, Bauer E, Feurle J, Weissinger F, Tony HP, Wilhelm M. Stimulation of gammadelta T cells by aminobisphosphonates and induction of antiplasma cell activity in multiple myeloma. Blood 2000; 96:384 - 92; PMID: 10887096
- Morgan GJ, Davies FE, Gregory WM, Cocks K, Bell SE, Szubert AJ, Navarro-Coy N, Drayson MT, Owen RG, Feyler S, et al, National Cancer Research Institute Haematological Oncology Clinical Study Group. First-line treatment with zoledronic acid as compared with clodronic acid in multiple myeloma (MRC Myeloma IX): a randomised controlled trial. Lancet 2010; 376:1989 - 99; http://dx.doi.org/10.1016/S0140-6736(10)62051-X; PMID: 21131037
- Roelofs AJ, Thompson K, Gordon S, Rogers MJ. Molecular mechanisms of action of bisphosphonates: current status. Clin Cancer Res 2006; 12:6222s - 30s; http://dx.doi.org/10.1158/1078-0432.CCR-06-0843; PMID: 17062705
- Guenther A, Gordon S, Tiemann M, Burger R, Bakker F, Green JR, Baum W, Roelofs AJ, Rogers MJ, Gramatzki M. The bisphosphonate zoledronic acid has antimyeloma activity in vivo by inhibition of protein prenylation. Int J Cancer 2010; 126:239 - 46; http://dx.doi.org/10.1002/ijc.24758; PMID: 19621390
- Skerjanec A, Berenson J, Hsu C, Major P, Miller WH Jr., Ravera C, Schran H, Seaman J, Waldmeier F. The pharmacokinetics and pharmacodynamics of zoledronic acid in cancer patients with varying degrees of renal function. J Clin Pharmacol 2003; 43:154 - 62; http://dx.doi.org/10.1177/0091270002239824; PMID: 12616668
- Fyfe G, Fisher RI, Rosenberg SA, Sznol M, Parkinson DR, Louie AC. Results of treatment of 255 patients with metastatic renal cell carcinoma who received high-dose recombinant interleukin-2 therapy. J Clin Oncol 1995; 13:688 - 96; PMID: 7884429
- Atkins MB, Lotze MT, Dutcher JP, Fisher RI, Weiss G, Margolin K, Abrams J, Sznol M, Parkinson D, Hawkins M, et al. High-dose recombinant interleukin 2 therapy for patients with metastatic melanoma: analysis of 270 patients treated between 1985 and 1993. J Clin Oncol 1999; 17:2105 - 16; PMID: 10561265
- Figlin RA. Renal cell carcinoma: current status and future plans. Cancer J Sci Am 2000; 6:Suppl 1 S52 - 4; PMID: 10685659
- Soiffer RJ, Murray C, Gonin R, Ritz J. Effect of low-dose interleukin-2 on disease relapse after T-cell-depleted allogeneic bone marrow transplantation. Blood 1994; 84:964 - 71; PMID: 8043878
- Koreth J, Matsuoka K, Kim HT, McDonough SM, Bindra B, Alyea EP 3rd, Armand P, Cutler C, Ho VT, Treister NS, et al. Interleukin-2 and regulatory T cells in graft-versus-host disease. N Engl J Med 2011; 365:2055 - 66; http://dx.doi.org/10.1056/NEJMoa1108188; PMID: 22129252
- Fournié J-J, Sicard H, Poupot M, Bezombes C, Blanc A, Romagné F, Ysebaert L, Laurent G. What lessons can be learned from γδ T cell-based cancer immunotherapy trials?. Cell Mol Immunol 2013; 10:35 - 41; http://dx.doi.org/10.1038/cmi.2012.39; PMID: 23241899
- Vantourout P, Hayday A. Six-of-the-best: unique contributions of γδ T cells to immunology. Nat Rev Immunol 2013; 10:35 - 41; PMID: 23348415
- Braza MS, Klein B. Anti-tumour immunotherapy with Vγ9Vδ2 T lymphocytes: from the bench to the bedside. Br J Haematol 2013; 160:123 - 32; http://dx.doi.org/10.1111/bjh.12090; PMID: 23061882
- Li Z. Potential of human γδ T cells for immunotherapy of osteosarcoma. Mol Biol Rep 2013; 40:427 - 37; http://dx.doi.org/10.1007/s11033-012-2077-y; PMID: 23065272
- Riganti C, Massaia M, Davey MS, Eberl M. Human γδ T-cell responses in infection and immunotherapy: common mechanisms, common mediators?. Eur J Immunol 2012; 42:1668 - 76; http://dx.doi.org/10.1002/eji.201242492; PMID: 22806069
- Kunzmann V, Smetak M, Kimmel B, Weigang-Koehler K, Goebeler M, Birkmann J, Becker J, Schmidt-Wolf IGH, Einsele H, Wilhelm M. Tumor-promoting versus tumor-antagonizing roles of γδ T cells in cancer immunotherapy: results from a prospective phase I/II trial. J Immunother 2012; 35:205 - 13; http://dx.doi.org/10.1097/CJI.0b013e318245bb1e; PMID: 22306909
- Wilhelm M, Kunzmann V, Eckstein S, Reimer P, Weissinger F, Ruediger T, Tony HP. Gammadelta T cells for immune therapy of patients with lymphoid malignancies. Blood 2003; 102:200 - 6; http://dx.doi.org/10.1182/blood-2002-12-3665; PMID: 12623838
- Lang JM, Kaikobad MR, Wallace M, Staab MJ, Horvath DL, Wilding G, Liu G, Eickhoff JC, McNeel DG, Malkovsky M. Pilot trial of interleukin-2 and zoledronic acid to augment γδ T cells as treatment for patients with refractory renal cell carcinoma. Cancer Immunol Immunother 2011; 60:1447 - 60; http://dx.doi.org/10.1007/s00262-011-1049-8; PMID: 21647691
- Dieli F, Vermijlen D, Fulfaro F, Caccamo N, Meraviglia S, Cicero G, Roberts A, Buccheri S, D’Asaro M, Gebbia N, et al. Targeting human gammadelta T cells with zoledronate and interleukin-2 for immunotherapy of hormone-refractory prostate cancer. Cancer Res 2007; 67:7450 - 7; http://dx.doi.org/10.1158/0008-5472.CAN-07-0199; PMID: 17671215
- Meraviglia S, Eberl M, Vermijlen D, Todaro M, Buccheri S, Cicero G, La Mendola C, Guggino G, D’Asaro M, Orlando V, et al. In vivo manipulation of Vgamma9Vdelta2 T cells with zoledronate and low-dose interleukin-2 for immunotherapy of advanced breast cancer patients. Clin Exp Immunol 2010; 161:290 - 7; PMID: 20491785
- Kobayashi H, Tanaka Y, Yagi J, Minato N, Tanabe K. Phase I/II study of adoptive transfer of γδ T cells in combination with zoledronic acid and IL-2 to patients with advanced renal cell carcinoma. Cancer Immunol Immunother 2011; 60:1075 - 84; http://dx.doi.org/10.1007/s00262-011-1021-7; PMID: 21519826
- Kobayashi H, Tanaka Y, Yagi J, Osaka Y, Nakazawa H, Uchiyama T, Minato N, Toma H. Safety profile and anti-tumor effects of adoptive immunotherapy using gamma-delta T cells against advanced renal cell carcinoma: a pilot study. Cancer Immunol Immunother 2007; 56:469 - 76; http://dx.doi.org/10.1007/s00262-006-0199-6; PMID: 16850345
- Nicol AJ, Tokuyama H, Mattarollo SR, Hagi T, Suzuki K, Yokokawa K, Nieda M. Clinical evaluation of autologous gamma delta T cell-based immunotherapy for metastatic solid tumours. Br J Cancer 2011; 105:778 - 86; http://dx.doi.org/10.1038/bjc.2011.293; PMID: 21847128
- Nakajima J, Murakawa T, Fukami T, Goto S, Kaneko T, Yoshida Y, Takamoto S, Kakimi K. A phase I study of adoptive immunotherapy for recurrent non-small-cell lung cancer patients with autologous gammadelta T cells. Eur J Cardiothorac Surg 2010; 37:1191 - 7; http://dx.doi.org/10.1016/j.ejcts.2009.11.051; PMID: 20137969
- Abe Y, Muto M, Nieda M, Nakagawa Y, Nicol A, Kaneko T, Goto S, Yokokawa K, Suzuki K. Clinical and immunological evaluation of zoledronate-activated Vgamma9gammadelta T-cell-based immunotherapy for patients with multiple myeloma. Exp Hematol 2009; 37:956 - 68; http://dx.doi.org/10.1016/j.exphem.2009.04.008; PMID: 19409955
- Sakamoto M, Nakajima J, Murakawa T, Fukami T, Yoshida Y, Murayama T, Takamoto S, Matsushita H, Kakimi K. Adoptive immunotherapy for advanced non-small cell lung cancer using zoledronate-expanded γδTcells: a phase I clinical study. J Immunother 2011; 34:202 - 11; http://dx.doi.org/10.1097/CJI.0b013e318207ecfb; PMID: 21304399
- Graham J, Baker M, Macbeth F, Titshall V, Guideline Development Group. Diagnosis and treatment of prostate cancer: summary of NICE guidance. BMJ 2008; 336:610 - 2; http://dx.doi.org/10.1136/bmj.39498.525706.AD; PMID: 18340076
- Naito S, Tsukamoto T, Koga H, Harabayashi T, Sumiyoshi Y, Hoshi S, Akaza H. Docetaxel plus prednisolone for the treatment of metastatic hormone-refractory prostate cancer: a multicenter Phase II trial in Japan. Jpn J Clin Oncol 2008; 38:365 - 72; http://dx.doi.org/10.1093/jjco/hyn029; PMID: 18417502
- Machiels J-P, Mazzeo F, Clausse M, Filleul B, Marcelis L, Honhon B, D’Hondt L, Dopchie C, Verschaeve V, Duck L, et al. Prospective randomized study comparing docetaxel, estramustine, and prednisone with docetaxel and prednisone in metastatic hormone-refractory prostate cancer. J Clin Oncol 2008; 26:5261 - 8; http://dx.doi.org/10.1200/JCO.2008.16.9524; PMID: 18794543
- Cicero G, De Luca R. Docetaxel plus prednisone in patients with metastatic hormone-refractory prostate cancer: an Italian clinical experience. Eur Rev Med Pharmacol Sci 2011; 15:325 - 31; PMID: 21528780
- Motzer RJ, Escudier B, Oudard S, Hutson TE, Porta C, Bracarda S, Grünwald V, Thompson JA, Figlin RA, Hollaender N, et al, RECORD‐1 Study Group. Phase 3 trial of everolimus for metastatic renal cell carcinoma : final results and analysis of prognostic factors. Cancer 2010; 116:4256 - 65; http://dx.doi.org/10.1002/cncr.25219; PMID: 20549832
- Heigener DFD, Wu Y-LY, van Zandwijk N, Mali P, Horwood K, Reck M. Second-line erlotinib in patients with advanced non-small-cell lung cancer: subgroup analyses from the TRUST study. Lung Cancer 2011; 74:274 - 9; http://dx.doi.org/10.1016/j.lungcan.2011.02.017; PMID: 21439671
- Garassino MC, Martelli O, Broggini M, Farina G, Veronese S, Rulli E, Bianchi F, Bettini A, Longo F, Moscetti L, et al, TAILOR trialists. Erlotinib versus docetaxel as second-line treatment of patients with advanced non-small-cell lung cancer and wild-type EGFR tumours (TAILOR): a randomised controlled trial. Lancet Oncol 2013; 14:981 - 8; http://dx.doi.org/10.1016/S1470-2045(13)70310-3; PMID: 23883922
- Curiel TJ, Coukos G, Zou L, Alvarez X, Cheng P, Mottram P, Evdemon-Hogan M, Conejo-Garcia JR, Zhang L, Burow M, et al. Specific recruitment of regulatory T cells in ovarian carcinoma fosters immune privilege and predicts reduced survival. Nat Med 2004; 10:942 - 9; http://dx.doi.org/10.1038/nm1093; PMID: 15322536
- Curiel TJ. Tregs and rethinking cancer immunotherapy. J Clin Invest 2007; 117:1167 - 74; http://dx.doi.org/10.1172/JCI31202; PMID: 17476346
- Gao Q, Qiu SJ, Fan J, Zhou J, Wang XY, Xiao YS, Xu Y, Li YW, Tang ZY. Intratumoral balance of regulatory and cytotoxic T cells is associated with prognosis of hepatocellular carcinoma after resection. J Clin Oncol 2007; 25:2586 - 93; http://dx.doi.org/10.1200/JCO.2006.09.4565; PMID: 17577038
- Burjanadzé M, Condomines M, Reme T, Quittet P, Latry P, Lugagne C, Romagné F, Morel Y, Rossi JF, Klein B, et al. In vitro expansion of gamma delta T cells with anti-myeloma cell activity by Phosphostim and IL-2 in patients with multiple myeloma. Br J Haematol 2007; 139:206 - 16; http://dx.doi.org/10.1111/j.1365-2141.2007.06754.x; PMID: 17897296
- Kunzmann V, Kimmel B, Herrmann T, Einsele H, Wilhelm M. Inhibition of phosphoantigen-mediated gammadelta T-cell proliferation by CD4+ CD25+ FoxP3+ regulatory T cells. Immunology 2009; 126:256 - 67; http://dx.doi.org/10.1111/j.1365-2567.2008.02894.x; PMID: 18775028
- Hayday AC. Gammadelta T cells and the lymphoid stress-surveillance response. Immunity 2009; 31:184 - 96; http://dx.doi.org/10.1016/j.immuni.2009.08.006; PMID: 19699170
- Wang L, Kamath A, Das H, Li L, Bukowski JF. Antibacterial effect of human V γ 2V δ 2 T cells in vivo. J Clin Invest 2001; 108:1349 - 57; PMID: 11696580
- Wu Y, Wu W, Wong WM, Ward E, Thrasher AJ, Goldblatt D, Osman M, Digard P, Canaday DH, Gustafsson K. Human γ δ T cells: a lymphoid lineage cell capable of professional phagocytosis. J Immunol 2009; 183:5622 - 9; http://dx.doi.org/10.4049/jimmunol.0901772; PMID: 19843947
- Rosenberg SA, Packard BS, Aebersold PM, Solomon D, Topalian SL, Toy ST, Simon P, Lotze MT, Yang JC, Seipp CA, et al. Use of tumor-infiltrating lymphocytes and interleukin-2 in the immunotherapy of patients with metastatic melanoma. A preliminary report. N Engl J Med 1988; 319:1676 - 80; http://dx.doi.org/10.1056/NEJM198812223192527; PMID: 3264384
- Tang X, Liu T, Zang X, Liu H, Wang D, Chen H, Zhang B. Adoptive cellular immunotherapy in metastatic renal cell carcinoma: a systematic review and meta-analysis. PLoS One 2013; 8:e62847; http://dx.doi.org/10.1371/journal.pone.0062847; PMID: 23667530
- Rosenberg SA, Yang JC, Sherry RM, Kammula US, Hughes MS, Phan GQ, Citrin DE, Restifo NP, Robbins PF, Wunderlich JR, et al. Durable complete responses in heavily pretreated patients with metastatic melanoma using T-cell transfer immunotherapy. Clin Cancer Res 2011; 17:4550 - 7; http://dx.doi.org/10.1158/1078-0432.CCR-11-0116; PMID: 21498393
- Izumi T, Kondo M, Takahashi T, Fujieda N, Kondo A, Tamura N, Murakawa T, Nakajima J, Matsushita H, Kakimi K. Ex vivo characterization of γδ T-cell repertoire in patients after adoptive transfer of Vγ9Vδ2 T cells expressing the interleukin-2 receptor β-chain and the common γ-chain. Cytotherapy 2013; 15:481 - 91; http://dx.doi.org/10.1016/j.jcyt.2012.12.004; PMID: 23391461
- Moser B, Eberl M. γδ T-APCs: a novel tool for immunotherapy?. Cell Mol Life Sci 2011; 68:2443 - 52; http://dx.doi.org/10.1007/s00018-011-0706-6; PMID: 21573785
- Palucka K, Banchereau J. Cancer immunotherapy via dendritic cells. Nat Rev Cancer 2012; 12:265 - 77; http://dx.doi.org/10.1038/nrc3258; PMID: 22437871
- Escudier B, Farace F, Angevin E, Charpentier F, Nitenberg G, Triebel F, Hercend T. Immunotherapy with interleukin-2 (IL2) and lymphokine-activated natural killer cells: improvement of clinical responses in metastatic renal cell carcinoma patients previously treated with IL2. Eur J Cancer 1994; 30A:1078 - 83; http://dx.doi.org/10.1016/0959-8049(94)90460-X; PMID: 7654433
- deMagalhaes-Silverman M, Donnenberg A, Lembersky B, Elder E, Lister J, Rybka W, Whiteside T, Ball E. Posttransplant adoptive immunotherapy with activated natural killer cells in patients with metastatic breast cancer. J Immunother 2000; 23:154 - 60; http://dx.doi.org/10.1097/00002371-200001000-00018; PMID: 10687148
- Ishikawa E, Tsuboi K, Saijo K, Harada H, Takano S, Nose T, Ohno T. Autologous natural killer cell therapy for human recurrent malignant glioma. Anticancer Res 2004; 24:3b 1861 - 71; PMID: 15274367
- Hamid O, Robert C, Daud A, Hodi FS, Hwu W-J, Kefford R, Wolchok JD, Hersey P, Joseph RW, Weber JS, et al. Safety and tumor responses with lambrolizumab (anti-PD-1) in melanoma. N Engl J Med 2013; 369:134 - 44; http://dx.doi.org/10.1056/NEJMoa1305133; PMID: 23724846
- Hodi FS, O’Day SJ, McDermott DF, Weber RW, Sosman JA, Haanen JB, Gonzalez R, Robert C, Schadendorf D, Hassel JC, et al. Improved survival with ipilimumab in patients with metastatic melanoma. N Engl J Med 2010; 363:711 - 23; http://dx.doi.org/10.1056/NEJMoa1003466; PMID: 20525992
- Singh N, Madan RA, Gulley JL. Ipilimumab in prostate cancer. Expert Opin Biol Ther 2013; 13:303 - 13; http://dx.doi.org/10.1517/14712598.2012.754421; PMID: 23265575
- Martinet L, Fleury-Cappellesso S, Gadelorge M, Dietrich G, Bourin P, Fournié J-J, Poupot R. A regulatory cross-talk between Vgamma9Vdelta2 T lymphocytes and mesenchymal stem cells. Eur J Immunol 2009; 39:752 - 62; http://dx.doi.org/10.1002/eji.200838812; PMID: 19197941
- Mussai F, De Santo C, Cerundolo V. Interaction between invariant NKT cells and myeloid-derived suppressor cells in cancer patients: evidence and therapeutic opportunities. J Immunother 2012; 35:449 - 59; http://dx.doi.org/10.1097/CJI.0b013e31825be926; PMID: 22735803
- Magnus T, Schreiner B, Korn T, Jack C, Guo H, Antel J, Ifergan I, Chen L, Bischof F, Bar-Or A, et al. Microglial expression of the B7 family member B7 homolog 1 confers strong immune inhibition: implications for immune responses and autoimmunity in the CNS. J Neurosci 2005; 25:2537 - 46; http://dx.doi.org/10.1523/JNEUROSCI.4794-04.2005; PMID: 15758163
- Benzaïd I, Mönkkönen H, Stresing V, Bonnelye E, Green J, Mönkkönen J, Touraine JL, Clézardin P. High phosphoantigen levels in bisphosphonate-treated human breast tumors promote Vgamma9Vdelta2 T-cell chemotaxis and cytotoxicity in vivo. Cancer Res 2011; 71:4562 - 72; http://dx.doi.org/10.1158/0008-5472.CAN-10-3862; PMID: 21646473
- Choudhary A, Davodeau F, Moreau A, Peyrat MA, Bonneville M, Jotereau F. Selective lysis of autologous tumor cells by recurrent gamma delta tumor-infiltrating lymphocytes from renal carcinoma. J Immunol 1995; 154:3932 - 40; PMID: 7706731