Abstract
Previous studies have shown that genetic quantitative trait loci (QTL), strain barriers, inoculation dose and inoculation method modulate the incubation period of prion diseases. We examined the relationship between a diverse set of physical, genetic and immunological characteristics and the incubation period of prion disease using correlation analyses. We found that incubation period was highly correlated with brain weight. In addition, mean corpuscular volume and cell size were strongly correlated with incubation period, indicating that the physical magnitude of prion-infected organs or individual cells may be important in determining the incubation period. Given the same prion inoculation dose, animals with a lower brain weight, mean corpuscular volume or cell size may experience more virulent disease, as the effective concentration of abnormal prion, which might regulate the attachment rate of prions to aggregates, is increased with smaller capacity of brains and cells. This is partly consistent with previous theoretical modeling. The strong correlations between incubation period and physical properties of the brain and cells in this study suggest that the mechanism underlying prion disease pathology may be physical, indicating that the incubation process is governed by simple chemical stoichiometry.
Introduction
Transmissible spongiform encephalopathies (TSE) are fatal neurodegenerative diseases that arise from the accumulation of an abnormal isoform of prion protein (i.e., PrPSc). PrPSc is known to induce the conversion of the normal cellular isoform of prion protein (PrPC) into PrPSc. According to the results of secondary structure comparison study which was performed on the proteins purified from Syrian hamster (SHa) brains,Citation1 structural modedeling,Citation2 and NMR studies,Citation3,Citation4 PrPC is rich in α-helical content and has little β-sheet structure, whereas PrPSc has less α-helical content and a high β-sheet structure.Citation5
Marked differences in the incubation periods of TSE have been reported within infected murineCitation6,Citation7 and ovineCitation8 species. In a rodent model, the shortest incubation periods were achieved with an intracerebral (i.c.) inoculation of prions that showed identical sequences to that of the host animal.Citation7 When the PrP sequence of the donor prion differs from that of the recipient host, the incubation time is prolonged and can be quite variable; often, many such inoculated animals do not develop disease.Citation5,Citation9-Citation12 This effect consists part of the species barrier.Citation5,Citation13 Incubation time reflects species barrier effects in infections that occur across species, and the first passage has a significantly longer incubation time than subsequent passages.Citation14-Citation16
The Sinc and Prn-i genes were known to be the major determinants of incubation time in mice.Citation5 These are now known to be identical to the PRNP gene.Citation5,Citation17,Citation18 Although the inherited alleles of PRNP gene determines the large part of the susceptibility of TSE, there is discrepancy in the susceptibility and incubation times between individuals with similar PRNP genotypes.Citation8 Some genetic loci on genes other than PRNP have been shown to modulate the incubation time of prion diseases in mice,Citation19-Citation23 cattle,Citation24,Citation25 sheepCitation8,Citation26,Citation27 and humans.Citation28
The abbreviation, rather than general prolongation, of incubation periods due to strain barriers for particular prion strains that were transferred across species, as compared with the incubation period for passage within the same species, has also been observed.Citation5 The variation in incubation times induced by strain barriers is presumably due to differences in the tertiary and quaternary structures of prion strains.Citation29-Citation33
Inoculation dose is also an important determinant of incubation period length. In previous studies, a model describing the dependence of incubation period on infection dose changed from a broad distribution, with deviation from logarithmic behavior, to logarithmic dependence and, finally, to a sharply defined dose-independent saturated value, as inoculation dose increased.Citation16,Citation34
In this study, we sought to identify novel factors that influence the prion incubation period among various species. We investigated specific variables, including brain weight (BW), body weight, maximum lifespan (MLS), total sleep (TS), white blood cell (WBC) count, red blood cell (RBC) count, cell size (CS), mean corpuscular volume (MCV), chromosome number (CN) and genome size (GS). WBC and RBC counts are known to be important indicators of immune activity.Citation35,Citation36 Genetic variables were used to represent possible genetic mechanisms, such as quantitative trait loci (QTL), which can influence incubation period. Physical and general biological attributes were also considered in our analyses.
Results
In this study, we analyzed six prion diseases in four susceptible species. Ten biological characteristics of susceptible animals for each prion disease were obtained and used to assess their correlation with incubation period. All 11 variables are listed according to the four types of prion diseases (i.e., scrapie, BSE, Kuru, and two types of iatrogenic CJD) in . Most of the 10 variables except incubation period showed rather broad correlations (mean R2 of 0.59) with others. MCV (R2 of 0.74), CS (R2 of 0.73) and BW (R2 of 0.72) showed high correlations with other 9 variables with the mean correlation coefficient above 0.70, while body weight and GS showed very small correlations with others with the mean correlation coefficient of 0.30 and 0.13, respectively.
Table 1. Correlation between various characteristics and the incubation period of prion diseases
We performed correlation analysis, as described in the Materials and Methods section, and the resultant p-values are shown in . Well-correlated variables (p < 0.02) are indicated in bold text. The variable showing the strongest correlation was BW (p ~0.0021), followed by MCV (p ~0.0022), both of which increased with increasing incubation time (). MLS and CS were also highly correlated with incubation period (p = 0.0033 and p = 0.0043, respectively; ). TS was also significantly correlated with the incubation period (p = 0.0052). Variables with p-values > 0.02 included genetic (GS and CN), blood-related (RBC and WBC counts), and physical (weight) factors. The p-values for the correlations between these factors and incubation period ranged from 0.0248 to 0.7628.
Figure 1. The top four factors correlated with the incubation period of prion disease. Correlation plots of (a) brain weight (BW), (b) mean corpuscular volume (MCV), (c) maximum lifespan (MLS), and (d) cell size (CS) vs. incubation period are shown. The p-values for the correlations were p ~0.002 for BW and MCV, p = 0.0033 for MLS, and p = 0.0043 for CS.
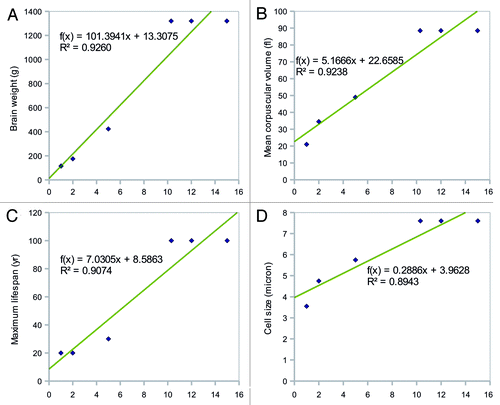
BW showed the strongest correlation with incubation period. MCV and CS were also highly correlated with incubation period, indicating that the physical magnitude of prion-infected organs or individual cells is important for the determination of the length of the latent period. Given the same inoculation dose of prion, animals with smaller BW, MCV, and CS may have more virulent disease and shorter latent periods than larger animals. This is possibly because the large capacity of brain and cell effectively decreases the concentration of abnormal prions which might determine the rate of attachments to the existing aggregates.
According to Kulkarni et al.,Citation16 incubation period of prion disease is determined by the time needed for individual aggregate growth following the duplication of these grown seeds until certain number of seeds is formed. It is true that larger brain needs more number of seeds to develop prion disease and thus need more rounds of replications. However, the authors insisted that the order of magnitude longer total incubation time of big animal (e.g., cow in BSE) to small animal is must ascribed to slower attachment rates of prions to aggregates of abnormal prions because of the exponential property of the doubling phase and comparable doubling time constant of big animal to that of the small animal.Citation16
The most probable contributor to the attachment rate was the concentration of prions of any forms in their model, and the authors implied that the low metabolism rate of big animal might be the cause of the low concentration of prions in BSE. However, this assertion based on the metabolism rate of big animal seems to be false for the low correlation of body weight and incubation period which was revealed in this study. We suggest, instead, that the effective concentration of pathogenic prions of infected organ and individual cell might influence the attachment rates, which partly agrees with this interpretation of BSE incubation period of previous modeling.Citation16
MLS was another significant factor influencing the incubation period length (p = 0.0033). Previous studies have demonstrated a correlation between BW and MLS, which may explain the correlation we detected between MLS and incubation period.Citation37-Citation39 The cause of the significant relationship between TS and incubation period is unclear. It is possible that sleep prolongs infection by stimulating metabolic processes that fight the aggregation of abnormal prions. Alternatively, the length of sleep may correlate with BW for reasons that are unrelated to prion disease.
General immunological activity may help prevent the aggregation of abnormal proteins (i.e., PrPSc). However, immunological characteristics, including WBC count and RBC count, were not significantly correlated with incubation period (p = 0.0403 and p = 0.0264, respectively). Although further analyses are required to confirm these results, it appears that immune activity (as measured by WBC and RBC count) is not strongly associated with the incubation period of the six prion diseases analyzed in this study.
Genetic variables, including GS and CN, showed rather weak correlations (p = 0.3256 and p = 0.0248, respectively) with the prion disease incubation period. This may indicate that the scale of the genome does not correlate well with the number of possible occurrences of QTL, or that the effect of QTL on incubation period is nonlinear, even if larger genomes have more QTL. It is also possible that large genomic spaces do not act as barriers to prion infection, which would otherwise support longer incubation periods.
In contrast to brain weight (BW), body weight showed a weak correlation (p = 0.7628) with the incubation period of prion disease. This characteristic had the least influence on the latent period of prion disease among the variables analyzed in this study. Considering that BW showed a marked association with incubation period, we speculate that the mass of organs and tissues other than the brain are less important to the pathology of prion diseases. It is also possible to conclude that large body masses do not sufficiently dilute infectious prions as compared with small bodies, which differs from the case of brains.
Discussion
The incubation period of prion diseases is long as compared with other common diseases. However, the genetic and biological reasons for these longer incubation periods remain unclear. In this study, we investigated numerous genetic, biological, and physical factors for their potential to significantly affect the incubation period.
BW showed a strong positive correlation with incubation period length (p ~0.0021). We postulated that the large brain effectively reduces the concentration of pathogenic prions by more solvable capacity leading to the decrease of attachment rate of prions to existing aggregates. We also made similar suggestions for MCV and cell size. However, more profound experimental data, especially of the big animals with prion diseases, would help to solidly postulate the possible mechanism of the size of the brain in the determination of the latent period of prion disease. Once data of such scale are available, one might scrutinize the possible relationship of the size of brains and cells with incubation period based on the analysis of the attachment rates.
The incubation data used here were selected to remove any influences of species barrier effects, although the data were obtained using various inoculation methods and dosages. Experimental data obtained under more robust conditions would be helpful to reveal a more accurate picture of the relationship between incubation period and numerous factors, including the ones considered in this study. Regardless, the marked correlation of physical properties of both the brain and individual cells with incubation period that we detected here suggests that the mechanism underlying prion disease pathology may be physical, in which the incubation process is governed by simple chemical stoichiometric principles.
Materials and Methods
Variable selection
We analyzed the relationship between a diverse set of characteristics and the length of the incubation period of prion diseases. We included physical (i.e., BW, body weight, MCV, and dry RBC size), genetic (i.e., GS and CN), and immunological factors (i.e., WBC count and RBC count), as well as other possible contributors, such as MLS and TS length, in our analyses. Physical properties were included to examine the potential relationships between physical dimensions of infected individuals, organs, and cells and the incubation period of prion diseases. Dry RBC size was used to circumvent the difficulty of obtaining general cell size data. MCV was used to approximate general cellular volume. MCV was measured from intact cells, while CS was obtained from dry RBC.
Data collection
The incubation periods of caprine scrapie,Citation5,Citation40 ovine scrapie,Citation5 bovine spongiform encephalopathy (BSE),Citation5,Citation41 human Kuru,Citation42 human growth hormone-type iatrogenic Creutzfeldt-Jakob disease (iCJD),Citation43 and human gonadotropin-type iCJDCitation43 were obtained from previously published studies. We used infection cases from early studies of scrapie instead of considering and averaging the effects of many quantitative trait loci (QTL). Mean incubation period of 1.0 y with the range of 0.67 to 1.83 and variance of 1.42 y from 17 goats were used for the caprine scrapie incubation period.Citation5,Citation40 Estimated mean of 2 y from the range of 1 to 3 y from early studies was used as the incubation period of ovine scrapie.Citation5 We also used published research on the incubation period based on the epidemics for other types of analyzed TSE. Mean of 5 y with variance of ~1.6 y from the statistical analysis of epidemiological data of BSE was used for the BSE incubation peiod.Citation5,Citation41 Mean incubation time of 10.3 y with 91% confidence interval of 9.8 to 11.1 y from Weibull model from 2486 death cases, which was the best fit according to χ2-test, was used as the incubation period of human kuru.Citation42 Mean of 15 y with the range of 4–36 y from 194 clinical cases was used for the human growth hormone-type iCJD and mean of 13 y with the range of 12 to 16 y from 4 clinical cases was used for human gonadotropin-type iCJD.Citation43
Ideally, prions with the same sequence to the host animal prion should be injected using the same inoculation method and with weight-leveled doses to obtain the shortest incubation time for each organisms. This is partly supported by the studies in a rodent model showing that the fastest incubation periods are observed upon infection with a prion with the same sequence as the host prion.Citation5 This was believed to settle the effects of strain differences and host prion polymorphisms since the almost identical infected prions and host PRNP genes would induce the close to the shortest incubation time for each case of species. Although we were unable to validate whether the scrapie, BSE, Kuru, and iCJD prions which infected their respective hosts had the same sequence as the hosts’ prions, we assumed their similarity because they were from the same species.
We excluded prion diseases caused by infection across species in order to accurately examine the relationships between incubation period and various characteristics without the confounding effects. We included data of non-passaged scrapie; however, it could not be confirmed whether iCJD, Kuru, and BSE strains had undergone multiple passages.
We also excluded some types of iCJD cases with massive transplantations and only considered infections using moderate doses. For the current data set, inoculation methods varied and included subcutaneous (s.c.; used in scrapie and iCJD cases), intramuscular (i.m.; used in iCJD cases), and oral (used in Kuru and BSE cases) inoculations. We excluded intracerebral (i.c.) infection, which shows a drastically shortened incubation period. We included oral infections since we were unable to identify s.c. or i.m. infection data for BSE or human Kuru.
We tried to use epidemic studies of natural outbreaks infected within the same species to generalize the effects of parameters including sex and various QTLs. In the cases when this was not possible, as in the case of scrapie, we used incubation period results from early studies.
We recognize that this type of data selection has limitations, especially in the light of the fact that small sequence differences between the infecting and host prions may have confounding effects. Although many more constraints should be used to correctly determine and compare incubation periods for different species, to our knowledge, this was the best possible approach, particularly considering the lack of tightly controlled previous experimental results and difficulty of experimental investigation and necessity of the analysis of big animals.
We obtained BW, MLS, body weight, and TS data from a website administered by the University of Illinois (http://mste.illinois.edu/regression/species.html).Citation44 We obtained WBC and RBC counts of normal, healthy animals from credible information provided in the literature.Citation45-Citation47 We obtained MCV, CS, and GS data from the animal genome size database (http://www.genomesize.com/cellsize/mammals.htm), as well as from numerous studies.Citation48-Citation62 We also gathered CN data from previously reported studies.Citation44,Citation45,Citation63
Statistical analyses
We analyzed the relationship between each of the 10 variables and incubation period using correlation analyses. A correlation analysis represents the linear relationship between two variables and calculates the significance of this relationship using T-tests, which are based on the correlation coefficients. Our goal was to determine which factors are significantly related to incubation period using p-values derived from this analysis. The p-values of smaller than 0.02 were considered significant, whereas p-values of larger than 0.02 were considered non-significant trends. Simple spreadsheet applications of Excel of MS Office 2007 packageCitation64 were used for these analyses.
Disclosure of Potential Conflicts of Interest
No potential conflicts of interest were disclosed.
Acknowledgments
This work was supported by the BK 21 project in 2011. We also acknowledge the invaluable contribution of the researchers who have made their data publicly available.
Reference
- Pan K-M, Baldwin M, Nguyen J, Gasset M, Serban A, Groth D, et al. Conversion of α-helices into β-sheets features in the formation of the scrapie prion proteins. Proc Natl Acad Sci USA 1993; 90:10962 - 6; http://dx.doi.org/10.1073/pnas.90.23.10962; PMID: 7902575
- Huang Z, Prusiner SB, Cohen FE. Scrapie prions: a three-dimensional model of an infectious fragment. Fold Des 1995; 1:13 - 9; http://dx.doi.org/10.1016/S1359-0278(96)00007-7; PMID: 9162135
- Riek R, Hornemann S, Wider G, Billeter M, Glockshuber R, Wüthrich K. NMR structure of the mouse prion protein domain PrP(121-231). Nature 1996; 382:180 - 2; http://dx.doi.org/10.1038/382180a0; PMID: 8700211
- Liu H, Farr-Jones S, Ulyanov NB, Llinas M, Marqusee S, Groth D, et al. Solution structure of Syrian hamster prion protein rPrP(90-231). Biochemistry 1999; 38:5362 - 77; http://dx.doi.org/10.1021/bi982878x; PMID: 10220323
- Prusiner SB. Prion Biology and Diseases. New York: Cold Spring Harbor Laboratory Press, 2004:3,10,14,17-18,143-144,600,1021.
- Kimberlin RH, Walker CA. Incubation periods in six models of intraperitoneally injected scrapie depend mainly on the dynamics of agent replication within the nervous system and not the lymphoreticular system. J Gen Virol 1988; 69:2953 - 60; http://dx.doi.org/10.1099/0022-1317-69-12-2953; PMID: 3143808
- Barron RM, Thomson V, Jamieson E, Melton DW, Ironside J, Will R, et al. Changing a single amino acid in the N-terminus of murine PrP alters TSE incubation time across three species barriers. EMBO J 2001; 20:5070 - 8; http://dx.doi.org/10.1093/emboj/20.18.5070; PMID: 11566872
- Moreno CR, Cosseddu GM, Schibler L, Roig A, Moazami-Goudarzi K, Andreoletti O, et al. Identification of new quantitative trait Loci (other than the PRNP gene) modulating the scrapie incubation period in sheep. Genetics 2008; 179:723 - 6; http://dx.doi.org/10.1534/genetics.108.088146; PMID: 18493086
- Carlson GA, Westaway D, DeArmond SJ, Peterson-Torchia M, Prusiner SB. Primary structure of prion protein may modify scrapie isolate properties. Proc Natl Acad Sci USA 1989; 86:7475 - 9; http://dx.doi.org/10.1073/pnas.86.19.7475; PMID: 2798418
- Telling GC, Scott M, Hsiao KK, Foster D, Yang S-L, Torchia M, et al. Transmission of Creutzfeldt-Jakob disease from humans to transgenic mice expressing chimeric human-mouse prion protein. Proc Natl Acad Sci U S A 1994; 91:9936 - 40; http://dx.doi.org/10.1073/pnas.91.21.9936; PMID: 7937921
- Telling GC, Scott M, Mastrianni J, Gabizon R, Torchia M, Cohen FE, et al. Prion propagation in mice expressing human and chimeric PrP transgenes implicates the interaction of cellular PrP with another protein. Cell 1995; 83:79 - 90; http://dx.doi.org/10.1016/0092-8674(95)90236-8; PMID: 7553876
- Tateishi J, Kitamoto T, Hoque MZ, Furukawa H. Experimental transmission of Creutzfeldt-Jakob disease and related diseases to rodents. Neurology 1996; 46:532 - 7; PMID: 8614527
- Pattison IH. Experiments with scrapie with special reference to the nature of the agent and the pathology of the disease. In: Gajdusek DC, et al. eds. Slow, Latent and Temperate Virus Infections (NINDB Monogr 2). Washington DC: U.S. Government Printing Office, 1965:249-257.
- Kimberlin RH, Walker CA. Characteristics of a short incubation model of scrapie in the golden hamster. J Gen Virol 1977; 34:295 - 304; http://dx.doi.org/10.1099/0022-1317-34-2-295; PMID: 402439
- Kimberlin RH, Walker CA. Pathogenesis of scrapie: agent multiplication in brain at the first and second passage of hamster scrapie in mice. J Gen Virol 1979; 42:107 - 17; http://dx.doi.org/10.1099/0022-1317-42-1-107; PMID: 103999
- Kulkarni RV, Slepoy A, Singh RRP, Cox DL, Pázmándi F. Theoretical modeling of prion disease incubation. Biophys J 2003; 85:707 - 18; http://dx.doi.org/10.1016/S0006-3495(03)74514-7; PMID: 12885622
- Carlson GA, Kingsbury DT, Goodman PA, Coleman S, Marshall ST, DeArmond S, et al. Linkage of prion protein and scrapie incubation time genes. Cell 1986; 46:503 - 11; http://dx.doi.org/10.1016/0092-8674(86)90875-5; PMID: 3015416
- Moore RC, Hope J, McBride PA, McConnell I, Selfridge J, Melton DW, et al. Mice with gene targetted prion protein alterations show that Prnp, Sinc and Prni are congruent. Nat Genet 1998; 18:118 - 25; http://dx.doi.org/10.1038/ng0298-118; PMID: 9462739
- Stephenson DA, Chiotti K, Ebeling C, Groth D, DeArmond SJ, Prusiner SB, et al. Quantitative trait loci affecting prion incubation time in mice. Genomics 2000; 69:47 - 53; http://dx.doi.org/10.1006/geno.2000.6320; PMID: 11013074
- Lloyd SE, Onwuazor ON, Beck JA, Mallinson G, Farrall M, Targonski P, et al. Identification of multiple quantitative trait loci linked to prion disease incubation period in mice. Proc Natl Acad Sci U S A 2001; 98:6279 - 83; http://dx.doi.org/10.1073/pnas.101130398; PMID: 11353827
- Manolakou K, Beaton J, McConnell I, Farquar C, Manson J, Hastie ND, et al. Genetic and environmental factors modify bovine spongiform encephalopathy incubation period in mice. Proc Natl Acad Sci U S A 2001; 98:7402 - 7; http://dx.doi.org/10.1073/pnas.121172098; PMID: 11404459
- Lloyd SE, Uphill JB, Targonski PV, Fisher EM, Collinge J. Identification of genetic loci affecting mouse-adapted bovine spongiform encephalopathy incubation time in mice. Neurogenetics 2002; 4:77 - 81; http://dx.doi.org/10.1007/s10048-002-0133-9; PMID: 12481985
- Moreno CR, Lantier F, Lantier I, Sarradin P, Elsen JM. Detection of new quantitative trait Loci for susceptibility to transmissible spongiform encephalopathies in mice. Genetics 2003; 165:2085 - 91; PMID: 14704188
- Hernández-Sánchez J, Waddington D, Wiener P, Haley CS, Williams JL. Genome-wide search for markers associated with bovine spongiform encephalopathy. Mamm Genome 2002; 13:164 - 8; http://dx.doi.org/10.1007/BF02684022; PMID: 11919688
- Zhang C, De Koning DJ, Hernández-Sánchez J, Haley CS, Williams JL, Wiener P. Mapping of multiple quantitative trait loci affecting bovine spongiform encephalopathy. Genetics 2004; 167:1863 - 72; http://dx.doi.org/10.1534/genetics.104.026401; PMID: 15342524
- Marcos-Carcavilla A, Moreno C, Serrano M, Laurent P, Cribiu EP, Andréoletti O, et al. Polymorphisms in the HSP90AA1 5′ flanking region are associated with scrapie incubation period in sheep. Cell Stress Chaperones 2010; 15:343 - 9; http://dx.doi.org/10.1007/s12192-009-0149-2; PMID: 19838832
- Moreno CR, Moazami-Goudarzi K, Briand S, Robert-Granié C, Weisbecker JL, Laurent P, et al. Mapping of quantitative trait loci affecting classical scrapie incubation time in a population comprising several generations of scrapie-infected sheep. J Gen Virol 2010; 91:575 - 9; http://dx.doi.org/10.1099/vir.0.014134-0; PMID: 19828762
- Yun J, Jin HT, Lee YJ, Choi EK, Carp RI, Jeong BH, et al. The first report of RPSA polymorphisms, also called 37/67 kDa LRP/LR gene, in sporadic Creutzfeldt-Jakob disease (CJD). BMC Med Genet 2011; 12:108; http://dx.doi.org/10.1186/1471-2350-12-108; PMID: 21838916
- Bessen RA, Marsh RF. Distinct PrP properties suggest the molecular basis of strain variation in transmissible mink encephalopathy. J Virol 1994; 68:7859 - 68; PMID: 7966576
- Telling GC, Parchi P, DeArmond SJ, Cortelli P, Montagna P, Gabizon R, et al. Evidence for the conformation of the pathologic isoform of the prion protein enciphering and propagating prion diversity. Science 1996; 274:2079 - 82; http://dx.doi.org/10.1126/science.274.5295.2079; PMID: 8953038
- Scott MR, Groth D, Tatzelt J, Torchia M, Tremblay P, DeArmond SJ, et al. Propagation of prion strains through specific conformers of the prion protein. J Virol 1997; 71:9032 - 44; PMID: 9371560
- Scott MR, Will R, Ironside J, Nguyen H-OB, Tremblay P, DeArmond SJ, et al. Compelling transgenetic evidence for transmission of bovine spongiform encephalopathy prions to humans. Proc Natl Acad Sci U S A 1999; 96:15137 - 42; http://dx.doi.org/10.1073/pnas.96.26.15137; PMID: 10611351
- Peretz D, Williamson RA, Legname G, Matsunaga Y, Vergara J, Burton DR, et al. A change in the conformation of prions accompanies the emergence of a new prion strain. Neuron 2002; 34:921 - 32; http://dx.doi.org/10.1016/S0896-6273(02)00726-2; PMID: 12086640
- McLean AR, Bostock CJ. Scrapie infections initiated at varying doses: an analysis of 117 titration experiments. Philos Trans R Soc Lond B Biol Sci 2000; 355:1043 - 50; http://dx.doi.org/10.1098/rstb.2000.0641; PMID: 11186305
- Grogan ED, Lund R. Reactivity of human white blood cells to factors of elasmobranch origin. Copeia 1991; 2:402 - 8; http://dx.doi.org/10.2307/1446589
- Snyder GK, Sheafor BA. Red blood cells: centerpiece in the evolution of the vertebrate circulatory system. Am Zool 1999; 39:189 - 98
- Sacher GA. Relation of lifespan to brain weight and body weight in mammals, In: Wolstenholme GEW and O’Conner M eds. CIBA Foundation Colloquia on Aging, 1959; 5:115-141.
- Sacher GA. Longevity and aging in vertebrate evolution. Bioscience 1978; 28:497 - 501; http://dx.doi.org/10.2307/1307295
- Allman J, McLaughlin T, Hakeem A. Brain weight and life-span in primate species. Proc Natl Acad Sci U S A 1993; 90:118 - 22; http://dx.doi.org/10.1073/pnas.90.1.118; PMID: 8419913
- Pattison IH. The relative susceptibility of sheep, goats and mice to two types of the goat scrapie agent. Res Vet Sci 1966; 7:207 - 12; PMID: 4163198
- Anderson RM, Donnelly CA, Ferguson NM, Woolhouse MEJ, Watt CJ, Udy HJ, et al. Transmission dynamics and epidemiology of BSE in British cattle. Nature 1996; 382:779 - 88; http://dx.doi.org/10.1038/382779a0; PMID: 8752271
- Huillard d’Aignaux JN, Cousens SN, Maccario J, Costagliola D, Alpers MP, Smith PG, et al. The incubation period of kuru. Epidemiology 2002; 13:402 - 8; http://dx.doi.org/10.1097/00001648-200207000-00007; PMID: 12094094
- Brown P, Brandel J-P, Preece M, Sato T. Iatrogenic Creutzfeldt-Jakob disease: the waning of an era. Neurology 2006; 67:389 - 93; http://dx.doi.org/10.1212/01.wnl.0000231528.65069.3f; PMID: 16855204
- Allison T, Cicchetti DV. Sleep in mammals: ecological and constitutional correlates. Science 1976; 194:732 - 4; http://dx.doi.org/10.1126/science.982039; PMID: 982039
- Wigal RA, Coggins VL. Mountain goat. In: Wild Mammals of North America: Biology, Management, Economics. The Johns Hopkins University Press. 1982:1008-1020.
- Voight GL. Hematology Techniques and Concepts for Veterinary Technicians. 1st. Ames, Iowa: Iowa State University Press, 2000:51.
- Pisek L, Travnicek J, Salat J, Kroupova V, Soch M. Changes in white blood cells in sheep blood during selenium supplementation. Vet Med-Czech 2008; 53:255 - 9
- Malassez ML. De la numération des globules rouges du sang chez les mammifères, les oisseaux et les poissons. Comptes Rendus de l'Académie des Sciences 1872; 75:1528 - 31
- Gulliver G. Observations on the sizes and shapes of the red corpuscles of the blood of vertebrates, with drawings of them to a uniform scale, and extended and revised tables of measurements. Proc Zool Soc Lond 1875; 474 - 95
- Cleland JB, Johnston TH. Relative dimensions of the red blood cells of vertebrates, especially of birds. Emu 1912; 11:188 - 97; http://dx.doi.org/10.1071/MU911188
- Emmons WF. The interrelation of number, volume, diameter and area of mammalian erythrocytes. J Physiol 1927; 64:215 - 28; PMID: 16993914
- Wintrobe MM. Variations in the size and hemoglobin content of erythrocytes in the blood of various vertebrates. Folia Haematol (Frankf) 1933; 51:32 - 49
- Ponder E. Hemolysis and Related Phenomena. New York: Grune and Stratton, 1948.
- Kisch B. Hemoglobin content, size and amount of erythrocytes in fishes. Exp Med Surg 1949; 7:118 - 33; PMID: 18151280
- Kisch B. Observations on the haematology of fishes and birds. Exp Med Surg 1949; 7:318 - 26, illust; PMID: 15396127
- Albritton EC. Standard Values in Blood. WB Saunders Company, 1954:409.
- Dunaway PB, Lewis LL. Taxonomic relation of erythrocyte count, mean corpuscular volume, and body weight in mammals. Nature 1965; 205:481 - 4; http://dx.doi.org/10.1038/205481a0; PMID: 14265293
- Huser HJ. Atlas of Comparative Primate Hematology. Academic Press, 1970:405.
- Hawkey CM. Comparative Mammalian Haematology. London: William Heinemann Medical Books, 1975.
- Withers PC. Comparative Animal Physiology. New York: Saunders College Publishing, 1992.
- Ragan HA. Comparative hematology. In: Wintrobe's Clinical Hematology. Baltimore: Williams & Wilkins, 1999:2749-2763.
- Benga G, Kuchel PW, Chapman BE, Cox GC, Ghiran I, Gallagher CH. Comparative cell shape and diffusional water permeability of red blood cells from Indian elephant (Elephas maximus) and Man (Homo sapiens). Comp Haematol Int 2000; 10:1 - 8; http://dx.doi.org/10.1007/s005800070020
- Ratcliffe NA, Millar DA. Comparative aspects and possible phylogenetic affinities of vertebrate and invertebrate blood cells. In: Roweley AF, Ratcliffe NA eds. Vertebrate Blood Cells. Cambridge University Press, 1988.
- Microsoft Corporation. MS office 2007. Redmond, WA, USA 2007.