Abstract
We recently showed in a publication in Nature that the eukaryotic translation initiation factor eIF5 has a second regulatory function and is a GDI (GDP dissociation inhibitor) in addition to its previously characterized role as a GAP (GTPase accelerating protein). These findings provide new insight into the mechanism of translation initiation in eukaryotic cells. Additional findings show that the GDI function is critical for the normal regulation of protein synthesis by phosphorylation of eIF2α at ser51. Because eIF2 phosphorylation is a ubiquitous mode of translational control these results are of broad interest. Here we review these and related studies and suggest they offer further evidence of parallels between the functions of regulators of the translation factor eIF2 and both heterotrimeric and small GTPases.
Overview of the eIF2 Cycle in Translation Initiation
Eukaryotic Initiation Factor 2 (eIF2) is a GTP-binding protein that functions during protein translation initiation to deliver initiator methionyl tRNA to the ribosome in all eukaryotic cells. This ensures that protein synthesis is initiated at an appropriate AUG codon. Excellent and detailed reviews of the translation process have been presented elsewhere.Citation1,Citation2 Here we focus on the process from the perspective of the eIF2 G protein cycle (). eIF2 binds Met-tRNAi only when in its active GTP-bound form before interacting with the small 40S ribosomal subunit and other initiation factors including eIFs 1, 1A, 3 and 5 to form a 43S pre-initiation complex (PIC). Following mRNA binding at the 5′ end, PIC migration in a 3′ direction (scanning) ensues to identify a suitable initiation codon. eIF2-bound GTP hydrolysis to GDP+Pi is triggered by the GAP activity of eIF5. This occurs on or before AUG start codon recognition, which is signaled by release of eIF1 from the PIC, promoting release from the ribosome/mRNA/Met-tRNAi complex of an eIF2•GDP/eIF5 complex.Citation3,Citation4 In a newly described step, eIF5 acts as a GDI preventing premature GDP release (see below).Citation5 For subsequent rounds of translation initiation to occur eIF2 must be reactivated to allow rebinding of Met-tRNAi. This process is carried out by the GEF eIF2B and is an important point of translation initiation regulation.Citation6 Hence, in a manner functionally analogous to small GTPases and heterotrimeric G proteins, eIF2 activity is controlled by the GAP, GDI and GEF activities of eIF5 and eIF2B ().
eIF2 is a three subunit protein (αβγ)Citation7 but is distinct from heterotrimeric G-proteins. Nucleotides and Met-tRNAi bind the (largest) γ subunit, while the β subunit makes important contacts with its binding partners (including eIFs 2B, 3 and 5). One well-characterised way of regulating eIF2 activity is by the phosphorylation of eIF2α subunit at Ser 51 [eIF2(αP)] by any of several ser/thr kinases in response to various different cellular stresses. In yeast there is a single eIF2α kinase, Gcn2p, that is primarily activated in response to amino acid deprivation.Citation8 Phosphorylated eIF2 acts as a competitive inhibitor for its own GEF, eIF2B causing a reduction in eIF2-GTP and general translation levels as well as activation of translationally-controlled stress response genes such as ATF4 (in mammals) and GCN4 (yeast).Citation8
eIF5 has GDI Activity
Several lines of evidence suggested that eIF5 had a role in translation initiation in addition to its functions in PIC assembly and GAP activity. It had been shown that eIF5 interacts with eIF2 in both its GDP and GTP form with little difference in affinity.Citation9 This is an unusual finding for a protein thought to solely function with eIF2•GTP•Met-tRNAi complexes. Secondly it was demonstrated that a significant fraction of yeast eIF2 is found in complex with eIF5 in the absence of Met-tRNAi.Citation4 As eIF2•GTP has a high affinity for Met-tRNAi, the eIF2/eIF5 complex was not predicted by eIF5 acting simply as a GAP or standard models for the translation pathway. Genetic evidence suggested that eIF5 can antagonise eIF2B GEF activityCitation10 and fourthly it was shown that eIF2 and eIF5 leave the ribosome at the same time in an in vitro model of initiation.Citation3 Hence it was proposed that eIF2•GDP is released from the PIC in complex with eIF5 and that this complex antagonised eIF2B GEF activity.Citation10 These findings led us to investigate whether eIF5 might also act as a GDI for eIF2 in addition to its GAP function. We demonstrated that eIF5 does indeed possess GDI activity for eIF2•GDP and therefore functions to both stimulate hydrolysis of eIF2 bound GTP (GAP) and prevent the release of GDP (GDI).Citation5
eIF5 is known to possess two independently folded domains: a N-terminal GAP domain bearing residue R15 proposed to act similarly to arginine fingers of GAPs for small GTPasesCitation11–Citation13 linked by a region of unknown structure which we termed the ‘linker region’ (LR) to a C-terminal HEAT repeat structured C-terminal domain (CTD) () known to mediate binding to eIFs 1, 2β and 3c.Citation14,Citation15 We showed that GDI is independent of GAP activity as the R15M mutant and the GAP domain were not required, but instead required both the LR and CTD. We identified a well-conserved motif in the LR, which we term the ‘DWEAR motif’ after the absolutely conserved residues (). Mutation of seven conserved DWEAR motif residues (to generate the LR-7A mutant) or a conserved Trp in the CTD (W391F) each eliminated both GDI activity and binding to eIF2γ (). Our data are consistent with a model where GDI activity of eIF5 is actually two separable functions. First the eIF5-CTD provides for binding between eIF5 and eIF2•GDP, which physically antagonises eIF2B (GEF) binding. Our genetic data suggest that this is most critical function for regulation in vivo. Second the DWEAR motif within the eIF5-LR most likely specifically antagonises spontaneous GEF-independent GDP release. Because the critical DWEAR motif is highly conserved throughout eukaryotes, it is reasonable to suggest that it is critical in all systems. Taken together these observations suggest a new, more complex, path for recycling of the G protein eIF2 between successive rounds of translation initiation.
eIF5 GDI has a Regulatory Role
As indicated above, one common mechanism of translational control is via phosphorylation of eIF2α and the inhibition of eIF2B GEF activity (). Studies employing mouse and other animal and cellular model systems have shown that signaling pathways leading to eIF2 phosphorylation are critical for appropriate cellular responses to diverse stimuli including: viral infection, diet and glucose homeostasis and also for long-term memory formation. Although repressive to general translation, some mRNAs are translationally activated by these responses. One key translationally-controlled mRNA is ATF4 which has upstream open-reading frames in its 5′ leader sequence that mediate this control.Citation16–Citation20 In yeast GCN4 translation is similarly regulated by amino acid starvation and eIF2(αP). Importantly simple genetic and biochemical assays can be used to monitor this response.Citation8 These assays have proved powerful tools to dissect mechanisms of translation and its control. Using some of these assays we showed that the W391F mutant in eIF5, which eliminated GDI activity, impacted on the normal cellular response to eIF2(αP) preventing downregulation of general translation and GCN4 activation.Citation5 We interpret these results as suggesting that antagonism of eIF2B by eIF5 GDI activity is necessary for proper control of eIF2B activity by eIF2(αP). We found that the affinity between eIF5 and eIF2 was enhanced, as we observed a 2-fold increase in the cellular fraction of eIF2 precipitated with eIF5 in amino acid starved cells. It was already known that eIF2B activity is controlled by eIF2(αP).Citation21 This new data indicates that eIF5 GDI activity is also regulated and that eIF5 GDI activity antagonises eIF2B further under conditions when eIF2(αP) is activated. Therefore eIF5 GDI is necessary for tight control of translation initiation.
How do GEF and GDI Regulation Combine to Control eIF2 Activity?
The precise mechanism for inhibition of translation by reducing GEF and GDI functions is not yet clear. The GEF eIF2B is a complex of five separate subunits. Three of these (eIF2Bα, β and δ) all share sequence similarity and genetic and biochemical evidence shows they play direct roles in sensing and binding to each other and phosphorylated eIF2α.Citation21–Citation25 A recent crystal structure of the human eIF2Bα subunit proposed a model for direct binding between phosphorylated eIF2α and a pocket on the surface of eIF2Bα.Citation26 However an alternative model has been suggested based upon both structural data and mutational analyses in yeast.Citation27 Thus there is strong evidence that phosphorylation of eIF2α leads to the formation of a stable complex between eIF2α and three of the five eIF2B subunits. Our finding that eIF2/eIF5 complex stability increases upon amino acid starvation suggests at least two possible models for the combined action of GDI and GEF with phosphorylated eIF2. Firstly, that there are two independent complexes regulated by eIF2(αP). In this model, first an eIF2/GDI complex is more stable, but when eIF2 is released it binds eIF2B in a second stable complex. As eIF5 is not thought to bind directly to phosphorylated eIF2α, either this modification at Ser51 induces a conformational change one or both of the other eIF2 subunits which do bind eIF5, or perhaps there is a further stress-dependent modification yet to discover, perhaps on eIF5 itself? Alternatively it is possible that eIF5 is part of the inhibited complex containing all three factors, ie an eIF5/eIF2(αP)/eIF2B complex. In support of the latter model, both the GEF domain of eIF2Bε and the eIF5-CTD share a common HEAT repeat structureCitation28,Citation29 that bind to the eIF2β and γ subunits.Citation5,Citation30–Citation32 In addition, it was shown that excess eIF5 antagonises GEF mutants (and vice versa).Citation10 Thus from our current knowledge it seems possible that stable binding between eIF5-GDI and eIF2βγ accompanies eIF2B binding to eIF2(αP) and that together both prevent eIF2Bε- eIF2γ interactions and inhibit GEF activity. We are currently testing these ideas experimentally.
Implications of eIF5-GDI for Health and Disease
Our findings that eIF5-GDI activity is part of the regulatory response to eIF2(αP) may have important implications for mammals, including man. eIF5 mutations that reduce the inhibitory effects of eIF2 control may mimic those seen the level of eIF2(αP) is reduced by genetically interfering with the signaling kinases or eIF2α itself. Four protein kinases are known (GCN2, PERK, PKR and HRI) which all phosphorylate eIF2α at Ser51 ().Citation2 Mice and humans with mutations inactivating PERK develop insulin dependent diabetes and other abnormalities.Citation16 In man this disorder is called Wolcott-Rallison Syndrome.Citation33 PKR is important for cellular antiviral responses, and HRI is regulated by haem levels in reticulocytes. GCN2 is important for sensing indispensable amino acids in the hypothalamus and controlling feeding behaviour of rodents.Citation18,Citation34 GCN2 is also important for synaptic plasticity and long-term memory formation in mice.Citation17 While mice with a heterozygous ‘knockin' S51A mutation also show similar memory defectsCitation35 and become obese when fed a high fat diet.Citation19 Thus reduced signaling through eIF2(αP) which impairs translational control through the inability to downregulate eIF2 activity has diverse and significant outcomes. Exploring the impact of eIF5-GDI on these pathways in mammals may also be illuminating.
A progressive and fatal human disease results from inherited mutations in eIF2B subunit genes. Variously called ‘eIF2B-related disorders’ or ‘childhood ataxia with CNS hypomyelination’ or ‘vanishing white matter disease’,Citation36 the disorder apparently results from reduced eIF2B GEF activity leading to elevated stress responses. eIF2 activity should be constitutively lower than normal and therefore have opposite effects to those mutations described above that impair the ability to phosphorylate eIF2. Unexpectedly for mutations affecting a general translation factor, this disease specifically affects glial cells within the CNS, leaving most other tissues unaffected except for the severest disease forms. It is not yet known if or how eIF5-GDI activity impacts on this disease. However perhaps cell specific variations in eIF5 expression or regulation could contribute to the cell specificity of this disease. In yeast eIF5 and eIF2 are equimolarCitation4 and form a tight complex (Kd = 23 nM)Citation9 while eIF2B is considerably less abundant than either eIF2 or eIF5 (∼8–12 fold).Citation4 The relative abundance of these factors in different mammalian primary cells and tissues is not yet clear. However variations in the abundances of each factor could provide one mechanism to affect the steady state levels of each complex and could therefore determine the degree of regulation possible. In addition, both yeast and mammalian eIF5 have been shown to be phosphorylated by Casein Kinase II.Citation37,Citation38 While regulation by phosphorylation has been demonstrated for a number of other GDIsCitation39–Citation41 it is not yet clear if eIF5-GDI is regulated by changes in post-translational modification.
Comparing Other GDIs with eIF5
GDI proteins have been described and studied in detail for several small G proteins including RhoGDI for the Rho GTPases. Several excellent reviews provide extensive information on these proteins.Citation42–Citation44 Similar to our findings for eIF5 GDI and eIF2, Rho GDIs bind to and inhibit dissociation of GDP from inactive Rho proteins. However, in addition, they can also interact with the GTP-bound G proteins to antagonise GAP stimulated and intrinsic GTPase activity. In some reports this additional function was termed GTPase inhibitory protein activity (or GIP) to distinguish between the two GDI roles.Citation45,Citation46 For clarity we use the GIP term here. Does eIF5 also prevent premature GAP activity within the PIC? This has not yet been assessed directly and is potentially complicated to assess in vivo because eIF5 has dual GAP and GDI activities. It was long thought that eIF5 stimulates eIF2•GTP hydrolysis following AUG codon-anticodon recognition by bound tRNAiMet within the PIC. However recent experiments suggest that GTP hydrolysis to a bound GDP+Pi state can precede AUG recognition within the scanning PIC.Citation9 This latter evidence is consistent with observations that movement or release of eIF1 from the PIC is a critical signal for AUG recognition, Pi and eIF2•GDP release. If so then any eIF5-GIP function with eIF2•GTP within the PIC should not be critical for general protein synthesis. This is supported by our genetic evidence as we found that yeast eIF5 mutants that lack GDI function (W391F and LR7A) can support growth at normal rates, implying no major defect in essential eIF5 functions. In contrast the GAP mutant (R15M) is lethal.Citation5 Thus in contrast to RhoGDI and other GDIs for small G proteins, it seems unlikely that any eIF5-GIP function is critical for preventing premature eIF2•GTP hydrolysisat least in yeast growing optimally. Instead available evidence for the role of eIF1 in promoting an open scanning competent ribosome conformation, the structural similarity between eIF1 and the eIF5-GAP domain and the timing of eIF1 dissociation on AUG codon recognition are consistent with eIF1 performing the role of a GIP for eIF2•GTP.Citation47–Citation49
The X-ray co-structure of RhoGDI with Cdc42 revealed that Asp45 is a conserved critical GDI residue as it binds to Cdc42 Switch I and in concert with additional contacts with Switch II stabilises GDP and Mg2+ binding.Citation50 In eIF5 Asp220 is the first invariant residue in the conserved DWEAR motif necessary for its GDI (). Further work will be necessary to assess if this residue is critical for eIF5 GDI. However, unlike RhoGDI, eIF5 is a bi-functional GAP/GDI protein that is more reminiscent of the dual activities demonstrated by the GPCR heterotrimeric G-protein regulators RGS12 and 14. Similar to eIF5, each function can be localised to separate polypeptide regions, with the N-terminal RGS domain providing GAP activity and a C-terminal ‘GoLoco’ motif necessary for GDI function on Gαi. GoLoco (18–20 aa) and DWEAR motifs (20 aa) are similar sizes and both contain conserved charged residues (). Structural analysis showed GoLoco to be helical51 and to interact with the flexible Switch regions I and II of its G protein partner Gαi. Unlike RhoGDI, the GoLoco motif has a conserved acidic-glutamine-Arginine triadCitation52 that positions the Arg side chain directly into the nucleotide pocket stabilizing the GDP phosphates.Citation51 This is reminiscent of the Arginine finger used by GAP proteins to stimulate GTPase activity suggesting a similar mechanism for these G-protein effectors. Whether the highly conserved Arginine within the DWEAR motif might also function in such a manner is not known.
In summary, the identification of a new GDI function for eIF5 suggests that the translation initiation pathway and its control are more complex that previously thought and that there are even more parallels between translation factor control and those of Small and heterotrimeric G proteins. These findings suggest several new questions that can hopefully be addressed experimentally in the near future. Further work investigating eIF5 regulation may establish additional layers of complexity for eIF2B regulation or identify other translational regulatory mechanisms for responding to cellular stress.
Figures and Tables
Figure 1 GAP and GDI functions of eIF5 during translation initiation. (A) The eIF2 G-protein cycle is central to translation initiation. (B) Domain structure of eIF5 and related functions. Relevant mutations and functional impacts described in the text are indicated. Structural cartoons were created with PyMol (http://www.pymol.org/) using PDB IDs 2E9H and 2FUL. (C) The GoLoco and eIF5 DWEAR motifs. Alignments were taken from Pfam (http://pfam.sanger.ac.uk/) and motifs logos were generated using WebLogo (http://weblogo.berkeley.edu/).
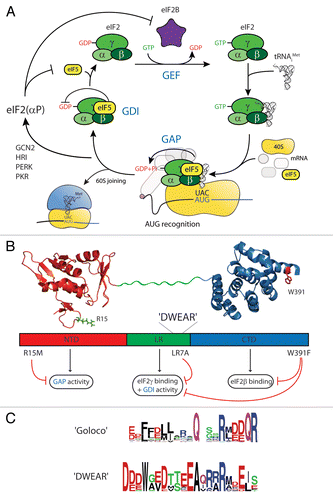
Acknowledgements
This work and M.D.J. were supported by grants BB/E002005/1 and BB/H010599/1 from the BBSRC UK to G.D.P.
Extra View to: Jennings MD, Pavitt GD. eIF5 has GDI activity necessary for translational control by eIF2 phosphorylation. Nature 2010; 465:378 - 381; PMID: 20485439; http://dx.doi.org/10.1038/nature09003
References
- Lorsch JR, Dever TE. Molecular view of 43S complex formation and start site selection in eukaryotic translation initiation. J Biol Chem 2010; 285:21203 - 21207
- Jackson RJ, Hellen CU, Pestova TV. The mechanism of eukaryotic translation initiation and principles of its regulation. Nat Rev Mol Cell Biol 2010; 11:113 - 127
- Unbehaun A, Borukhov SI, Hellen CU, Pestova TV. Release of initiation factors from 48S complexes during ribosomal subunit joining and the link between establishment of codon-anticodon base-pairing and hydrolysis of eIF2-bound GTP. Genes Dev 2004; 18:3078 - 3093
- Singh CR, Udagawa T, Lee B, Wassink S, He H, Yamamoto Y, et al. Change in nutritional status modulates the abundance of critical pre-initiation intermediate complexes during translation initiation in vivo. J Mol Biol 2007; 370:315 - 330
- Jennings MD, Pavitt GD. eIF5 has GDI activity necessary for translational control by eIF2 phosphorylation. Nature 2010; 465:378 - 381
- Pavitt GD. eIF2B, a mediator of general and genespecific translational control. Biochem Soc Trans 2005; 33:1487 - 1492
- Schmitt E, Naveau M, Mechulam Y. Eukaryotic and archaeal translation initiation factor 2: a heterotrimeric tRNA carrier. FEBS Lett 2010; 584:405 - 412
- Hinnebusch AG. Translational regulation of GCN4 and the general amino acid control of yeast. Annu Rev Microbiol 2005; 59:407 - 450
- Algire MA, Maag D, Lorsch JR. Pi release from eIF2, not GTP hydrolysis, is the step controlled by startsite selection during eukaryotic translation initiation. Mol Cell 2005; 20:251 - 262
- Singh CR, Lee B, Udagawa T, Mohammad-Qureshi SS, Yamamoto Y, Pavitt GD, et al. An eIF5/eIF2 complex antagonizes guanine nucleotide exchange by eIF2B during translation initiation. EMBO J 2006; 25:4537 - 4546
- Scheffzek K, Ahmadian MR, Kabsch W, Wiesmuller L, Lautwein A, Schmitz F, et al. The Ras-RasGAP complex: structural basis for GTPase activation and its loss in oncogenic Ras mutants. Science 1997; 277:333 - 338
- Paulin FE, Campbell LE, O'Brien K, Loughlin J, Proud CG. Eukaryotic translation initiation factor 5 (eIF5) acts as a classical GTPase-activator protein. Curr Biol 2001; 11:55 - 59
- Rittinger K, Walker PA, Eccleston JF, Smerdon SJ, Gamblin SJ. Structure at 1.65 Å of RhoA and its GTPase-activating protein in complex with a transition-state analogue. Nature 1997; 389:758 - 762
- Asano K, Clayton J, Shalev A, Hinnebusch AG. A multifactor complex of eukaryotic initiation factors, eIF1, eIF2, eIF3, eIF5 and initiator tRNA(Met) is an important translation initiation intermediate in vivo. Genes Dev 2000; 14:2534 - 2546
- Yamamoto Y, Singh CR, Marintchev A, Hall NS, Hannig EM, Wagner G, et al. The eukaryotic initiation factor (eIF) 5 HEAT domain mediates multifactor assembly and scanning with distinct interfaces to eIF1, eIF2, eIF3 and eIF4G. Proc Natl Acad Sci USA 2005; 102:16164 - 16169
- Harding HP, Zeng H, Zhang Y, Jungries R, Chung P, Plesken H, et al. Diabetes mellitus and exocrine pancreatic dysfunction in perk−/− mice reveals a role for translational control in secretory cell survival. Mol Cell 2001; 7:1153 - 1163
- Costa-Mattioli M, Gobert D, Harding H, Herdy B, Azzi M, Bruno M, et al. Translational control of hippocampal synaptic plasticity and memory by the eIF2alpha kinase GCN2. Nature 2005; 436:1166 - 1173
- Hao S, Sharp JW, Ross-Inta CM, McDaniel BJ, Anthony TG, Wek RC, et al. Uncharged tRNA and sensing of amino acid deficiency in mammalian piriform cortex. Science 2005; 307:1776 - 1778
- Scheuner D, Vander Mierde D, Song B, Flamez D, Creemers JW, Tsukamoto K, et al. Control of mRNA translation preserves endoplasmic reticulum function in beta cells and maintains glucose homeostasis. Nat Med 2005; 11:757 - 764
- Garcia MA, Meurs EF, Esteban M. The dsRNA protein kinase PKR: virus and cell control. Biochimie 2007; 89:799 - 811
- Pavitt GD, Ramaiah KVA, Kimball SR, Hinnebusch AG. eIF2 independently binds two distinct eIF2B subcomplexes that catalyze and regulate guaninenucleotide exchange. Gene Dev 1998; 12:514 - 526
- Wang S, Raven JF, Baltzis D, Kazemi S, Brunet DV, Hatzoglou M, et al. The catalytic activity of the eukaryotic initiation factor-2alpha kinase PKR is required to negatively regulate Stat1 and Stat3 via activation of the T-cell protein-tyrosine phosphatase. J Biol Chem 2006; 281:9439 - 9449
- Pavitt GD, Yang W, Hinnebusch AG. Homologous segments in three subunits of the guanine nucleotide exchange factor eIF2B mediate translational regulation by phosphorylation of eIF2. Mol Cell Biol 1997; 17:1298 - 1313
- Kimball SR, Fabian JR, Pavitt GD, Hinnebusch AG, Jefferson LS. Regulation of guanine nucleotide exchange through phosphorylation of eukaryotic initiation factor eIF2alpha. Role of the alpha- and deltasubunits of eIF2B. J Biol Chem 1998; 273:12841 - 12845
- Krishnamoorthy T, Pavitt GD, Zhang F, Dever TE, Hinnebusch AG. Tight binding of the phosphorylated alpha subunit of initiation factor 2 (eIF2alpha) to the regulatory subunits of guanine nucleotide exchange factor eIF2B is required for inhibition of translation initiation. Mol Cell Biol 2001; 21:5018 - 5030
- Hiyama TB, Ito T, Imataka H, Yokoyama S. Crystal structure of the alpha subunit of human translation initiation factor 2B. J Mol Biol 2009; 392:937 - 951
- Dev K, Santangelo TJ, Rothenburg S, Neculai D, Dey M, Sicheri F, et al. Archaeal aIF2B interacts with eukaryotic translation initiation factors eIF2alpha and eIF2Balpha: Implications for aIF2B function and eIF2B regulation. J Mol Biol 2009; 392:701 - 722
- Boesen T, Mohammad SS, Pavitt GD, Andersen GR. Structure of the catalytic fragment of translation initiation factor 2B and identification of a critically important catalytic residue. J Biol Chem 2004; 279:10584 - 10592
- Bieniossek C, Schutz P, Bumann M, Limacher A, Uson I, Baumann U. The crystal structure of the carboxy-terminal domain of human translation initiation factor eIF5. J Mol Biol 2006; 360:457 - 465
- Asano K, Krishnamoorthy T, Phan L, Pavitt GD, Hinnebusch AG. Conserved bipartite motifs in yeast eIF5 and eIF2B epsilon, GTPase-activating and GDP-GTP exchange factors in translation initiation, mediate binding to their common substrate eIF2. EMBO Journal 1999; 18:1673 - 1688
- Alone PV, Dever TE. Direct binding of translation initiation factor eIF2gamma-G domain to its GTPase-activating and GDP-GTP exchange factors eIF5 and eIF2B epsilon. J Biol Chem 2006; 281:12636 - 12644
- Mohammad-Qureshi SS, Haddad R, Palmer KS, Richardson JP, Gomez E, Pavitt GD. Purification of FLAG-tagged eukaryotic initiation factor 2B complexes, subcomplexes and fragments from Saccharomyces cerevisiae. Methods Enzymol 2007; 431:1 - 13
- Delepine M, Nicolino M, Barrett T, Golamaully M, Lathrop GM, Julier C. EIF2AK3, encoding translation initiation factor 2-alpha kinase 3, is mutated in patients with Wolcott-Rallison syndrome. Nat Genet 2000; 25:406 - 409
- Maurin AC, Jousse C, Averous J, Parry L, Bruhat A, Cherasse Y, et al. The GCN2 kinase biases feeding behavior to maintain amino acid homeostasis in omnivores. Cell Metab 2005; 1:273 - 277
- Costa-Mattioli M, Gobert D, Stern E, Gamache K, Colina R, Cuello C, et al. eIF2alpha phosphorylation bidirectionally regulates the switch from short- to long-term synaptic plasticity and memory. Cell 2007; 129:195 - 206
- Pavitt GD, Proud CG. Protein synthesis and its control in neuronal cells with a focus on vanishing white matter disease. Biochem Soc Trans 2009; 37:1298 - 1310
- Maiti T, Bandyopadhyay A, Maitra U. Casein kinase II phosphorylates translation initiation factor 5 (eIF5) in Saccharomyces cerevisiae. Yeast 2003; 20:97 - 108
- Majumdar R, Bandyopadhyay A, Deng H, Maitra U. Phosphorylation of mammalian translation initiation factor 5 (eIF5) in vitro and in vivo. Nucleic Acids Res 2002; 30:1154 - 1162
- Knezevic N, Roy A, Timblin B, Konstantoulaki M, Sharma T, Malik AB, et al. GDI-1 phosphorylation switch at serine 96 induces RhoA activation and increased endothelial permeability. Mol Cell Biol 2007; 27:6323 - 6333
- Hollinger S, Ramineni S, Hepler JR. Phosphorylation of RGS14 by protein kinase A potentiates its activity toward Galphai. Biochemistry 2003; 42:811 - 819
- Bourmeyster N, Vignais PV. Phosphorylation of Rho GDI stabilizes the Rho A-Rho GDI complex in neutrophil cytosol. Biochem Biophys Res Commun 1996; 218:54 - 60
- Dovas A, Couchman JR. RhoGDI: multiple functions in the regulation of Rho family GTPase activities. Biochem J 2005; 390:1 - 9
- DerMardirossian C, Bokoch GM. GDIs: central regulatory molecules in Rho GTPase activation. Trends Cell Biol 2005; 15:356 - 363
- Dransart E, Olofsson B, Cherfils J. RhoGDIs revisited: novel roles in Rho regulation. Traffic 2005; 6:957 - 966
- Hart MJ, Maru Y, Leonard D, Witte ON, Evans T, Cerione RA. A GDP dissociation inhibitor that serves as a GTPase inhibitor for the Ras-like protein CDC42Hs. Science 1992; 258:812 - 815
- Kishida S, Shirataki H, Sasaki T, Kato M, Kaibuchi K, Takai Y. Rab3A GTPase-activating protein-inhibiting activity of Rabphilin-3A, a putative Rab3A target protein. J Biol Chem 1993; 268:22259 - 22261
- Nanda JS, Cheung YN, Takacs JE, Martin-Marcos P, Saini AK, Hinnebusch AG, et al. eIF1 controls multiple steps in start codon recognition during eukaryotic translation initiation. J Mol Biol 2009; 394:268 - 285
- Passmore LA, Schmeing TM, Maag D, Applefield DJ, Acker MG, Algire MA, et al. The eukaryotic translation initiation factors eIF1 and eIF1A induce an open conformation of the 40S ribosome. Mol Cell 2007; 26:41 - 50
- Cheung YN, Maag D, Mitchell SF, Fekete CA, Algire MA, Takacs JE, et al. Dissociation of eIF1 from the 40S ribosomal subunit is a key step in start codon selection in vivo. Genes Dev 2007; 21:1217 - 1230
- Hoffman GR, Nassar N, Cerione RA. Structure of the Rho family GTP-binding protein Cdc42 in complex with the multifunctional regulator RhoGDI. Cell 2000; 100:345 - 356
- Kimple RJ, Kimple ME, Betts L, Sondek J, Siderovski DP. Structural determinants for GoLoco-induced inhibition of nucleotide release by Galpha subunits. Nature 2002; 416:878 - 881
- Willard FS, Kimple RJ, Siderovski DP. Return of the GDI: the GoLoco motif in cell division. Annu Rev Biochem 2004; 73:925 - 51