Abstract
Plants possess a single subfamily of Rho GTPases, ROP, which does usual things as do Rho-family GTPases in animal and fungal systems, namely participating in the spatial control of cellular processes by signaling to the cytoskeleton and vesicular trafficking. As one would expect, ROPs are modulated by conserved regulators such as DHR2-type GEFs, RhoGAPs, and Rho GDIs. What is surprising is that plants have invented new regulators such as PRONE-type GEFs (known as RopGEFs) and effectors such as RICs and ICRs/RIPs in the regulation of the cytoskeleton and vesicular trafficking. This review will discuss recent work on characterizing ROP regulators and effectors as well as addressing why and how a mixture of conserved and novel Rho signaling mechanisms is utilized to modulate fundamental cellular processes such as cytoskeletal dynamics/reorganization and vesicular trafficking.
Figures and Tables
Figure 1 Phylogenetic relationships of Rho-family small GTPases. A unrooted phylogenetic tree for representative members of the Rho family of small GTPases from different kingdoms. Representative members of ROP proteins are included, and only some members of Rho, CDC42 and Rac subfamilies are shown.
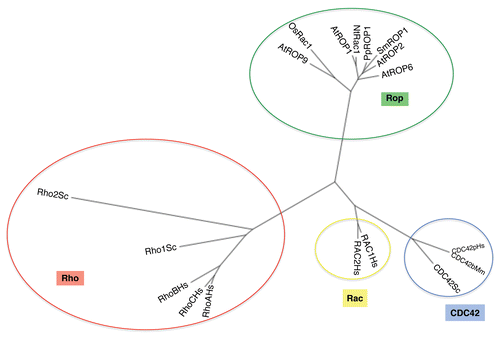
Figure 2 ROP signaling pathways in pollen tube and pavement cells. (A) ROP1 is locally activated at the apex of pollen tube PM. ROP1 activates downstream effector RIC4 that can promote F-actin assembly. ROP1 also activates another effector RIC3 that promotes Actin disassembly through regulating calcium concentration. These two pathways coordinately regulate actin dynamic that is essential for exocytosis at the sub-apical region of growing pollen tubes. REN1, a RhoGAP in plant, is translocated to the sub-apical region by exocytosis that negatively regulate ROP1 activity. (B) Counteraction of RIC3 and RIC4 in pollen tubes. All tubes are expressing pLat52::mTalin that marks actin, with co-expression of indicated RICs, and incubated with indicated amount of Ca concentration. Note that actin cable are protruding at the extreme tip of tubes in RIC3 expressing tube, while meshwork of actin covers the tip of RIC4 expressing tubes. Depolarized growth and abnormal actin organization are suppressed by coexpressing RIC3 and RIC4. Increase of Ca concentration also suppresses abnormal phenotypes induced by RIC4 expression. Images are reproduced from Gu et al.Citation13 (C) In leaf pavement cells that contain interdigitated lobes and indentations, ROP2 is locally activated at the apex of lobes by auxin through ABP1, which leads to the activation of RIC4 at lobe sites that promotes accumulation of F-Acin, which is essential for lobe outgrowth. Local active ROP2 also promotes PIN1 polar localization to apical PM of lobe. And PIN1-exported auxin in turn activates ROP2 at the cell surface to initiate a positive feedback loop which is essential for continuous outgrowth of lobe. PIN1-exported auxin at the lobe sites can diffuse across the cell wall to activate ROP6 pathway at adjacent cell. ROP6 activate RIC1 that can bind with MTs and regulate MT organization to restrict growth in this region. (D) Polar localization of ROP2, ROP6 and PIN1 in pavement cells. GFP-ROP6 signal is stronger in indenting region while GFP-ROP2 is stronger in lobe tip in adjacent lobes and indentations (arrows). Images are reproduced from Fu et al.Citation12 PIN1 localizes to tip of lobes in WT cells (arrows). Polar localization of PIN1 is lost and cytoplsmic signal is increased in rop2 RNAi rop4-1 cells (arrowheads). Images are reproduced from Xu et al.Citation68
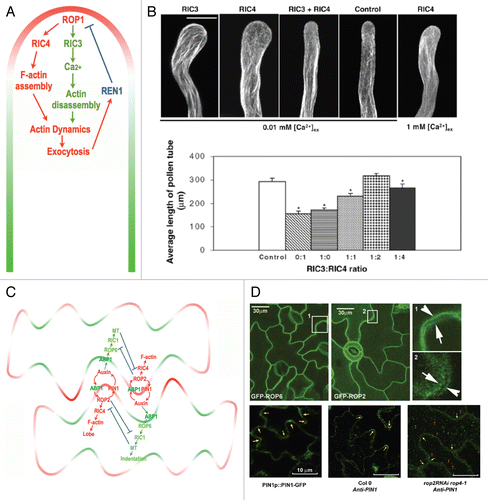
Figure 3 Comparison of CRIB motifs from CRIB-containing proteins found in plants and other kingdoms. Comparison of CRIB motifs of RIC proteins and other CRIB motif containing proteins. ROPGAPs from Arabidopsis as well as CDC42/Rac effector proteins are included. Note the high conservation of RIC proteins.
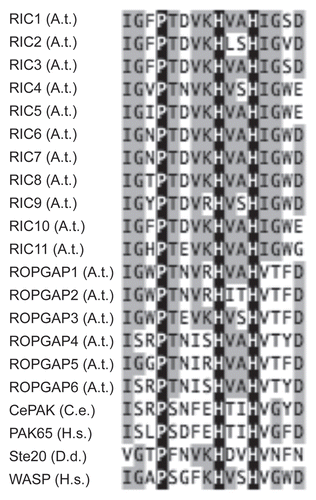
Figure 4 Novel ROP-interactive motif found in the C-terminal region of ICR/RIP proteins from Arabidopsis. Comparison of C-terminal conserved region in ICR/RIP proteins from Arabidopsis. Highly conserved QWRKAA motif required for ROP bining are highlighted.
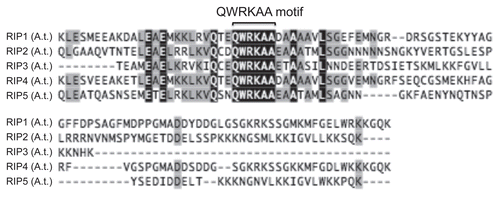
Figure 5 Rho mediated regulation of exocyst complex in different kingdoms. Models describing regulation of exocyst complex by Rho proteins in different kingdoms. Note the difference in subunit or adaptor/scaffolding protein in mediating Rho regulation.
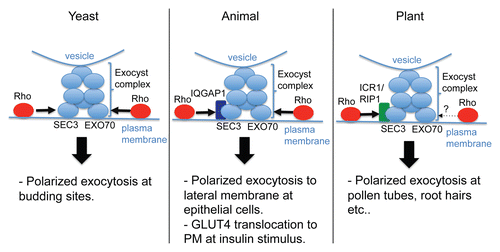
Figure 6 A working model for the polarization of PIN1 by ROP-mediated local suppression of endocytosis and polarized recycling. A working model describing PIN1 polarization achieved by ROP2-mediated regulation of both endocytosis and recycling. ROP2 activated by auxin is speculated to suppress endocytosis of PIN1 by unknown mechanisms. Active ROP2 presumably promotes recycling of PIN1 through the promotion of the ICR1/RIP1-mediated exocyst pathway. As a consequence, PIN1 accumulates at the side where ROP2 is activated, and transport auxin out of cell there. This will further increase auxin levels at the side, and thus a positive feedback loop is formed.
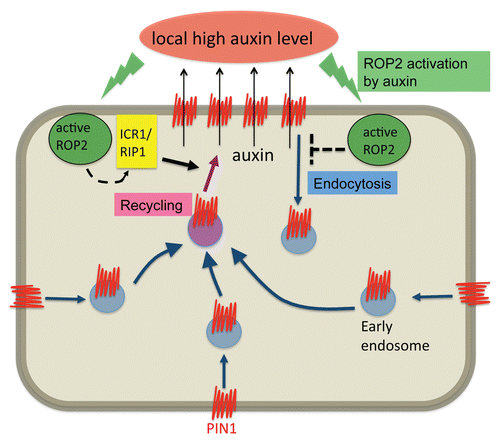
Figure 7 Schematic structures for two types of RhoGAPs in Arabidopsis. The AtRopGAP family that has 6 family members contains a CRIB domain for binding with RhoGTPase and a conserved RhoGAP domain. AtRENs family contains an N-terminal PH domain and a RhoGAP domain, and two coiled-coil (CC) domains at the C-terminus.

Table 1 List of ROP effectors