Abstract
One of the goals of understanding cytokinesis is to uncover the molecular regulation of the cellular mechanical properties that drive cell shape change. Such regulatory pathways are likely to be used at multiple stages of a cell’s life, but are highly featured during cell division. Recently, we demonstrated that 14-3-3 (encoded by a single gene in the social amoeba Dictyostelium discoideum) serves to integrate key cytoskeletal components—microtubules, Rac and myosin II—to control cell mechanics and cytokinesis. As 14-3-3 proteins are frequently altered in a variety of human tumors, we extend these observations to suggest possible additional roles for how 14-3-3 proteins may contribute to tumorigenesis.
Introduction
Cytokinesis is the process by which a mother cell divides into two daughters cells. Inherently mechanical, this process depends on the integration of the entire cytoskeletal network as multiple processes must be coordinated in space and time.Citation1 Traditionally, the mitotic spindle, the network of microtubules that separates the chromosomes, has been considered the primary source of the regulatory cues that coordinate events at the cell cortex.Citation2 While trimeric G-proteins and regulators of Rho small GTPases have been implicated in a number of systems,Citation2,Citation3 several holes and in some cases inconsistencies exist in our understanding of how the mitotic spindle regulates the cortical remodeling that drives cytokinesis. To begin to address this, we used a chemical genetic approach to identify suppressors of nocodazole (a microtubule depolymerizer), and one of the major suppressors identified was overexpression of 14-3-3.Citation4 14-3-3 proteins are acidic ∼30 kDa proteins, which dimerize to form a protein-binding cleft that often, but not always, binds phosphorylated target proteins.Citation5–Citation9 14-3-3 proteins are known to interact with several hundred proteins involved in a wide array of cellular processes such as apoptosis, cell cycle progression and cytokinesis so their involvement in cell division is not unanticipated.Citation6,Citation10,Citation11
14-3-3 and RacE
In Dictyostelium, 14-3-3 is enriched in the cell cortex in a manner dependent on the RacE small GTPase.Citation4 A portion of 14-3-3 also fractionates into an insoluble pool that depends on RacE and GTP, suggesting that active RacE is essential for the cortical binding. 14-3-3 overexpression was able to rescue the severe cortical mechanical and cytokinesis defects of racE null mutants, solidly implicating a RacE-14-3-3 pathway. The RacE isoform in Dictyostelium is a Rac-family small GTPase that is a little more distantly related to canonical Rac members of mammals and is found to be somewhat related to Rac and Rho subfamilies.Citation12 Dictyostelium contain several typical Rac isoforms, which interact with p21-binding domains (PBDs) found in proteins such as pak proteins (p21-activated kinases).Citation13 RacE does not interact with PBDs,Citation14 and it is unclear what the relevant direct downstream effectors of RacE are. However, the dependence of 14-3-3 on RacE for cortical distribution and its solubility along with the ability of 14-3-3 overexpression to rescue several racE phenotypes indicates that 14-3-3 interacts closely with and downstream of RacE, though a direct interaction has not yet been detected ().
14-3-3 and Myosin II
Though most organisms have two or more 14-3-3 isoforms (mammals have seven), the Dictyostelium genome has just one. It is not surprising then that 14-3-3 is an essential gene in this system.Citation4 However, partial silencing of 14-3-3 to 30% of normal levels using a hairpin plasmid (14-3-3 hp) led to cell cultures that produced multinucleated cells, a hallmark of cytokinesis failure. The dynamics of cleavage furrow contractility and the morphology of the 14-3-3 hp cells as their shaped evolved was highly reminiscent of the very stereotypical myosin II null cytokinesis. Subsequent proteomics analysis revealed that myosin II heavy chain is a major binding partner for 14-3-3. Follow-up analysis confirmed that 14-3-3 does indeed bind wild type myosin II though not the phosphomimic 3x Asp mutant myosin II, which is myosin II bipolar thick filament (BTF) assembly incompetent.
Three well characterized consensus binding motifs are commonly found in 14-3-3-binding partners.Citation9 These include two phosphorylation-dependent binding motifs (motif 1 and motif 2) and a nonphosphorylation-dependent motif (motif 3). However, other types of interaction sites have also been observed. Interestingly, Dictyostelium myosin II has no predicted consensus motif 1 binding sites, only motifs 2 and 3, most of which are found in the coiled coil tail.Citation4 All of the predicted sites near the 3x Asp mutant sites are of the motif 3 class, suggesting that the 14-3-3-myosin binding interactions may not be directly dependent on phosphorylation. The inability of 14-3-3 to associate with 3x Asp myosin II may be because 3x Asp is severely impaired in its ability to assemble into bipolar thick filamentsCitation15 or its cellular distribution is shifted from the cortex to the cytoplasm.Citation16 Alternatively, subtle structural changes in the myosin II tail upon phosphorylation might reduce the affinity of the 14-3-3-myosin II interaction, thereby reducing the association of 14-3-3 with the phosphomimic 3x Asp myosin II.Citation17
Nevertheless, assessment of the myosin II distribution in cells with altered 14-3-3 levels demonstrated that 14-3-3 promotes myosin II BTF remodeling (): depletion of 14-3-3 led to decreased myosin II mobile fraction and clumping, whereas 14-3-3 overexpression led to an increased mobile fraction (). Mobility here refers to the mobile and immobile fractions of myosin II as assessed by fluorescence recovery after photobleaching. Consistent with the RacE dependency for 14-3-3 function, myosin II also shows aggregation in racE null cells. In in vitro myosin II BTF assembly assays, the addition of 14-3-3 reduced the amount of assembled myosin II. The combination of in vivo and in vitro data suggests that 14-3-3 might reduce the affinity or increase accessibility of the myosin II monomers (the functional monomer is the hexamer of two heavy chains, two essential light chains and two regulatory light chains) for the BTF, which in vivo leads to alterations in myosin II mobility that correlates with 14-3-3 levels.
The direct biochemical interactions between myosin II and 14-3-3 suggest an intriguing new twist in myosin II regulation. Much of the known regulation of myosin II BTF assembly occurs through heavy chain phosphorylation and dephosphorylation.Citation15 Clearly, this level of regulation is critical for maintaining a free pool of myosin monomers so that myosin II BTFs may be remodeled during dynamic processes such as cytokinesis and cell motility. Undoubtedly, this represents just part of the story. It remains unknown how myosin II BTF nucleation is controlled and what the cortical receptor/anchor(s) are for myosin II BTFs in most contexts (here, we define the cortex as the composite of cortical actin network and the overlying plasma membrane). Where there is evidence for a receptor, such as in the case of anillin (in metazoans), the structure-function studies raise questions about whether anillin is really an anchor or alternatively one of several anchors.Citation17–Citation19 In a recent study, α4β1 integrins may also provide one possible cortical anchor for myosin IIA in mammalian cells,Citation20 demonstrating that multiple myosin II cortical receptors likely exist. As 14-3-3 is cortically enriched, one possibility is that it forms part of one such cortical receptor for myosin II. By having many receptors, the myosin II BTFs may be nucleated at several sites, yielding smaller BTFs (), which in turn may also lead to greater mobility. The recovery kinetics (τrec) largely reflect the release of myosin II monomers from the BTFs, which remain unchanged between the different levels of 14-3-3 expression (τrec = 5 s for WT, 14-3-3 hp and 14-3-3-overexpressing cells) (). By helping set the steady state mobility of the myosin II monomers and by maintaining a uniform distribution of myosin II BTFs, 14-3-3 contributes to cortical mechanics and cell shape changes such as in cytokinesis (). Depletion of 14-3-3 leads to a significant reduction in cortical tension while its overexpression could rescue the mechanical defects associated with racE deletion or with nocodazole treatment. However, 14-3-3 overexpression failed to alter the cortical mechanics of myosin II null cells further illustrating that 14-3-3 works through myosin II.
14-3-3 and Microtubules
Since we found 14-3-3 as a suppressor of nocodazole, we also analyzed the microtubule-network structure. By mapping out and measuring the lengths of several tens of microtubules, we found that 14-3-3 helps establish the steady state average microtubule length (). Because 14-3-3 was cortically enriched, we paid particular attention to the microtubulecortex contact lengths. Upon measuring around 50,000 microtubule-cortex interaction events, we concluded that 14-3-3 does contribute to the microtubulecortex interactions but that the nature of these interactions is complex. Both loss-of-function and overexpression of 14-3-3 led to a reduction in the length of microtubule-cortex interactions though the overall lifetime of the interactions was unchanged (τ = 5 s). These observations are of added interest as previous particletracking studies revealed that the cell cortex has super-diffusive activities that are myosin II-dependent.Citation21 Yet, in a myosin II null mutant cell-line, these activities could be recovered by depleting the actin crosslinking protein dynacortin.Citation21,Citation22 These observations argue then that myosin II potentiates but does not drive these superdiffusive activities. Implicated through a process of elimination but not formally proven, microtubules were suggested to be likely drivers of the super-diffusive activities. Putting it all together, one might speculate that 14-3-3 helps anchor myosin II and microtubules at the cortex and these assemblies cooperatively modulate the super-diffusive interacting network of the cortex. One could imagine then that 14-3-3 helps link several components of the cortical myosin II-microtubule-RacE network. As an example, focal adhesions can stabilize microtubules,Citation23 and integrins have been recently shown to bind to myosin IIACitation20 and 14-3-3 proteinsCitation24–Citation26 in mammalian cells.Citation26 Thus, such a complex network could allow cross-talk from microtubules to myosin II, and 14-3-3 is poised to provide part of the critical link. Interestingly, 14-3-3 binding to β2 integrin in human T cells also leads to activation of Rac1 and Cdc42 small GTPases though how 14-3-3 leads to Rac1 and Cdc42 activation in this context is not yet fully understood.Citation24 This sort of conceptual network then could interact cooperatively to facilitate the remodeling of the cortex to promote cell shape changes and to control cortex mechanics.
14-3-3, Cell Mechanics and Cytokinesis
One of the more fascinating aspects of cytokinesis is how molecular-scale dynamics lead to cellular-scale mechanical properties that ultimately drive cell shape change.Citation27 These mechanical properties include cortical and cytoplasmic viscoelasticity, cortical tension and actively generated stresses (force/area). The list of proteins that contribute to cell mechanics include myosin II, actin polymers, actin crosslinkers, microtubules and their regulatory proteins, such as Rac small GTPases and 14-3-3.Citation4 Ultimately, the mechanical parameters generated by these proteins specify the geometry (i.e., cell shape and morphology) and shape change kinetics (e.g., rate of furrow constriction). The properties work together to lead to other cellular-scale features such as Laplace-like pressures that result from the cortical tension and the membrane curvature. All of these factors collaborate to squeeze the cytoplasm from the mid-zone, pinching the cell into two daughter cells.
Possible Implications for Cancer Biology
While Dictyostelium cells do not suffer from cancer (at least from our perspective), several possible medical implications are suggested by this body of work. Alterations of 14-3-3 expression levels (both up and down) are associated with a broad variety of invasive tumor states and many tumor cell-types have alterations in cell mechanics.Citation28–Citation30 Furthermore, it is becoming increasingly appreciated that the fidelity of cytokinesis is crucial for maintaining chromosome stability.Citation31 While much effort has rightly gone into understanding the role of 14-3-3 in cell cycle control, we suggest that by regulating myosin II dynamics, 14-3-3 may play a very direct role in the myosin II contractile networks that establish the mechanical properties (cortical tension and viscoelasticity) and that drive cytokinesis contractility. Perturbations of myosin II-mediated function would promote cell transformation, tumor progression and metastasis. Indeed, myosin II heavy chain appears on the list of potential 14-3-3σ interactors from proteomics studies,Citation32 and 14-3-3 has been implicated in regulating myosin light chain phosphatase as well as contractile ring disassembly in mammalian cells,Citation33,Citation34 supporting these possibilities. 14-3-3 also plays other roles during mitosis and cytokinesis such as in the regulation of the centralspindlin complex,Citation35 providing yet another means by which it may impact cytokinesis fidelity. An intriguing recent study suggests that increasing myosin II-mediated contractility enhances cytokinesis fidelity and chromosomal stability, whereas inhibiting myosin II contractility reduces cytokinesis fidelity, promoting chromosomal instability.Citation36 Thus, many of the pieces for how cytokinesis fidelity, chromosomal instability, myosin II contractility and human cancer genes work together to promote normal and pathological cell division have been observed.
In the context of cell mechanics, one mammalian cell study has attempted to directly test the impact of overexpressing 14-3-3ζ on cell stiffness and cell transforming potential of mammary epithelial cells (MEC).Citation37 Overexpression of 14-3-3ζ failed to have a major impact on the mechanics of these cells. This may be analogous to our observations that 14-3-3 overexpression only measurably changed the mechanics of Dictyostelium cells if the cells were challenged in some way (racE deletion or nocodazole treatment). From our studies,Citation27,Citation38 we find that some proteins show saturable effects on mechanics so that overexpression fails to have an impact unless the system has been perturbed in some other way, while depletion leads to significant softening of the cell (as in the case of 14-3-3). In contrast, other proteins like some actin crosslinkers impact mechanics, showing an increase or reduction in stiffness and tension with overexpression or depletion, respectively. We do not yet fully understand the basis for this dichotomy. However, it would be interesting to determine whether reducing the expression levels of 14-3-3 isoforms, such as 14-3-3σ (which is downregulated in many tumor types, suggesting potential tumor suppressor activityCitation28,Citation38,Citation39), affects cell mechanics.
Critical Questions
Clearly, discerning the nature of the cortical receptor/anchors for myosin II is essential to determining how myosin II and 14-3-3 interact. Pressing questions include: do multiple myosin II cortical anchors exist, what are they, how do Rac and 14-3-3 regulate or modulate the binding to myosin II, and how do these complexes interact with microtubules. In this review, a discussion of integrins has been included as one possible scenario. However, other types of membrane proteins may be just as likely to play the role of cortex anchor. Chloride channels have been shown to bind to 14-3-3 proteins in Arabidopsis and are found in the cleavage furrow region in mammalian cells.Citation40,Citation41,Citation44 Further, myosin II can co-purify with a subset of chloride channel containing vesicles.Citation42 Perhaps a similar network of a chloride channel-family protein and 14-3-3 is involved in myosin II cleavage furrow anchoring. Overall, 14-3-3 mediates some amount of cross-talk between these critical elements of microtubules, myosin II and RacE small GTPase. Additionally, such cross-talk creates the foundation for feedback, allowing the integration of mechanical and biochemical pathways and processes.Citation27 What will be interesting to decipher ultimately is how the cross-talk in this system leads to cooperativity and robustness to ensure cytokinesis fidelity to protect against the genomic instability that comes from cytokinesis failure.
Figures and Tables
Figure 1 14-3-3 coordinates RacE, microtubules and myosin II. (A) A pathway diagram demonstrating how 14-3-3 operates downstream of RacE and microtubules (MT) but is required to maintain MT length. 14-3-3 also promotes myosin II bipolar thick filament (BTF) remodeling along the cortex (depicted by the curved line) in order to modulate cortical mechanics and cytokinesis shape change. (B) Myosin II BTF assembly occurs through a nucleation-elongation process.Citation43 The assembly incompetent form of myosin II (M0) is maintained by heavy chain phosphorylation. The assembly competent form (M) can nucleate BTFs with n monomers per BTF. These can be formed in the cytoplasm or on the cortex. Some evidence suggests that cortex anchoring may occur through some receptor R that binds to the coiled coils of the BTFs.Citation44,Citation45 (C) Alterations in 14-3-3 expression levels do not affect the recovery time (τrec = 5 s) measured by FRAP. However, the immobile fraction is increased by 14-3-3 depletion using a 14-3-3 RNAi hairpin vector (14-3-3 hp). One way to achieve this is if the myosin monomers M (depicted as rods with motor domains not shown) are able to release with similar kinetics, but if 14-3-3 helps maintain smaller BTFs (which are predicted to be exponentially distributed by sizeCitation46), then fewer monomers will be buried deep within the BTF (BTFs assemble through lateral interactions so that the BTF primarily grows by increasing in diameter rather than length). (D) 14-3-3 (along with RacE) might also form part of a cortical receptor complex (R). If the cortical receptors also help nucleate the BTFs, fewer receptors (for example by depleting 14-3-3 with 14-3-3 hp) would lead to fewer nucleation centers. This might then increase the immobile fraction as depicted in (C).
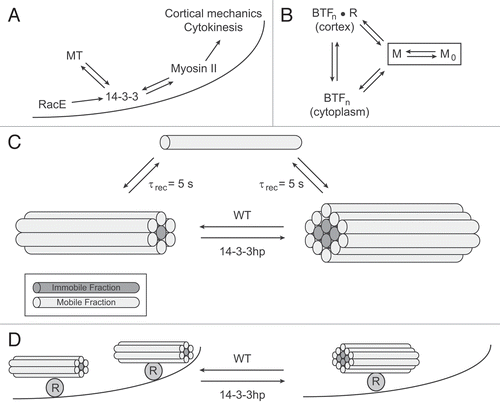
Extra View to: Zhou Q, Kee Y-S, Poirier CC, Jilenik C, Osborne J, Divi S, Surcel A, Tran ME, Eggert US, Müller-Taubenberger A, Iglesias PA, Cotter RJ, Robinson DN. 14-3-3 coordinates microtubules, Rac, and myosin II to control cell mechanics and cytokinesis. Curr Biol 2010; 20:1881 - 1889; PMID: 20951045; http://dx.doi.org/10.1016/j.cub.2010.09.048
References
- Reichl EM, Effler JC, Robinson DN. The stress and strain of cytokinesis. Trends Cell Biol 2005; 15:200 - 206
- Glotzer M. The molecular requirements for cytokinesis. Science 2005; 307:1735 - 1739
- Bringmann H, Cowan CR, Kong J, Hyman A. LET-99, GOA-1/GPA-16 and GPR-1/2 are required for aster-positioned cytokinesis. Curr Biol 2007; 17:185 - 191
- Zhou QQ, Kee YS, Poirier CC, Jelinek C, Osborne J, Divi S, et al. 14-3-3 coordinates microtubules, Rac and myosin II to control cell mechanics and cytokinesis. Curr Biol 2010; 20:1881 - 1889
- Xiao B, Smerdon SJ, Jones DH, Dodson GG, Soneji Y, Aitken A, et al. Structure of a 14-3-3 protein and implications for coordination of multiple signalling pathways. Nature 1995; 376:188 - 191
- Liu D, Bienkowska J, Petosa C, Collier RJ, Fu H, Liddington R. Crystal structure of the zeta isoform of the 14-3-3 protein. Nature 1995; 376:191 - 194
- Yaffe MB, Rittinger K, Volinia S, Caron PR, Aitken A, Leffers H, et al. The structural basis for 14-3-3: phosphopeptide binding specificity. Cell 1997; 91:961 - 971
- Rittinger K, Budman J, Xu J, Volinia S, Cantley LC, Smerdon SJ, et al. Structural analysis of 14-3-3 phosphopeptide complexes identifies a dual role for the nuclear export signal of 14-3-3 in ligand binding. Mol Cell 1999; 4:153 - 166
- Aitken A. 14-3-3 proteins: a historic overview. Semin Cancer Biol 2006; 16:162 - 172
- Jin J, Smith FD, Stark C, Wells CD, Fawcett JP, Kulkarni S, et al. Proteomic, functional and domainbased analysis of in vivo 14-3-3 binding proteins involved in cytoskeletal regulation and cellular organization. Curr Biol 2004; 14:1436 - 1450
- Pozuelo Rubio M, Geraghty KM, Wong BH, Wood NT, Campbell DG, Morrice N, et al. 14-3-3-affinity purification of over 200 human phosphoproteins reveals new links to regulation of cellular metabolism, proliferation and trafficking. Biochem J 2004; 379:395 - 408
- Vlahou G, Rivero F. Rho GTPase signaling in Dictyostelium discoideum: Insights from the genome. Eur J Cell Biol 2006; 85:947 - 959
- Eichinger L, Pachebat JA, Glockner G, Rajandream MA, Sucgang R, Berriman M, et al. The genome of the social amoeba Dictyostelium discoideum. Nature 2005; 435:43 - 57
- Park KC, Rivero F, Meili R, Lee S, Apone F, Firtel RA. Rac regulation of chemotaxis and morphogenesis in Dictyostelium. EMBO J 2004; 23:4177 - 4189
- Egelhoff TT, Lee RJ, Spudich JA. Dictyostelium myosin heavy chain phosphorylation sites regulate myosin filament assembly and localization in vivo. Cell 1993; 75:363 - 371
- Sabry JH, Moores SL, Ryan S, Zang JH, Spudich JA. Myosin heavy chain phosphorylation sites regulate myosin localization during cytokinesis in live cells. Mol Biol Cell 1997; 8:2647 - 2657
- Straight AF, Field CM, Mitchison TJ. Anillin binds nonmuscle myosin II and regulates the contractile ring. Mol Biol Cell 2005; 16:193 - 201
- Maddox AS, Lewellyn L, Desai A, Oegema K. Anillin and the septins promote asymmetric ingression of the cytokinetic furrow. Dev Cell 2007; 12:827 - 835
- Piekny AJ, Glotzer M. Anillin is a scaffold protein that links RhoA, actin and myosin during cytokinesis. Curr Biol 2008; 18:30 - 36
- Rivera Rosado LA, Horn TA, McGrath SC, Cotter RJ, Yang JT. Association between α4 integrin cytoplasmic tail and non-muscle myosin IIA regulates cell migration. J Cell Sci 2011; In press
- Girard KD, Kuo SC, Robinson DN. Dictyostelium myosin-II mechanochemistry promotes active behavior of the cortex on long time-scales. Proc Natl Acad Sci USA 2006; 103:2103 - 2108
- Robinson DN, Ocon SS, Rock RS, Spudich JA. Dynacortin is a novel actin bundling protein that localizes to dynamic actin structures. J Biol Chem 2002; 277:9088 - 9095
- Kaverina I, Rottner K, Small JV. Targeting, capture and stabilization of microtubules at early focal adhesions. J Cell Biol 1998; 142:181 - 190
- Nurmi SM, Autero M, Raunio AK, Gahmberg CG, Fagerholm SC. Phosphorylation of the LFA-1 integrin beta2-chain on Thr-758 leads to adhesion, Rac-1/Cdc42 activation and stimulation of CD69 expression in human T cells. J Biol Chem 2007; 282:968 - 975
- Takala H, Nurminen E, Nurmi SM, Aatonen M, Strandin T, Takatalo M, et al. Beta2 integrin phosphorylation on Thr758 acts as a molecular switch to regulate 14-3-3 and filamin binding. Blood 2008; 112:1853 - 1862
- Legate KR, Fassler R. Mechanisms that regulate adaptor binding to beta-integrin cytoplasmic tails. J Cell Sci 2009; 122:187 - 198
- Surcel A, Kee YS, Luo T, Robinson DN. Cytokinesis through biochemical-mechanical feedback loops. Semin Cell Dev Biol 2010; 21:866 - 873
- Hermeking H. The 14-3-3 cancer connection. Nature Rev Cancer 2003; 3:931 - 943
- Tzivion G, Gupta VS, Kaplun L, Balan V. 14-3-3 proteins as potential oncogenes. Sem Cancer Biol 2006; 16:203 - 213
- Suresh S. Biomechanics and biophysics of cancer cells. Acta Biomater 2007; 3:413 - 438
- Ganem NJ, Godinho SA, Pellman D. A mechanism linking extra centrosomes to chromosomal instability. Nature 2009; 460:278 - 282
- Wilker EW, van Vugt MA, Artim SA, Huang PH, Petersen CP, Reinhardt HC, et al. 14-3-3sigma controls mitotic translation to facilitate cytokinesis. Nature 2007; 446:329 - 332
- Koga Y, Ikebe M. A novel regulatory mechanism of myosin light chain phosphorylation via binding of 14-3-3 to myosin phosphatase. Mol Biol Cell 2008; 19:1062 - 1071
- Saurin AT, Durgan J, Cameron AJ, Faisal A, Marber MS, Parker PJ. The regulated assembly of a PKCepsilon complex controls the completion of cytokinesis. Nat Cell Biol 2008; 10:891 - 901
- Douglas ME, Davies T, Joseph N, Mishima M. Aurora B and 14-3-3 coordinately regulate clustering of centralspindlin during cytokinesis. Curr Biol 2010; 20:927 - 933
- Wu Q, Sahasrabudhe RM, Luo LZ, Lewis DW, Gollin SM, Saunders WS. Deficiency in myosin light-chain phosphorylation causes cytokinesis failure and multipolarity in cancer cells. Oncogene 2010; 29:4183 - 4193
- Baker EL, Lu J, Yu D, Bonnecaze RT, Zaman MH. Cancer Cell Stiffness: Integrated roles of three-dimensional matrix stiffness and transforming potential. Biophys J 2010; 99:2048 - 2057
- Mhawech P, Benz A, Cerato C, Greloz V, Assaly M, Desmond JC, et al. Downregulation of 14-3-3sigma in ovary, prostate and endometrial carcinomas is associated with CpG island methylation. Mod Pathol 2005; 18:340 - 348
- Lodygin D, Hermeking H. Epigenetic silencing of 14-3-3sigma in cancer. Semin Cancer Biol 2006; 16:214 - 224
- Berryman MA, Goldenring JR. CLIC4 is enriched at cell-cell junctions and colocalizes with AKAP350 at the centrosome and midbody of cultured mammalian cells. Cell Motil Cytoskeleton 2003; 56:159 - 172
- Chang IF, Curran A, Woolsey R, Quilici D, Cushman JC, Mittler R, et al. Proteomic profiling of tandem affinity purified 14-3-3 protein complexes in Arabidopsis thaliana. Proteomics 2009; 9:2967 - 2985
- Ecay TW, Conner TD, Decker ER. Nonmuscle myosin IIA copurifies with chloride channel-enriched membranes from epithelia. Biochem Biophys Res Comm 1997; 231:369 - 372
- Mahajan RK, Pardee JD. Assembly mechanism of Dictyostelium myosin II: Regulation by K+, Mg2+ and actin filaments. Biochemistry 1996; 35:15504 - 15514
- Zang JH, Spudich JA. Myosin II localization during cytokinesis occurs by a mechanism that does not require its motor domain. Proc Natl Acad Sci USA 1998; 95:13652 - 13657
- Shu S, Liu X, Korn ED. Dictyostelium and Acanthamoeba myosin II assembly domains go to the cleavage furrow of Dictyostelium myosin II-null cells. Proc Natl Acad Sci USA 2003; 100:6499 - 6504
- Ren Y, Effler JC, Norstrom M, Luo T, Firtel RA, Iglesias PA, et al. Mechanosensing through cooperative interactions between myosin II and the actin crosslinker cortexillin I. Curr Biol 2009; 19:1421 - 1428