Abstract
The slow growth rate of Mycobacterium tuberculosis hinders research progress, since estimating the bacterial numbers present in all experiments normally relies on determination of colony forming units on agar plates. M. tuberculosis colonies can take as long as four to six weeks to become visible. Whole animal imaging is an emerging technology that has broad applications in all areas of biological sciences, including monitoring infections. Imaging allows bacterial numbers to be determined in real-time for each infected animal, individually, which allows inter-animal variability to be observed and controlled for. Reporter enzyme fluorescence (REF) utilizes custom substrates that allow production of a fluorescent product after cleavage by a bacterial enzyme. In our recently published studies, we demonstrate that the enzyme β-lactamase, a naturally occurring enzyme expressed by M. tuberculosis, can be used for REF. The resulting imaging system is the first that allows non-invasive detection of natural M. tuberculosis strains directly in pulmonary infected living animals. Use of REF for M. tuberculosis infected mice allows detection of ~104 CFU in the lungs, which is very sensitive. This system also displays promise for allowing rapid evaluation of differences in virulence strains and efficacy of therapeutics and vaccines. This system could be developed into a diagnostic tool for tuberculosis through the use of REF to identify infected tissues or other diagnostic specimens.
Acknowledgements
This work was funded by grant 48523 from the Bill & Melinda Gates Foundation and grant AI47866 from the National Institutes of Health.
Figures and Tables
Figure 1 Description of the reporter enzyme fluorescence (REF) approach to imaging bacterial infections. (A) The reaction that generates RE F signal is catalyzed by a bacterial enzyme, preferably naturally produced. In this case, the bacteria is Mycobacterium tuberculosis (Mtb) and the enzyme is β-lactamase. A custom substrate is used that either is based on fluorescence resonance energy transfer (FRET) quenching of the fluorophore or uses a structure that is not fluorescent until cleavage. (B) The structure of CNIR5, one of the primary substrates used successfully for REF. The β-lactam ring indicated is cleaved by β-lactamase and separates the fluorescent dye, in this case Cy5.5, from the quencher (QSY22) that absorbs light at the Cy5.5 wavelength of emission (∼690 nm). Modifications that impact catalytic activity and specificity can include changes to any component of this structure, but those substitutions surrounding the β-lactam ring have the greatest impact. (C) Proposed model that outlines the high sensitivity of REF. The substrate (CNIR5) is cleaved to a product by intracellular Mtb. The product (Cy5.5) is retained within the host cell and builds up to very high levels until substrate is no longer available.
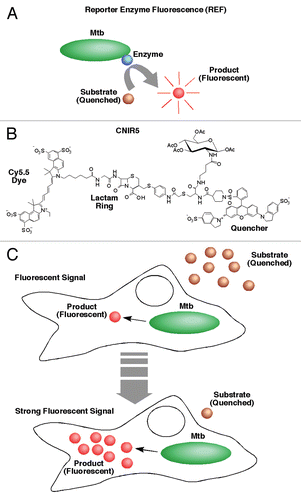
Figure 2 Observed fluorescent product from REF accumulating in host macrophages over time post-infection with mycobacteria. Host cell (J774A.1 murine macrophages) nuclei were stained with DAPI (blue), mycobacteria were expressing GFP (green) and the CNIR5 fluorescent dye is Cy5.5 (red). Note the increase in product (red) fluorescence between four and 24 h post-infection with mycobacteria. This confocal microscopy supports the model in . A pool of fluorescent product is visible within infected cells that is sufficient for fluorescence-activated cell sorting (FACS) and confocal microscopy, explaining the high levels of signal observed over time, even when animals are infected with low bacterial numbers.
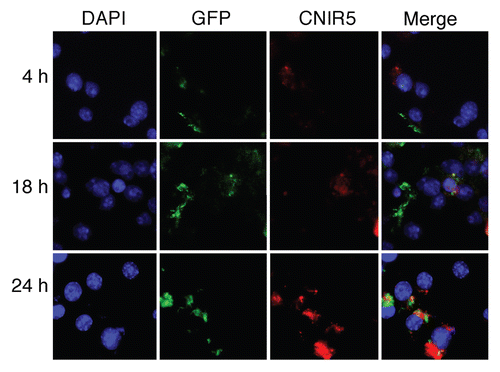
Addendum to: