Abstract
The blood-brain barrier (BBB) is a structural and functional barrier that protects the central nervous system (CNS) from invasion by blood-borne pathogens including parasites. However, some intracellular and extracellular parasites can traverse the BBB during the course of infection and cause neurological disturbances and/or damage which are at times fatal. The means by which parasites cross the BBB and how the immune system controls the parasites within the brain are still unclear. In this review we present the current understanding of the processes utilized by two human neuropathogenic parasites, Trypanosoma brucei spp and Toxoplasma gondii, to go across the BBB and consequences of CNS invasion. We also describe briefly other parasites that can invade the brain and how they interact with or circumvent the BBB. The roles played by parasite-derived and host-derived molecules during parasitic and white blood cell invasion of the brain are discussed.
Introduction
The spread of a pathogen to the brain during an infection is in general considered as a rare but serious complication of the disease. However, certain parasites have a propensity to infiltrate into the central nervous system (CNS), which may provide a so-called immune-privileged site and therefore be of advantage for survival of the invading microbe. For such parasite-host interactions certain sets of parasite-derived molecules may facilitate invasion of the parasites into the tissues, while host-derived immune response molecules are produced with the aim to inhibit their spread. A number of mechanisms have also evolved by which the immune response against a parasite may be dampened. For instance, the Th1 immune response, which is directed against intracellular pathogens, can be inhibited during infections with certain microbes in which the Th2 response, which is directed against extracellular pathogens, instead is promoted; the two arms of the immune response being mutually inhibitory.Citation1
Recent reviews have been focused on bacterial invasion across the BBB, but also covered broader aspects by which various microbes spread to the nervous system.Citation2-Citation4 In this review we will describe the mechanisms by which one extracellular parasite, Trypanosoma brucei (T. b.) may pass across the blood-brain barrier (BBB) to enter the brain parenchyma, since this event is considered to be of crucial importance for therapeutic considerations. In order to disclose any distinguishing features in the passage of this parasite, comparisons will be made other parasites and in particular with Toxoplasma gondii that can be both extra- and intra-cellular, but predominantly intracellular.
The two human pathogenic trypanosome subspecies T. b. gambiense and T. b. rhodesiense invariably cause fatal meningoencephalitis if the infections are left untreated, while the Toxoplasma gondii parasites can remain dormant for long periods of time in cysts within neurons and astrocytes, but can be reactivated if the host is immunocompromised and result in encephalitis that also is lethal if not treated.Citation5,Citation6 Before reviewing mechanisms for neuroinvasion by the pathogens, certain properties of the BBB that the parasites have to overcome to be able to enter into the brain parenchyma will be high-lighted.
In order to maintain constancy of the CNS internal environment, which is vital for neuronal function, the passage of molecules across blood vessels in the CNS is restricted and tightly regulated compared with other tissues. This is attributed to the BBBCitation7,Citation8 (), which has been described in detail by reviews referred to above. Here we will only highlight certain features of relevance for an understanding of passage of the parasites, which are the focus of this review. The cerebral endothelial cells are bound together with tight junctions, which provide a structural barrier that prevents the diffusion of molecules between the endothelial cells into the brain parenchyma, i.e., through the paracellular pathway. The cerebral endothelial cells have low levels of pinocytotic activity or transcytosis and form a functional barrier by selectively transporting only specific molecules into the brain parenchyma.Citation8,Citation9 The endothelial basement membrane enwraps pericytes, which among other things are important for regulating transcytosis across the BBB.Citation10 At the level of capillaries, where exchange of metabolites across the BBB mainly occurs, one basement membrane separates endothelial cells and astrocytes, whereas at the level of post-capillary venules, where infiltration of white blood cells (WBCs) into the brain parenchyma occurs during inflammation, two basement membranes separate the endothelial cells and the abutting astrocytic endfeet, namely the endothelial and the parenchymal basement membranes.Citation11,Citation12 The latter basement membrane is also referred to as the astrocytic basement membrane because it is produced principally by astrocytes and deposited at their endfeet.Citation13 The laminin isotype composition of these two basement membranes plays a role in infiltration of WBCs into the brain parenchyma. The endothelial basement membrane containing laminin α4 is permissive to WBC penetration, while the one with laminin α5, which in contrast to laminin α4 has a patchy distribution, is not. Penetration of WBCs across the parencyhmal basement membrane, which contains laminin α1 and α2, requires focal activation of the matrix metalloproteases (MMP) 2 and 9. These proteases cleave the dystroglycan receptors, which anchor the astrocytic end-feet to this basement membrane.Citation11 Before activation of the MMPs occur, infiltrating WBCs are trapped between the two basement membranes.Citation14
Figure 1. Crossing of the blood-brain barrier (BBB) by parasites associated with WBCs. (A) Illustration of a cerebral post-capillary vessel showing the BBB, consisting of a complex of cerebral endothelial cells and their tight junctions, basement membranes and pericytes as well as astrocytic end-feet. Note the perivascular space that is noticeable during inflammation. (B) Toxoplasma gondii crossing the BBB. (1) Induction of vascular cell adhesion molecule 1 (VCAM-1) and adhesion molecules activated leukocyte cell adhesion molecule (ALCAM) in the brain during infection might aid the monocytes infected Toxoplasma gondii tachyzoites to cross the BBB. The infection cause an increased motility of the monocytes which may facilitate their migration into the brain. Toxoplasma gondii can invade endothelial cells, however, its ability to cross the BBB as extracellular parasites in vivo is not clear. (C) The extracellular parasite Trypanosoma brucei spp and T cells cross the endothelial cell layer, the endothelial basement membrane and the parenchymal to invade the brain parenchyma. (1) TNFα and IFNα/β are released upon TLR9/MyD88-mediated activation of the innate immune response. (2) TNFα induces ICAM/VCAM on cerebral endothelial cells which allow attachment of T cells. IFNα/β induces a limited release of CXCL10 by endothelial cells and/or astrocytes, which is enough for penetration of T cells accompanied by some trypanosomes into the perivascular space. (3) Trypanosome-derived antigens taken up and expressed by macrophages could then be recognized by sensitized T cells to induce IFNγ, which augment the process through (4) induction of CXCL10 production by astrocytes and (5) molecules that open the parenchymal basement membrane for spread of both T cells and trypanosomes into the brain parenchyma. (6) Once inside the brain the parasites are most likely controlled by macrophages or microglia which produce trypanotoxic or static molecules.
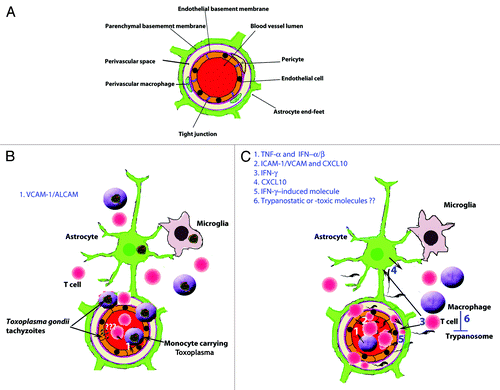
The endothelial cells of the leptomeningeal vessels are also linked together by tight junctions, but in spite of this they are more permeable to proteins than the cerebral vessels and they are not equipped with an astrocyte-derived parenchymal basement membrane.Citation15 Of relevance for spread of parasites to the brain also is the choroid plexus, which has fenestrations in the endothelial cells of the vessels that permit passage of proteins from the blood into the stroma. Further passage into the cerebrospinal fluid (CSF) is, however, prevented by the choroid plexus epithelial cells, which are linked to each other by tight junctions. Similarly, the vessels of the circumventricular organs (CVOs), which are secretory or sensory organs distributed along the walls of the third and fourth cerebral ventricles, are fenestrated to provide direct contact between nervous tissue elements and the blood.
These differences in the permeability of the vessels within these three territories, i.e., the brain parenchyma, leptomeninges and the choroid plexus/CVOs, play a role in the targeting of different parasites to the brain.
Parasite-Derived Factors that Promote BBB Crossing by Parasites
In order to enter into an organism or a cell, several parasites produce and secrete proteases to facilitate their passage across the skin, epithelial cells layers or plasma membranes.Citation16-Citation19 The question has therefore arisen as to whether parasites in the bloodstream could secrete proteases that would also facilitate their passage across the BBB (Box 1).
In vitro studies have shown the capabilities of free Toxoplasma gondii parasites to cross epithelial barriers made up of human foreskin fibroblast (HFF) cell monolayers.Citation20,Citation21 The Toxoplasma gondii adhesin micronemal protein 2 (MIC2) interaction with intercellular adhesion molecule 1 (ICAM-1) initiates this process.Citation21 Proteases and kinases that are released from Toxoplasma gondii’s apical secretory organelles rhoptries, micronemes and dense granules, in particular, serine- and cysteine-proteases play an important role in the parasite invasion of host cells by processing and shedding parasite-derived proteins that execute the penetration into the host cell (reviewed by Li et al.).Citation22 In this way the parasite can invade almost any nucleated cell in the body of the host including endothelial cells.Citation23-Citation25 Although Toxoplasma gondii parasites infect endothelial cells and modulate the transcriptome of these cells, almost none of the free parasites migrate across an in vitro co-culture (brain endothelial cells and astrocytes) model of the BBB in the absence of leukocytes.Citation24 In addition, in an in vivo study very few Toxoplasma gondii tachyzoites injected into the tail vein of mice were observed in the brain.Citation26 Thus, although Toxoplasma gondii can invade endothelial cells, its ability to cross the BBB as extracellular parasites in vivo is not clear.
Similarly, there are several molecules that are expressed on and released from the external surface of Trypanosoma brucei spp, which might promote their passage across endothelial cells. Trypanosomes express phosphatases on their external surface and can release cysteine- and metallo-proteases.Citation27-Citation30 A few of these proteases have been described to play a role in parasite penetration across cerebral endothelial cells in in vitro models of the BBB.Citation31-Citation34 For instance, in such in vitro models of human BBB, RNA interference against the cysteine proteases cathepsin L (brucipain) resulted in reduced passage of T. b. bruceiCitation31 similar to the effects of an irreversible inhibitor of cathepsin-L like proteases on passage of a T. b. rhodesiense strain.Citation32 Data from Grab and coworkers have also indicated that T. b. brucei transmigrate across a BBB in vitro model via the parasite-derived cysteine proteases or parasite-induced activation of endothelial cell G protein-coupled receptors (i.e., protease-activated receptor-2, PAR2).Citation34,Citation35 These studies therefore indicate a role for parasite-derived proteases, specifically brucipain, in the migration of trypanosomes across the BBB. The route for the passage taken by the parasite for crossing the BBB in vitro models is not clear, since both a paracellular and a transcellular route have been indicated.Citation29,Citation32,Citation33
Since most studies suggest that Toxoplasma gondii most likely invade the CNS as an intracellular parasite, research activities have tried to elucidate how parasite derived molecules play a role in attracting and increasing the migration of infected cells. A molecule secreted by Toxoplasma gondii, cyclophilin 18, interacts with the chemokine receptor CCR5 and this results in the production of nitric oxide (NO), interleukin 12 (IL-12), and tumor necrosis factor α (TNFα) as well as attraction of macrophages to the site of infection to increase the chances of parasite-leukocyte interaction.Citation36,Citation37 Once the parasite has invaded the immune cells it increases their motility and migratory activities via a Gi-protein coupled receptor signaling pathway.Citation38-Citation40 How the parasite activates the Gi-protein coupled receptor signaling pathway is still not known. Toxoplasma gondii-infected dendritic or monocytic CD11b+ cells, migrate from blood vessels to deliver parasites into the brain extravascular space of rodents () in a manner which is dependent on CD11b integrin function.Citation41
At the level of the blood-CSF barrier, Toxoplasma parasites can, in immunosuppressed humansCitation42 as well as in experimental models,Citation43 localize to the choroid plexus, which may serve as an point of entrance for the parasites to the CSF similar to what is described during infections with the lymphocytic choriomeningitis virus (LCMV).Citation44 In experimental rodent models, T. b. brucei also crosses the fenestrated vessels in the stroma of the choroid plexus () and CVOs very early after infection accompanied by WBCs and induction of cytokines including TNFα.Citation45,Citation46 TNFα can disrupt the choroid plexus epithelial barrier,Citation47 and trypanosomes may appear early during infection in the CSF when trypanotoxic drugs that do not pass across the BBB are still effective as seen in a primate model of the disease.Citation48 Although trypanosomes may cross damaged choroid plexus epithelial cells into the CSF, they do not seem to enter the brain parenchyma through the ependymal cell layer. Neither are there signs of accumulation of Toxoplasma parasites or Toxoplasma-infected cells around the ventricles.Citation43 Instead there are perivascular accumulations of WBCs and parasites around the intracerebral vessels, and this is most prominent within the white matter of the trypanosome-infected brain.
Figure 2. Large accumulation of T. b. brucei (red) in the choroid plexus following intra-peritoneal injection in a rodent model. Choroid plexus loaded with trypanosomes is seen already one week after the infection and before trypanosome crossing of the BBB, of which timing and prevalence is dependent on the rodent strain.
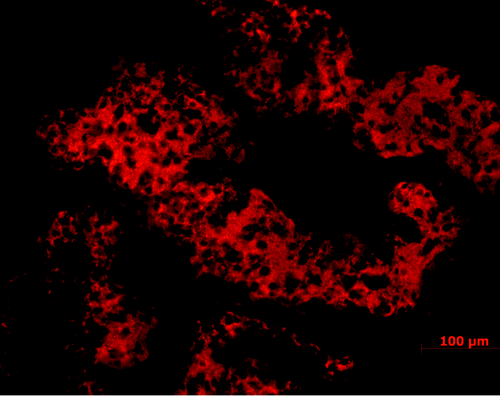
Both Toxoplasma parasites and African trypanosomes can cross the leptomeningeal vessels, and in trypanosome infections WBC infiltration in the leptomeninges precede inflammatory changes in the brain parenchyma.Citation49 Since both trypanosomes and WBCs can pass into the CSF before they have infiltrated the brain parenchyma, the correlation between numbers of WBCs in the CSF and severity of the disease in human African trypanosomiasis (HAT) patients is still under debate for therapeutic considerations, i.e., the use of drugs that cross the BBB or not.
Host-Derived Factors that Promote Crossing of the BBB by Parasites Associated with WBCs
Although the in vitro studies have shown that free-living Toxoplasma parasites and African trypanosomes by themselves can cross epithelial or endothelial cell layers, it is not clear to what extent such phenomena contributes to the neuroinvasion in vivo, in which host-derived mechanisms also may contribute to or even prevail in the process. As described in detail in a recent review,Citation50 the Toxoplasma parasite can infect monocytes and dendritic cells and be carried within such cells across the BBB (a “Trojan horse mechanism,” ). The extracellular trypanosomes may on the other hand follow T cells that pave their way into the brain by focal and transient openings of the BBB (Box 2).Citation51
During toxoplasmosis and trypanosomiasis, both in humans and experimental animal models, there is expression of host derived molecules such as adhesion molecules, chemokines, cytokines, metalloproteases, which play a role in the traversal of WBCs across the BBB and thereby also in the neuroinvasion of parasites.Citation24,Citation52-Citation54
In line with this hypothesis, it was observed that infection with Toxoplasma gondii resulted in an upregulation of the adhesion molecules activated leukocyte cell adhesion molecule (ALCAM), ICAM-1 and vascular cell adhesion molecule 1 (VCAM-1) in the CNS of C57BL/6 and BALB/c mice (both haplotype H-2d).Citation54 However, C57BL/6 mice that expressed more VCAM-1 and ALCAM had more inflammatory cells and Toxoplasma gondii parasites in the CNS than BALB/c mice,Citation54 suggesting an important role of these adhesion molecules in the differential CNS invasion by the parasite. A higher expression of interferon (IFN) -γ in C57BL/6 mice compared with BALB/c mice was most likely an important factor for the induction of the higher expression of VCAM-1 in the brains of the former mice;Citation54 since Toxoplasma gondii-infected IFNγ-deficient mice had less VCAM-1 than wild-type mice, but treatment of these mutant mice with recombinant IFNγ restored the expression of VCAM-1 in cerebral vessels.Citation55
The role played by host factors in African trypanosome neuroinvasion is further emphasized by the observation that in two major histocompatibility complex (MHC)-matched (haplotype H-2b) C57BL/6 and SV-129/Ev mice, an isolate of T. b. brucei crosses extensively the BBB in one strain of the mice (C57BL/6), but not in the other (129Sv/Ev), although the levels of parasites in the blood are even higher in the latter mouse strain.Citation56
We have observed that African trypanosomes enter the brain in two phases, namely first across the fenestrated vessels in the choroid plexus and CVOs as well as through leptomeningeal vessels, and then in a multi-step passage across the BBB. The role played by molecules released by the immune response for the multi-step passage of trypanosomes across the BBB has been studied systematically using a series of gene knockout C57BL/6 mice. Based on the following observations the passage of T. b. brucei across the BBB into the brain parenchyma appears to similar to that of WBCs during inflammation. Mice deficient of TNFα had reduced numbers of both parasites and T cells in the brain parenchyma compared with wild type mice.Citation57 Such mice also had a reduced expression of adhesion molecules, e.g., ICAM-1 on the cerebral endothelial cells, indicating that TNFα could facilitate T cell and parasite penetration by increasing expression of adhesion molecules.Citation58-Citation61 Furthermore, we have found that mice deficient of IFNγ, its receptor or recombination activating gene (RAG) had higher parasitemia than wild type mice, while the parasites as well as T cells accumulated in the perivascular space like cuffs.Citation51 The parasites passed the endothelial cell layer and its basement membrane but were trapped between this and the parenchymal basement membranes, which can be transiently opened for passage of T cells by activation of MMP-2 and -9.Citation62
Mice deficient of the IFNγ inducible chemokine CXCL10 or its receptor CXCR3 were found to have less parasites in the brain parenchyma albeit similar parasitemia levels compared with wild type mice.Citation63 CXCL10 was induced during the infection and expressed on cerebral endothelial cells and most prominently on astrocytes. This indicates that astrocytes by expressing CXCL10 could play a role by increasing the trafficking of both T cells and trypanosomes across the BBB or their retention in the brain parenchyma. However, cuffing of trypanosomes and T cells around cerebral vessels was not seen in these mice, which suggests that different IFNγ-regulated genes are involved in attraction or retention of T cells and trypanosomes on one hand and opening the parenchymal basement membrane for their passage on the other.
Interestingly, IFNα/β also plays a role in the neuroinvasion of T cells and trypanosomes, since IFNα/β receptor knockout mice show both less infiltration into the brain parenchyma as well as reduced levels of CXCL10. IFNα/β has been suggested to initiate a series of events that are amplified when IFNγ is induced following antigen-T cell recognition in the brain during infections with LCMV.Citation44 The following sequence of events for T. b. brucei crossing the BBB could therefore be suggested: The innate immune response molecules TNFα and IFNα/β are released upon activation of macrophages after stimulation of toll-like receptor (TLR) 9 by trypanosomal CpG-DNA.Citation57 TNFα induces ICAM/VCAM on cerebral endothelial cells which allow attachment of T cells. IFNα/β induces a limited release of CXCL10, which is enough for penetration of T cells accompanied by some trypanosomes into the perivascular space. Trypanosome-derived antigens taken up and expressed by macrophages could then be recognized by sensitized T cells to cause IFNγ release to augment the process; induce more CXCL10 in astrocytes and open the parenchymal basement membrane for more spread of both T cells and trypanosomes into the brain parenchyma ().
It is not clear why the T cells and trypanosomes have a predilection for invasion of the white matter and hypothalamic areas, but cytokines released into the CSF from the infected choroid plexus and CVOs could diffuse between ependymal cells and then permeate through the relatively wide extracellular spaces of the white matter, and nearby hypothalamic nuclei, respectively. Inflammatory cytokines in these areas could increase the immune response to augment T cells and trypanosome passage across the post-capillary venules.Citation64
The possible role of IFNγ and CXCL10 in the neuropathogenesis of the disease has been further corroborated with a clinical study in HAT patients.Citation65 Patients with higher plasma titers of IFNγ had increased frequency of progression to and severity of the meningoencephalitic stage of HAT than those with lower titers of the cytokine.Citation65 Moreover, CXCL10, which may be a biologically meaningful marker for inflammatory processes within the brain parenchyma since it is induced in astrocytes, was expressed more in the CSF of patients with late stage HAT in comparison to early stage HAT patients caused by either T. b. gambiense or T. b. rhodesiense.Citation63,Citation66-Citation68
From these studies it is apparent that immune response molecules and reaction to them play a crucial role for the brain invasion of both Toxoplasma gondii and T. b. brucei (). Infections with intracellular pathogens, like Toxoplasma gondii, activate mainly the Th1 arm of the immune response, whereas infections with extracellular pathogens including helminthes elicit Th2 cell responses.Citation69-Citation71 It is therefore paradoxical that infections of C57BL/6 mice with T. b. brucei elicit mainly a Th1 response in the host resulting in elevated levels of pro-inflammatory cytokines such as IFNγ and TNFα.Citation72-Citation76 Since these cytokines facilitate the infiltration of WBCs across the BBB in to the brain, the immune response promotes parasite dissemination into the brain in contrast to their traditional one in parasite control.Citation77-Citation79
Table 1. Interaction of selected blood-borne parasites with the blood-brain barrier (BBB)
Parasite Survival and Death in the Brain Parenchyma
As described in the previous section it is apparent that immune response derived molecules can facilitate the passage of WBCs and parasites across the barriers provided by the BBB. These molecules could therefore serve a dual function since they also provide an immunological barrier against further replication and spread of the parasites within the brain parenchyma. The immunological control of a pathogen within the immune-privileged brain presents a high order of complexity that is not well understoodCitation50 and beyond the scope of the present review. Only a few observations of relevance for the accumulation of parasites in the brain as a result of processes regulating both their spread into and control within the parenchyma will be pointed out.
In studies aiming at defining the role of host factors as determinants of parasite neuroinvasion or control of parasites it is often difficult to separate between these two different events, i.e., opening of the BBB for passage of parasites and control of their replication within the parenchyma. From studies on T. b. brucei infections in C57BL/6 mice data indicate that while TNFα and IFNα/β derived from the innate immune response promote the initiation of brain invasion of T cells and trypanosomes, they do not contribute to control of parasite growth in the brain.Citation57 Although the induction of TNFα and IFNα/β and the control of parasite growth in the brain is dependent on TLR9-myeloid differentiation primary response gene (88) (MyD88)-mediated signaling, the effector molecules for the control of parasite growth are distinct from those promoting invasion, but their nature is still not clear.Citation57
Once Toxoplasma gondii tachyzoites enter the brain parenchyma inside monocytes/dendritic cells they are transmitted to astrocytes, microglia and neurons. The rapidly replicating tachyzoites transform in to the very slowly replicating bradyzoites, which form cysts and persist as a chronic latent infection in the immunocompetent host. The importance of the immune response also in the control of growth of this parasite in the brain is demonstrated by the reactivation of the infection in an immunodeficient host, which results in encephalitis that can be fatal if not treated.Citation5,Citation6,Citation80,Citation81 IL-12 and IFNγ play an important role in the control of Toxoplasma gondii within the CNS and a number of effector molecules produced by different cells such as macrophages, microglia and astrocytes have been proposed.Citation82-Citation84 In addition, although infections with Toxoplasma gondii elicit a prominent Th1 immune response, a balancing Th2 immune response within the CNS has been observed in mice on a resistant BALBc background. IL-33, which is a recently described cytokine that amplifies the Th2 response, plays an essential role in controlling Toxoplasma gondii within the brain and limiting immune mediated neuropathology.Citation85 The effector molecules for the control of parasites in these mice, which is independent of TNFα and IFNγ, are not clear; a CD40-CD40L signaling, which increase autophagocytosis, has been pointed out as one possible mechanism for the parasite growth control.Citation85
BBB Interactions with other Parasites
Several other parasites, besides Toxoplasma gondii and Trypanosoma brucei spp, affect the CNS resulting in devastating or lethal consequences to the host, with or without crossing the BBB as will be briefly described for comparisons ().
In contrast to the African trypanosomes, Trypanosoma cruzi, which is prevalent in Latin America, replicates intra-cellularly. Infrequently, and mainly in children less than 2 y old, the parasites can spread to the brain and form nests in astrocytes at an acute stage of the disease. In immunosuppressed patients with chronic disease the infection in the brain can be reactivated showing necrosis and large nodular lesions with numerous parasites in astrocytes.Citation86 The mechanisms for spread of the parasites to the brain are not clear, but probably involve dissemination of infected WBCs through the BBB.Citation87,Citation88
The endothelial cells and their tight junctions present the first impediments parasites encounter when they cross the BBB. In addition, they play an important role in the neuropathogenesis of infections with the parasite Plasmodium falciparum, which does not enter the brain.Citation89 P. falciparum infested erythrocytes attach to the activated cerebral vessel endothelial cells via the P. falciparum erythrocyte membrane protein-1 (PfEMP-1), thus, are sequestered in the CNS and cause microcirculatory and neurological dysfunctions (cerebral malaria) without crossing the BBB. However, during cerebral malaria there is disruption of the BBB integrity and function.Citation90,Citation91 In experimental models of cerebral malaria there is induction of pro-inflammatory cytokines such as IFNγ and IL-12, which contribute to the neuropathology of the disease.Citation92 It is hypothesized that cerebral malaria could be a result of various factors such as pro-inflammatory immune mediated pathology, mechanical damage by sequestered infected erythrocytes, platelets or microparticles, or a combination of both factors i.e., immune pathology and mechanical damage.Citation93
Acanthamoeba invade the brain to cause granulomatous encephalitis mainly in immune compromised individuals. In vitro studies suggest that its passage across the BBB is facilitated by extracellular serine proteases which degrade the tight junction proteins resulting in increased permeability of the BBB.Citation94 Balamuthia mandrillaris also invades the CNS to cause encephalitis. Within the CNS the parasites are usually found clustered around blood vessels in localized areas of the brain, but they have also been found in the CSF of a patient who died of Balamuthia encephalitis.Citation95,Citation96 Using in vitro models the crossing of the human BBB by Balamuthia seems to be aided by a galactose-binding protein (GBP), induction of host cytokines and parasite metalloproteases.Citation97,Citation98 Balamuthia surface GBP can bind to endothelial cell galactose-containing glycoproteins and laminin, which possibly leads to attachment of the parasite to the BBB and later release of parasite proteases which degrade the tight junction proteins and basement membranes to aid its passage across the BBB.Citation95,Citation97,Citation99,Citation100
Toxocara canis larvae have been found in the choroid plexus and adjacent brain areas after oral administration of embryonated eggs. The mechanisms of invasion of the larvae into the brain parenchyma are not clear, but the presence of larvae is not correlated with enhanced BBB permeability.Citation101
Larvae of the pork tapeworm, Taenia solium can spread in the bloodstream and upon reaching small blood vessels in the brain, they lodge and start to develop into cysts. When the larvae die, after 3–5 y, an inflammatory reaction ensues and neurocysticercosis may develop, which results in neurological symptoms including epilepsy and intracranial hypertension.Citation102 Patients with symptomatic neurocysticercosis have increased expression of pro-inflammatory cytokines and adhesion molecules, TNFα, IFNγ, IL-1β and ICAM-1.Citation103 In a murine model of neurocysticercosis breakdown of the BBB and blood-cerebrospinal fluid barrier was observed and found to be associated with leukocyte migration into the CNS.Citation104 Recently, extensive astrogliosis, neuronal damage, rapid angiogenesis and disruption of BBB, which allowed an influx of WBCs into brain lesions, were observed in a porcine model of neurocysticercosis.Citation105
Neuroschistosomiasis is a severe clinical outcome associated with infection with the human pathogenic subspecies of Schistosoma, i.e., S. mansoni, S. hematobium and S. japonicum. Schistosoma eggs may spread to the CNS, through the arterial system after crossing previously developed pulmonary shunts or anastomosis or through retrograde venous flow. They are deposited in cerebral vessels and secrete antigens such as glycans and glycoproteins that elicit a Th2 immune response leading to granuloma formation.Citation106-Citation108 Adult worms can also migrate via vessels to reach meninges and the choroid plexus where they may shed massive amounts of eggs into the CNS.Citation107-Citation109
Olfactory Route
One way to circumvent the BBB is to invade the brain via the olfactory route, i.e., from the olfactory epithelia in the nasal cavity along the olfactory nerve into the CSF and brain. Although infrequent, certain free-living parasites found in water can infect humans along this route of neuroinvasion. The most prominent example is Naegleria fowleri, which can thrive in warm lakes as well as in untreated swimming pools, resort spas and hot springs.Citation110
After inhalation, the amoeba can attach to the olfactory epithelium possibly via a Nfa1 protein expressed in their food pockets.Citation111,Citation112 They may then move along the spaces between the unmyelinated nerve fibers of the fila olfactoria and traverse the cribriform plate to reach the CSF and olfactory bulbs.Citation113 Through further release of proteases, the parasites digest the olfactory bulb and spread into the brain parenchyma to cause a granulomatous meningoencephalitis.Citation114 Since it was first described in 1965, cases of infection by this amoeba have been observed around the world and the infections are almost invariably fatal.Citation110
Consequences of Parasite Invasion of the Brain
In general, infection of the CNS by a parasite is considered as a serious complication of the disease. Several parasitic infections of the brain are lethal if left untreated, (e.g., Balamuthia, African trypanosomes), while others can be controlled by the immune response to remain as a chronic or persistent infection (e.g., Toxoplasma gondii, Trypanosoma cruzi) or be cleared over time (e.g., Taenia solium larvae, the rat lungworm A. cantonensis), in spite of the fact that the brain provides an immunoprivileged site. In addition to life-threatening conditions, parasitic infections of the brain can cause disturbances of the brain function. For instance, they are a common cause of seizures and epilepsy (Taenia solium larvae, P. falciparum), disruption of the sleep pattern (African trypanosomes) and behavior or cognitive disturbances (P. falciparum).Citation64,Citation102,Citation115
By being inside the brain, parasites may have an advantage because of the less efficient immune responses in the brain and also be protected behind the BBB from circulating antibodies. Thereby, Toxoplasma parasites may remain in the brain for the life span of an infected rodent. To facilitate the spread of the parasite to its definite host, the cat, it has been suggested that persistent Toxoplasma infections in rodents may change the rodents’ behavior to show reduced avoidance of the predator cats.Citation116,Citation117 It has also been suggested that African trypanosomes may hide in the brain, behind the BBB, between relapses in sub-optimally treated individuals.Citation118-Citation121 In contrast to toxoplasmosis, there are no obvious changes in the behavior of the host caused be this parasite that could be associated with an increased rate of transmission. However, persistence over long periods of time in a host animal would favor the chances of spread of the parasite within populations, since it is transmitted by tsetse flies, the bite of which is a relatively rare event.
Conclusions
Both intracellular and extracellular parasites that invade the brain can result in devastating or lethal consequences to the host. These parasites pose a significant threat to human health, mostly in low-income countries. There are gaps in knowledge in terms of the interplay between the parasite-derived molecules and host-derived molecules and the weight of their roles in parasite traversal of the BBB into the brain parenchyma. Parasite derived molecules, including adhesins and proteases, promote cell penetration of several parasites, and several studies indicate that they play an important role also in the traversal of Toxoplasma gondii and Trypanosoma brucei spp across in vitro models of the BBB. The role of these parasite derived molecules in migration of parasites across the BBB in an in vivo setting warrant to be evaluated, because they might present novel drug targets to reduce neuropathology in these parasitic infections. For instance, molecules on the surface of parasites, which play a role in parasite interaction with the BBB, such as MIC2 for Toxoplasma gondii, PfEMP-1 for Plasmodium falciparum, are plausible targets for anti-parasitic vaccine development.Citation122-Citation124 In in vivo settings host derived immune response molecules are, however, crucial in the migration of these parasites into the brain parenchyma. The immune response molecules that promote passage of the parasites across the BBB may be distinct from those that inhibit their growth within the brain. The immune response can therefore have the dual and partly paradoxical function to on one hand reduce parasite growth in the brain, but on the other promote their brain invasion. Further research on the role of host derived molecules and the immune system on the invasion of the CNS and control of the parasites inside the CNS would not only further the knowledge of the neuropathology of parasitic diseases, but could provide a platform for drug discovery to reduce CNS invasion and ameliorate immune mediated pathology in these parasitic CNS infections.
Box 1. Suggested roles of parasite-derived factors in Trypanosoma brucei spp and Toxoplasma gondii crossing of the BBB
Trypanosoma brucei spp cross the BBB as extracellular parasites. T. brucei-derived cysteine proteases (i.e., brucipain) may interact with host endothelial cell G protein-coupled receptors (i.e., protease-activated receptor-2, PAR2). It has been suggested that this results in an increase in BBB permeability which promote parasite crossing of the BBB.Citation31-Citation35 The extracellular form of Toxoplasma gondii’s adhesin MIC2 interacts with host ICAM-1 to initiate the free parasite to cross epithelial barriers made up of human foreskin fibroblast (HFF) cell monolayers.Citation20,Citation21 In order to cross the BBB as an intracellular parasite Toxoplasma gondii secreted cyclophilin 18 interacts with the chemokine receptor CCR5 and attracts WBCs to the site of infection.Citation36,Citation37Toxoplasma gondii derived proteases process and shed parasite-derived proteins necessary for the penetration into the host cell. Once the parasite has invaded the immune cells (e.g., CD11b+ monocytes or dendritic cells) it increases their motility and migratory activities from blood vessels to deliver parasites into the brain extravascular space in a manner that is dependent on CD11b integrin function.Citation38-Citation41
Box 2. Suggested roles of host-derived factors in Trypanosoma brucei spp and Toxoplasma gondii crossing of the BBB
T. b. brucei cross the BBB into the brain parenchyma in a multi-step passage that appear to be similar to that of T cells during inflammation. Results obtained using animal models of African trypanosomiasis including genetic modified rodents indicate an important role of host-derived molecules in T cell and parasite penetration across the BBB. These molecules include the cytokines IFNα/β, IFNγ and TNFα, the chemokine CXCL10 and possibly the adhesion molecules i.e., ICAM-1.Citation51,Citation57,Citation63 The possible role of IFNγ and CXCL10 in the neuropathogenesis of the disease has been further corroborated with a clinical study in HAT patients and analysis of CSF of patients with HAT.Citation63,Citation65 The Toxoplasma parasite most likely cross the BBB inside leukocytes.Citation41,Citation50 Host-derived molecules such as ALCAM, ICAM-1, VCAM-1 and IFNγ, which facilitate the passage of leukocytes across the BBB, have also implicated in the migration of Toxoplasma gondii infested CD11b+ monocytes/dendritic cells to deliver parasites into the brain parenchyma.Citation54,Citation55
Abbreviations: | ||
ALCAM | = | activated leukocyte cell adhesion molecule |
BBB | = | blood-brain barrier |
CSF | = | cerebrospinal fluid |
CNS | = | central nervous system |
CVOs | = | circumventricular organs |
CXCL10 | = | C-X-C motif chemokine 10 |
GBP | = | galactose-binding protein |
HAT | = | human African trypanosomiasis |
HFF | = | human foreskin fibroblast |
ICAM-1 | = | intercellular adhesion molecule 1 |
IFN | = | interferon |
IL | = | interleukin |
LCMV | = | lymphocytic choriomeningitis virus |
MBP | = | mannose-binding protein |
MHC | = | major histocompatibility complex |
MIC2 | = | micronemal protein 2 |
MMP | = | matrix metalloprotease |
MyD88 | = | myeloid differentiation primary response gene (88) |
ND | = | not defined |
NO | = | nitric oxide |
PAR2 | = | protease-activated receptor-2 |
PfEMP-1 | = | Plasmodium falciparum erythrocyte membrane protein 1 |
RAG | = | recombination activating gene |
TLR | = | Toll-like receptor |
TNF | = | tumor necrosis factor |
VCAM-1 | = | vascular cell adhesion molecule 1 |
WBCs | = | white blood cells |
Acknowledgments
The studies have been in part supported by grants from the US NIH/Fogarty (1R21NS064888-01A1), and the Swedish Research Council (04480).
References
- Martinez FO, Helming L, Gordon S. Alternative activation of macrophages: an immunologic functional perspective. Annu Rev Immunol 2009; 27:451 - 83; http://dx.doi.org/10.1146/annurev.immunol.021908.132532; PMID: 19105661
- Bencurova E, Mlynarcik P, Bhide M. An insight into the ligand-receptor interactions involved in the translocation of pathogens across blood-brain barrier. FEMS Immunol Med Microbiol 2011; 63:297 - 318; http://dx.doi.org/10.1111/j.1574-695X.2011.00867.x; PMID: 22092557
- Kim KS. Mechanisms of microbial traversal of the blood-brain barrier. Nat Rev Microbiol 2008; 6:625 - 34; http://dx.doi.org/10.1038/nrmicro1952; PMID: 18604221
- Kristensson K. Microbes’ roadmap to neurons. Nat Rev Neurosci 2011; 12:345 - 57; http://dx.doi.org/10.1038/nrn3029; PMID: 21587289
- Montoya JG, Liesenfeld O. Toxoplasmosis. Lancet 2004; 363:1965 - 76; http://dx.doi.org/10.1016/S0140-6736(04)16412-X; PMID: 15194258
- Dellacasa-Lindberg I, Hitziger N, Barragan A. Localized recrudescence of Toxoplasma infections in the central nervous system of immunocompromised mice assessed by in vivo bioluminescence imaging. Microbes Infect 2007; 9:1291 - 8; http://dx.doi.org/10.1016/j.micinf.2007.06.003; PMID: 17897859
- Abbott NJ, Rönnbäck L, Hansson E. Astrocyte-endothelial interactions at the blood-brain barrier. Nat Rev Neurosci 2006; 7:41 - 53; http://dx.doi.org/10.1038/nrn1824; PMID: 16371949
- Wilhelm I, Fazakas C, Krizbai IA. In vitro models of the blood-brain barrier. Acta Neurobiol Exp (Wars) 2011; 71:113 - 28; PMID: 21499332
- Abbott NJ, Patabendige AA, Dolman DE, Yusof SR, Begley DJ. Structure and function of the blood-brain barrier. Neurobiol Dis 2010; 37:13 - 25; http://dx.doi.org/10.1016/j.nbd.2009.07.030; PMID: 19664713
- Armulik A, Genové G, Mäe M, Nisancioglu MH, Wallgard E, Niaudet C, et al. Pericytes regulate the blood-brain barrier. Nature 2010; 468:557 - 61; http://dx.doi.org/10.1038/nature09522; PMID: 20944627
- Sorokin L. The impact of the extracellular matrix on inflammation. Nat Rev Immunol 2010; 10:712 - 23; http://dx.doi.org/10.1038/nri2852; PMID: 20865019
- Engelhardt B, Sorokin L. The blood-brain and the blood-cerebrospinal fluid barriers: function and dysfunction. Semin Immunopathol 2009; 31:497 - 511; http://dx.doi.org/10.1007/s00281-009-0177-0; PMID: 19779720
- Sixt M, Engelhardt B, Pausch F, Hallmann R, Wendler O, Sorokin LM. Endothelial cell laminin isoforms, laminins 8 and 10, play decisive roles in T cell recruitment across the blood-brain barrier in experimental autoimmune encephalomyelitis. J Cell Biol 2001; 153:933 - 46; http://dx.doi.org/10.1083/jcb.153.5.933; PMID: 11381080
- Owens T, Bechmann I, Engelhardt B. Perivascular spaces and the two steps to neuroinflammation. J Neuropathol Exp Neurol 2008; 67:1113 - 21; http://dx.doi.org/10.1097/NEN.0b013e31818f9ca8; PMID: 19018243
- Broadwell RD, Sofroniew MV. Serum proteins bypass the blood-brain fluid barriers for extracellular entry to the central nervous system. Exp Neurol 1993; 120:245 - 63; http://dx.doi.org/10.1006/exnr.1993.1059; PMID: 8491281
- Dowse TJ, Koussis K, Blackman MJ, Soldati-Favre D. Roles of proteases during invasion and egress by Plasmodium and Toxoplasma. Subcell Biochem 2008; 47:121 - 39; http://dx.doi.org/10.1007/978-0-387-78267-6_10; PMID: 18512347
- Dvorák J, Mashiyama ST, Braschi S, Sajid M, Knudsen GM, Hansell E, et al. Differential use of protease families for invasion by schistosome cercariae. Biochimie 2008; 90:345 - 58; http://dx.doi.org/10.1016/j.biochi.2007.08.013; PMID: 17936488
- He C, Nora GP, Schneider EL, Kerr ID, Hansell E, Hirata K, et al. A novel Entamoeba histolytica cysteine proteinase, EhCP4, is key for invasive amebiasis and a therapeutic target. J Biol Chem 2010; 285:18516 - 27; http://dx.doi.org/10.1074/jbc.M109.086181; PMID: 20378535
- Salter JP, Lim KC, Hansell E, Hsieh I, McKerrow JH. Schistosome invasion of human skin and degradation of dermal elastin are mediated by a single serine protease. J Biol Chem 2000; 275:38667 - 73; http://dx.doi.org/10.1074/jbc.M006997200; PMID: 10993899
- Barragan A, Sibley LD. Transepithelial migration of Toxoplasma gondii is linked to parasite motility and virulence. J Exp Med 2002; 195:1625 - 33; http://dx.doi.org/10.1084/jem.20020258; PMID: 12070289
- Barragan A, Brossier F, Sibley LD. Transepithelial migration of Toxoplasma gondii involves an interaction of intercellular adhesion molecule 1 (ICAM-1) with the parasite adhesin MIC2. Cell Microbiol 2005; 7:561 - 8; http://dx.doi.org/10.1111/j.1462-5822.2005.00486.x; PMID: 15760456
- Li H, Child MA, Bogyo M. Proteases as regulators of pathogenesis: Examples from the apicomplexa. Biochim Biophys Acta 2012; 1824:177 - 85; PMID: 21683169
- Däubener W, Spors B, Hucke C, Adam R, Stins M, Kim KS, et al. Restriction of Toxoplasma gondii growth in human brain microvascular endothelial cells by activation of indoleamine 2,3-dioxygenase. Infect Immun 2001; 69:6527 - 31; http://dx.doi.org/10.1128/IAI.69.10.6527-6531.2001; PMID: 11553600
- Lachenmaier SM, Deli MA, Meissner M, Liesenfeld O. Intracellular transport of Toxoplasma gondii through the blood-brain barrier. J Neuroimmunol 2011; 232:119 - 30; http://dx.doi.org/10.1016/j.jneuroim.2010.10.029; PMID: 21106256
- Taubert A, Krüll M, Zahner H, Hermosilla C. Toxoplasma gondii and Neospora caninum infections of bovine endothelial cells induce endothelial adhesion molecule gene transcription and subsequent PMN adhesion. Vet Immunol Immunopathol 2006; 112:272 - 83; http://dx.doi.org/10.1016/j.vetimm.2006.03.017; PMID: 16730378
- Unno A, Suzuki K, Xuan X, Nishikawa Y, Kitoh K, Takashima Y. Dissemination of extracellular and intracellular Toxoplasma gondii tachyzoites in the blood flow. Parasitol Int 2008; 57:515 - 8; http://dx.doi.org/10.1016/j.parint.2008.06.004; PMID: 18652914
- Bakalara N, Santarelli X, Davis C, Baltz T. Purification, cloning, and characterization of an acidic ectoprotein phosphatase differentially expressed in the infectious bloodstream form of Trypanosoma brucei. J Biol Chem 2000; 275:8863 - 71; http://dx.doi.org/10.1074/jbc.275.12.8863; PMID: 10722732
- Lonsdale-Eccles JD, Grab DJ. Trypanosome hydrolases and the blood-brain barrier. Trends Parasitol 2002; 18:17 - 9; http://dx.doi.org/10.1016/S1471-4922(01)02120-1; PMID: 11850009
- Grab DJ, Nikolskaia O, Kim YV, Lonsdale-Eccles JD, Ito S, Hara T, et al. African trypanosome interactions with an in vitro model of the human blood-brain barrier. J Parasitol 2004; 90:970 - 9; http://dx.doi.org/10.1645/GE-287R; PMID: 15562595
- de Sousa KP, Atouguia J, Silva MS. Partial biochemical characterization of a metalloproteinase from the bloodstream forms of Trypanosoma brucei brucei parasites. Protein J 2010; 29:283 - 9; http://dx.doi.org/10.1007/s10930-010-9250-8; PMID: 20496101
- Abdulla MH, O’Brien T, Mackey ZB, Sajid M, Grab DJ, McKerrow JH. RNA interference of Trypanosoma brucei cathepsin B and L affects disease progression in a mouse model. PLoS Negl Trop Dis 2008; 2:e298; http://dx.doi.org/10.1371/journal.pntd.0000298; PMID: 18820745
- Nikolskaia OV, de A Lima AP, Kim YV, Lonsdale-Eccles JD, Fukuma T, Scharfstein J, et al. Blood-brain barrier traversal by African trypanosomes requires calcium signaling induced by parasite cysteine protease. J Clin Invest 2006; 116:2739 - 47; PMID: 16998589
- Nikolskaia OV, Kim YV, Kovbasnjuk O, Kim KJ, Grab DJ. Entry of Trypanosoma brucei gambiense into microvascular endothelial cells of the human blood-brain barrier. Int J Parasitol 2006; 36:513 - 9; http://dx.doi.org/10.1016/j.ijpara.2006.01.011; PMID: 16620822
- Grab DJ, Garcia-Garcia JC, Nikolskaia OV, Kim YV, Brown A, Pardo CA, et al. Protease activated receptor signaling is required for African trypanosome traversal of human brain microvascular endothelial cells. PLoS Negl Trop Dis 2009; 3:e479; http://dx.doi.org/10.1371/journal.pntd.0000479; PMID: 19621073
- Grab DJ, Chakravorty SJ, van der Heyde H, Stins MF. How can microbial interactions with the blood-brain barrier modulate astroglial and neuronal function?. Cell Microbiol 2011; 13:1470 - 8; http://dx.doi.org/10.1111/j.1462-5822.2011.01661.x; PMID: 21824246
- Ibrahim HM, Bannai H, Xuan X, Nishikawa Y. Toxoplasma gondii cyclophilin 18-mediated production of nitric oxide induces Bradyzoite conversion in a CCR5-dependent manner. Infect Immun 2009; 77:3686 - 95; http://dx.doi.org/10.1128/IAI.00361-09; PMID: 19564392
- Ibrahim HM, Xuan X, Nishikawa Y. Toxoplasma gondii cyclophilin 18 regulates the proliferation and migration of murine macrophages and spleen cells. Clin Vaccine Immunol 2010; 17:1322 - 9; http://dx.doi.org/10.1128/CVI.00128-10; PMID: 20660134
- Dellacasa-Lindberg I, Fuks JM, Arrighi RB, Lambert H, Wallin RP, Chambers BJ, et al. Migratory activation of primary cortical microglia upon infection with Toxoplasma gondii. Infect Immun 2011; 79:3046 - 52; http://dx.doi.org/10.1128/IAI.01042-10; PMID: 21628522
- Lambert H, Hitziger N, Dellacasa I, Svensson M, Barragan A. Induction of dendritic cell migration upon Toxoplasma gondii infection potentiates parasite dissemination. Cell Microbiol 2006; 8:1611 - 23; http://dx.doi.org/10.1111/j.1462-5822.2006.00735.x; PMID: 16984416
- Lambert H, Dellacasa-Lindberg I, Barragan A. Migratory responses of leukocytes infected with Toxoplasma gondii. Microbes Infect 2011; 13:96 - 102; http://dx.doi.org/10.1016/j.micinf.2010.10.002; PMID: 20951223
- Courret N, Darche S, Sonigo P, Milon G, Buzoni-Gâtel D, Tardieux I. CD11c- and CD11b-expressing mouse leukocytes transport single Toxoplasma gondii tachyzoites to the brain. Blood 2006; 107:309 - 16; http://dx.doi.org/10.1182/blood-2005-02-0666; PMID: 16051744
- Falangola MF, Petito CK. Choroid plexus infection in cerebral toxoplasmosis in AIDS patients. Neurology 1993; 43:2035 - 40; PMID: 8413962
- Deckert M, Lütjen S, Leuker CE, Kwok LY, Strack A, Müller W, et al. Mice with neonatally induced inactivation of the vascular cell adhesion molecule-1 fail to control the parasite in Toxoplasma encephalitis. Eur J Immunol 2003; 33:1418 - 28; http://dx.doi.org/10.1002/eji.200322826; PMID: 12731069
- Thomsen AR. Lymphocytic choriomeningitis virus-induced central nervous system disease: a model for studying the role of chemokines in regulating the acute antiviral CD8+ T-cell response in an immune-privileged organ. J Virol 2009; 83:20 - 8; http://dx.doi.org/10.1128/JVI.00682-08; PMID: 18787010
- Quan N, Mhlanga JD, Whiteside MB, McCoy AN, Kristensson K, Herkenham M. Chronic overexpression of proinflammatory cytokines and histopathology in the brains of rats infected with Trypanosoma brucei. J Comp Neurol 1999; 414:114 - 30; http://dx.doi.org/10.1002/(SICI)1096-9861(19991108)414:1<114::AID-CNE9>3.0.CO;2-G; PMID: 10494082
- Schultzberg M, Ambatsis M, Samuelsson EB, Kristensson K, van Meirvenne N. Spread of Trypanosoma brucei to the nervous system: early attack on circumventricular organs and sensory ganglia. J Neurosci Res 1988; 21:56 - 61; http://dx.doi.org/10.1002/jnr.490210109; PMID: 3216412
- Schwerk C, Rybarczyk K, Essmann F, Seibt A, Molleken ML, Zeni P, et al. TNFalpha induces choroid plexus epithelial cell barrier alterations by apoptotic and nonapoptotic mechanisms. J Biomed Biotechnol 2010; 2010:307231.
- Ngotho M, Kagira JM, Kariuki C, Maina N, Thuita JK, Mwangangi DM, et al. Influence of trypanocidal therapy on the haematology of vervet monkeys experimentally infected with Trypanosoma brucei rhodesiense. Acta Trop 2011; 119:14 - 8; http://dx.doi.org/10.1016/j.actatropica.2011.02.013; PMID: 21420376
- Rodgers J. Trypanosomiasis and the brain. Parasitology 2010; 137:1995 - 2006; http://dx.doi.org/10.1017/S0031182009991806; PMID: 20028610
- Feustel SM, Meissner M, Liesenfeld O. Toxoplasma gondii and the blood-brain barrier. Virulence 2012; 3:172 - 82; http://dx.doi.org/10.4161/viru.19004; PMID: 22460645
- Masocha W, Robertson B, Rottenberg ME, Mhlanga J, Sorokin L, Kristensson K. Cerebral vessel laminins and IFN-gamma define Trypanosoma brucei brucei penetration of the blood-brain barrier. J Clin Invest 2004; 114:689 - 94; PMID: 15343387
- Deckert-Schlüter M, Schlüter D, Hof H, Wiestler OD, Lassmann H. Differential expression of ICAM-1, VCAM-1 and their ligands LFA-1, Mac-1, CD43, VLA-4, and MHC class II antigens in murine Toxoplasma encephalitis: a light microscopic and ultrastructural immunohistochemical study. J Neuropathol Exp Neurol 1994; 53:457 - 68; http://dx.doi.org/10.1097/00005072-199409000-00005; PMID: 7521903
- Strack A, Asensio VC, Campbell IL, Schlüter D, Deckert M. Chemokines are differentially expressed by astrocytes, microglia and inflammatory leukocytes in Toxoplasma encephalitis and critically regulated by interferon-gamma. Acta Neuropathol 2002; 103:458 - 68; http://dx.doi.org/10.1007/s00401-001-0491-7; PMID: 11935261
- Silva NM, Manzan RM, Carneiro WP, Milanezi CM, Silva JS, Ferro EA, et al. Toxoplasma gondii: the severity of toxoplasmic encephalitis in C57BL/6 mice is associated with increased ALCAM and VCAM-1 expression in the central nervous system and higher blood-brain barrier permeability. Exp Parasitol 2010; 126:167 - 77; http://dx.doi.org/10.1016/j.exppara.2010.04.019; PMID: 20434443
- Wang X, Michie SA, Xu B, Suzuki Y. Importance of IFN-gamma-mediated expression of endothelial VCAM-1 on recruitment of CD8+ T cells into the brain during chronic infection with Toxoplasma gondii. J Interferon Cytokine Res 2007; 27:329 - 38; http://dx.doi.org/10.1089/jir.2006.0154; PMID: 17477820
- Masocha W, Amin DN, Kristensson K, Rottenberg ME. Differential invasion of Trypanosoma brucei brucei and lymphocytes into the brain of C57BL/6 and 129Sv/Ev mice. Scand J Immunol 2008; 68:484 - 91; http://dx.doi.org/10.1111/j.1365-3083.2008.02170.x; PMID: 18822108
- Amin DN, Vodnala SK, Masocha W, Sun B, Kristensson K, Rottenberg ME. Distinct Toll-like Receptor Signals Regulate Cerebral Parasite Load and Interferon alpha/beta and Tumor Necrosis Factor alpha-Dependent T-Cell Infiltration in the Brains of Trypanosoma brucei-Infected Mice. J Infect Dis 2012; 205:320 - 32; http://dx.doi.org/10.1093/infdis/jir734; PMID: 22116836
- Osborn L, Hession C, Tizard R, Vassallo C, Luhowskyj S, Chi-Rosso G, et al. Direct expression cloning of vascular cell adhesion molecule 1, a cytokine-induced endothelial protein that binds to lymphocytes. Cell 1989; 59:1203 - 11; http://dx.doi.org/10.1016/0092-8674(89)90775-7; PMID: 2688898
- Bevilacqua MP, Stengelin S, Gimbrone MA Jr., Seed B. Endothelial leukocyte adhesion molecule 1: an inducible receptor for neutrophils related to complement regulatory proteins and lectins. Science 1989; 243:1160 - 5; http://dx.doi.org/10.1126/science.2466335; PMID: 2466335
- Hanemaaijer R, Koolwijk P, le Clercq L, de Vree WJ, van Hinsbergh VW. Regulation of matrix metalloproteinase expression in human vein and microvascular endothelial cells. Effects of tumour necrosis factor alpha, interleukin 1 and phorbol ester. Biochem J 1993; 296:803 - 9; PMID: 8280080
- Zeni P, Doepker E, Schulze-Topphoff U, Huewel S, Tenenbaum T, Galla HJ. MMPs contribute to TNF-alpha-induced alteration of the blood-cerebrospinal fluid barrier in vitro. Am J Physiol Cell Physiol 2007; 293:C855 - 64; http://dx.doi.org/10.1152/ajpcell.00470.2006; PMID: 17507431
- Masocha W, Rottenberg ME, Kristensson K. Migration of African trypanosomes across the blood-brain barrier. Physiol Behav 2007; 92:110 - 4; http://dx.doi.org/10.1016/j.physbeh.2007.05.045; PMID: 17582444
- Amin DN, Rottenberg ME, Thomsen AR, Mumba D, Fenger C, Kristensson K, et al. Expression and role of CXCL10 during the encephalitic stage of experimental and clinical African trypanosomiasis. J Infect Dis 2009; 200:1556 - 65; http://dx.doi.org/10.1086/644597; PMID: 19827943
- Kristensson K, Nygård M, Bertini G, Bentivoglio M. African trypanosome infections of the nervous system: parasite entry and effects on sleep and synaptic functions. Prog Neurobiol 2010; 91:152 - 71; http://dx.doi.org/10.1016/j.pneurobio.2009.12.001; PMID: 19995590
- Maclean L, Odiit M, Macleod A, Morrison L, Sweeney L, Cooper A, et al. Spatially and genetically distinct African Trypanosome virulence variants defined by host interferon-gamma response. J Infect Dis 2007; 196:1620 - 8; http://dx.doi.org/10.1086/522011; PMID: 18008245
- Amin DN, Ngoyi DM, Nhkwachi GM, Palomba M, Rottenberg M, Büscher P, et al. Identification of stage biomarkers for human African trypanosomiasis. Am J Trop Med Hyg 2010; 82:983 - 90; http://dx.doi.org/10.4269/ajtmh.2010.09-0770; PMID: 20519589
- Hainard A, Tiberti N, Robin X, Lejon V, Ngoyi DM, Matovu E, et al. A combined CXCL10, CXCL8 and H-FABP panel for the staging of human African trypanosomiasis patients. PLoS Negl Trop Dis 2009; 3:e459; http://dx.doi.org/10.1371/journal.pntd.0000459; PMID: 19554086
- Hainard A, Tiberti N, Robin X, Ngoyi DM, Matovu E, Enyaru JC, et al. Matrix metalloproteinase-9 and intercellular adhesion molecule 1 are powerful staging markers for human African trypanosomiasis. Trop Med Int Health 2011; 16:119 - 26; http://dx.doi.org/10.1111/j.1365-3156.2010.02642.x; PMID: 20958893
- Mosmann TR, Coffman RL. TH1 and TH2 cells: different patterns of lymphokine secretion lead to different functional properties. Annu Rev Immunol 1989; 7:145 - 73; http://dx.doi.org/10.1146/annurev.iy.07.040189.001045; PMID: 2523712
- Paul WE, Seder RA. Lymphocyte responses and cytokines. Cell 1994; 76:241 - 51; http://dx.doi.org/10.1016/0092-8674(94)90332-8; PMID: 7904900
- Pearce EJ, MacDonald AS. The immunobiology of schistosomiasis. Nat Rev Immunol 2002; 2:499 - 511; http://dx.doi.org/10.1038/nri843; PMID: 12094224
- Mhlanga JD, Bentivoglio M, Kristensson K. Neurobiology of cerebral malaria and African sleeping sickness. Brain Res Bull 1997; 44:579 - 89; http://dx.doi.org/10.1016/S0361-9230(97)00309-2; PMID: 9365801
- Magez S, Stijlemans B, Baral T, De Baetselier P. VSG-GPI anchors of African trypanosomes: their role in macrophage activation and induction of infection-associated immunopathology. Microbes Infect 2002; 4:999 - 1006; http://dx.doi.org/10.1016/S1286-4579(02)01617-9; PMID: 12106794
- Hertz CJ, Filutowicz H, Mansfield JM. Resistance to the African trypanosomes is IFN-gamma dependent. J Immunol 1998; 161:6775 - 83; PMID: 9862708
- Lucas R, Magez S, Songa B, Darji A, Hamers R, de Baetselier P. A role for TNF during African trypanosomiasis: involvement in parasite control, immunosuppression and pathology. Res Immunol 1993; 144:370 - 6; http://dx.doi.org/10.1016/S0923-2494(93)80082-A; PMID: 8278660
- Schleifer KW, Filutowicz H, Schopf LR, Mansfield JM. Characterization of T helper cell responses to the trypanosome variant surface glycoprotein. J Immunol 1993; 150:2910 - 9; PMID: 8454863
- Rosenberg GA, Estrada EY, Dencoff JE, Stetler-Stevenson WG. Tumor necrosis factor-alpha-induced gelatinase B causes delayed opening of the blood-brain barrier: an expanded therapeutic window. Brain Res 1995; 703:151 - 5; http://dx.doi.org/10.1016/0006-8993(95)01089-0; PMID: 8719627
- Gloor SM, Wachtel M, Bolliger MF, Ishihara H, Landmann R, Frei K. Molecular and cellular permeability control at the blood-brain barrier. Brain Res Brain Res Rev 2001; 36:258 - 64; http://dx.doi.org/10.1016/S0165-0173(01)00102-3; PMID: 11690623
- Yang GY, Gong C, Qin Z, Liu XH, Lorris Betz A. Tumor necrosis factor alpha expression produces increased blood-brain barrier permeability following temporary focal cerebral ischemia in mice. Brain Res Mol Brain Res 1999; 69:135 - 43; http://dx.doi.org/10.1016/S0169-328X(99)00007-8; PMID: 10350645
- Pereira-Chioccola VL, Vidal JE, Su C. Toxoplasma gondii infection and cerebral toxoplasmosis in HIV-infected patients. Future Microbiol 2009; 4:1363 - 79; http://dx.doi.org/10.2217/fmb.09.89; PMID: 19995194
- Weiss LM, Dubey JP. Toxoplasmosis: A history of clinical observations. Int J Parasitol 2009; 39:895 - 901; http://dx.doi.org/10.1016/j.ijpara.2009.02.004; PMID: 19217908
- Suzuki Y, Sa Q, Gehman M, Ochiai E. Interferon-gamma- and perforin-mediated immune responses for resistance against Toxoplasma gondii in the brain. Expert Rev Mol Med 2011; 13:e31; http://dx.doi.org/10.1017/S1462399411002018; PMID: 22005272
- Yap G, Pesin M, Sher A. Cutting edge: IL-12 is required for the maintenance of IFN-gamma production in T cells mediating chronic resistance to the intracellular pathogen, Toxoplasma gondii. J Immunol 2000; 165:628 - 31; PMID: 10878333
- Gazzinelli RT, Wysocka M, Hayashi S, Denkers EY, Hieny S, Caspar P, et al. Parasite-induced IL-12 stimulates early IFN-gamma synthesis and resistance during acute infection with Toxoplasma gondii. J Immunol 1994; 153:2533 - 43; PMID: 7915739
- Jones LA, Roberts F, Nickdel MB, Brombacher F, McKenzie AN, Henriquez FL, et al. IL-33 receptor (T1/ST2) signalling is necessary to prevent the development of encephalitis in mice infected with Toxoplasma gondii. Eur J Immunol 2010; 40:426 - 36; http://dx.doi.org/10.1002/eji.200939705; PMID: 19950183
- Pittella JE. Central nervous system involvement in Chagas disease: a hundred-year-old history. Trans R Soc Trop Med Hyg 2009; 103:973 - 8; http://dx.doi.org/10.1016/j.trstmh.2009.04.012; PMID: 19457529
- Rocha A, de Meneses AC, da Silva AM, Ferreira MS, Nishioka SA, Burgarelli MK, et al. Pathology of patients with Chagas’ disease and acquired immunodeficiency syndrome. Am J Trop Med Hyg 1994; 50:261 - 8; PMID: 8147485
- Da Silva AA, Pereira GV, de Souza AS, Silva RR, Rocha MS, Lannes-Vieira J. Trypanosoma cruzi-Induced Central Nervous System Alterations: From the Entry of Inflammatory Cells to Potential Cognitive and Psychiatric Abnormalities. J Neuroparasitol 2010; 1:101 - 13
- Combes V, El-Assaad F, Faille D, Jambou R, Hunt NH, Grau GE. Microvesiculation and cell interactions at the brain-endothelial interface in cerebral malaria pathogenesis. Prog Neurobiol 2010; 91:140 - 51; http://dx.doi.org/10.1016/j.pneurobio.2010.01.007; PMID: 20117166
- Hunt NH, Golenser J, Chan-Ling T, Parekh S, Rae C, Potter S, et al. Immunopathogenesis of cerebral malaria. Int J Parasitol 2006; 36:569 - 82; http://dx.doi.org/10.1016/j.ijpara.2006.02.016; PMID: 16678181
- Medana IM, Turner GD. Human cerebral malaria and the blood-brain barrier. Int J Parasitol 2006; 36:555 - 68; http://dx.doi.org/10.1016/j.ijpara.2006.02.004; PMID: 16616145
- Schmidt KE, Schumak B, Specht S, Dubben B, Limmer A, Hoerauf A. Induction of pro-inflammatory mediators in Plasmodium berghei infected BALB/c mice breaks blood-brain-barrier and leads to cerebral malaria in an IL-12 dependent manner. Microbes Infect 2011; 13:828 - 36; http://dx.doi.org/10.1016/j.micinf.2011.04.006; PMID: 21609776
- van der Heyde HC, Nolan J, Combes V, Gramaglia I, Grau GE. A unified hypothesis for the genesis of cerebral malaria: sequestration, inflammation and hemostasis leading to microcirculatory dysfunction. Trends Parasitol 2006; 22:503 - 8; http://dx.doi.org/10.1016/j.pt.2006.09.002; PMID: 16979941
- Siddiqui R, Emes R, Elsheikha H, Khan NA. Area 51: How do Acanthamoeba invade the central nervous system?. Trends Parasitol 2011; 27:185 - 9; http://dx.doi.org/10.1016/j.pt.2011.01.005; PMID: 21507718
- Matin A, Siddiqui R, Jayasekera S, Khan NA. Increasing importance of Balamuthia mandrillaris. Clin Microbiol Rev 2008; 21:435 - 48; http://dx.doi.org/10.1128/CMR.00056-07; PMID: 18625680
- Jayasekera S, Sissons J, Tucker J, Rogers C, Nolder D, Warhurst D, et al. Post-mortem culture of Balamuthia mandrillaris from the brain and cerebrospinal fluid of a case of granulomatous amoebic meningoencephalitis, using human brain microvascular endothelial cells. J Med Microbiol 2004; 53:1007 - 12; http://dx.doi.org/10.1099/jmm.0.45721-0; PMID: 15358823
- Matin A, Stins M, Kim KS, Khan NA. Balamuthia mandrillaris exhibits metalloprotease activities. FEMS Immunol Med Microbiol 2006; 47:83 - 91; http://dx.doi.org/10.1111/j.1574-695X.2006.00065.x; PMID: 16706791
- Siddiqui R, Khan NA. Balamuthia amoebic encephalitis: an emerging disease with fatal consequences. Microb Pathog 2008; 44:89 - 97; http://dx.doi.org/10.1016/j.micpath.2007.06.008; PMID: 17913450
- Matin A, Siddiqui R, Jung SY, Kim KS, Stins M, Khan NA. Balamuthia mandrillaris interactions with human brain microvascular endothelial cells in vitro. J Med Microbiol 2007; 56:1110 - 5; http://dx.doi.org/10.1099/jmm.0.47134-0; PMID: 17644721
- Rocha-Azevedo B, Jamerson M, Cabral GA, Silva-Filho FC, Marciano-Cabral F. The interaction between the amoeba Balamuthia mandrillaris and extracellular matrix glycoproteins in vitro. Parasitology 2007; 134:51 - 8; http://dx.doi.org/10.1017/S0031182006001272; PMID: 17032481
- Liao CW, Cho WL, Kao TC, Su KE, Lin YH, Fan CK. Blood-brain barrier impairment with enhanced SP, NK-1R, GFAP and claudin-5 expressions in experimental cerebral toxocariasis. Parasite Immunol 2008; 30:525 - 34; http://dx.doi.org/10.1111/j.1365-3024.2008.01048.x; PMID: 18627507
- Mahanty S, Garcia HH, Cysticercosis Working Group in Perú. Cysticercosis and neurocysticercosis as pathogens affecting the nervous system. Prog Neurobiol 2010; 91:172 - 84; http://dx.doi.org/10.1016/j.pneurobio.2009.12.008; PMID: 20035822
- Prasad A, Prasad KN, Gupta RK, Pradhan S. Increased expression of ICAM-1 among symptomatic neurocysticercosis. J Neuroimmunol 2009; 206:118 - 20; http://dx.doi.org/10.1016/j.jneuroim.2008.09.015; PMID: 18947884
- Alvarez JI, Teale JM. Breakdown of the blood brain barrier and blood-cerebrospinal fluid barrier is associated with differential leukocyte migration in distinct compartments of the CNS during the course of murine NCC. J Neuroimmunol 2006; 173:45 - 55; http://dx.doi.org/10.1016/j.jneuroim.2005.11.020; PMID: 16406118
- Sikasunge CS, Johansen MV, Phiri IK, Willingham AL 3rd, Leifsson PS. The immune response in Taenia solium neurocysticercosis in pigs is associated with astrogliosis, axonal degeneration and altered blood-brain barrier permeability. Vet Parasitol 2009; 160:242 - 50; http://dx.doi.org/10.1016/j.vetpar.2008.11.015; PMID: 19117683
- Schramm G, Haas H. Th2 immune response against Schistosoma mansoni infection. Microbes Infect 2010; 12:881 - 8; http://dx.doi.org/10.1016/j.micinf.2010.06.001; PMID: 20542135
- Ross AG, McManus DP, Farrar J, Hunstman RJ, Gray DJ, Li YS. Neuroschistosomiasis. J Neurol 2011.
- Ferrari TC, Moreira PR. Neuroschistosomiasis: clinical symptoms and pathogenesis. Lancet Neurol 2011; 10:853 - 64; http://dx.doi.org/10.1016/S1474-4422(11)70170-3; PMID: 21849166
- Pittella JE. Neuroschistosomiasis. Brain Pathol 1997; 7:649 - 62; http://dx.doi.org/10.1111/j.1750-3639.1997.tb01080.x; PMID: 9034571
- Heggie TW. Swimming with death: Naegleria fowleri infections in recreational waters. Travel Med Infect Dis 2010; 8:201 - 6; http://dx.doi.org/10.1016/j.tmaid.2010.06.001; PMID: 20970721
- Shin HJ, Cho MS, Jung SU, Kim HI, Park S, Kim HJ, et al. Molecular cloning and characterization of a gene encoding a 13.1 kDa antigenic protein of Naegleria fowleri. J Eukaryot Microbiol 2001; 48:713 - 7; http://dx.doi.org/10.1111/j.1550-7408.2001.tb00211.x; PMID: 11831780
- Cho MS, Jung SY, Park S, Kim KH, Kim HI, Sohn S, et al. Immunological characterizations of a cloned 13.1-kilodalton protein from pathogenic Naegleria fowleri. Clin Diagn Lab Immunol 2003; 10:954 - 9; PMID: 12965933
- Martinez AJ, Visvesvara GS. Free-living, amphizoic and opportunistic amebas. Brain Pathol 1997; 7:583 - 98; http://dx.doi.org/10.1111/j.1750-3639.1997.tb01076.x; PMID: 9034567
- Serrano-Luna J, Cervantes-Sandoval I, Tsutsumi V, Shibayama M. A biochemical comparison of proteases from pathogenic naegleria fowleri and non-pathogenic Naegleria gruberi. J Eukaryot Microbiol 2007; 54:411 - 7; http://dx.doi.org/10.1111/j.1550-7408.2007.00280.x; PMID: 17910685
- Idro R, Marsh K, John CC, Newton CR. Cerebral malaria: mechanisms of brain injury and strategies for improved neurocognitive outcome. Pediatr Res 2010; 68:267 - 74; http://dx.doi.org/10.1203/PDR.0b013e3181eee738; PMID: 20606600
- Gulinello M, Acquarone M, Kim JH, Spray DC, Barbosa HS, Sellers R, et al. Acquired infection with Toxoplasma gondii in adult mice results in sensorimotor deficits but normal cognitive behavior despite widespread brain pathology. Microbes Infect 2010; 12:528 - 37; http://dx.doi.org/10.1016/j.micinf.2010.03.009; PMID: 20348009
- Lamberton PH, Donnelly CA, Webster JP. Specificity of the Toxoplasma gondii-altered behaviour to definitive versus non-definitive host predation risk. Parasitology 2008; 135:1143 - 50; http://dx.doi.org/10.1017/S0031182008004666; PMID: 18620624
- Jennings FW, Gray GD. Relapsed parasitaemia following chemotherapy of chronic T. brucei infections in mice and its relation to cerebral trypanosomes. Contrib Microbiol Immunol 1983; 7:147 - 54; PMID: 6825436
- Jennings FW, Whitelaw DD, Holmes PH, Chizyuka HG, Urquhart GM. The brain as a source of relapsing Trypanosoma brucei infection in mice after chemotherapy. Int J Parasitol 1979; 9:381 - 4; http://dx.doi.org/10.1016/0020-7519(79)90089-4; PMID: 489242
- Moulton JE. Relapse infection after chemotherapy in goats experimentally infected with Trypanosoma brucei: pathological changes in central nervous system. Vet Pathol 1986; 23:21 - 8; PMID: 3946052
- Poltera AA, Sayer PD, Brighouse G, Bovell D, Rudin W. Immunopathological aspects of trypanosomal meningoencephalitis in vervet monkeys after relapse following Berenil treatment. Trans R Soc Trop Med Hyg 1985; 79:527 - 31; http://dx.doi.org/10.1016/0035-9203(85)90086-0; PMID: 4082265
- Ghumra A, Khunrae P, Ataide R, Raza A, Rogerson SJ, Higgins MK, et al. Immunisation with recombinant PfEMP1 domains elicits functional rosette-inhibiting and phagocytosis-inducing antibodies to Plasmodium falciparum. PLoS One 2011; 6:e16414; http://dx.doi.org/10.1371/journal.pone.0016414; PMID: 21305024
- Hviid L. The role of Plasmodium falciparum variant surface antigens in protective immunity and vaccine development. Hum Vaccin 2010; 6:84 - 9; http://dx.doi.org/10.4161/hv.6.1.9602; PMID: 19823032
- Rosenberg C, De Craeye S, Jongert E, Gargano N, Beghetto E, Del Porto P, et al. Induction of partial protection against infection with Toxoplasma gondii genotype II by DNA vaccination with recombinant chimeric tachyzoite antigens. Vaccine 2009; 27:2489 - 98; http://dx.doi.org/10.1016/j.vaccine.2009.02.058; PMID: 19368791
- Fernandez-Reyes D, Craig AG, Kyes SA, Peshu N, Snow RW, Berendt AR, et al. A high frequency African coding polymorphism in the N-terminal domain of ICAM-1 predisposing to cerebral malaria in Kenya. Hum Mol Genet 1997; 6:1357 - 60; http://dx.doi.org/10.1093/hmg/6.8.1357; PMID: 9259284
- McCormick CJ, Craig A, Roberts D, Newbold CI, Berendt AR. Intercellular adhesion molecule-1 and CD36 synergize to mediate adherence of Plasmodium falciparum-infected erythrocytes to cultured human microvascular endothelial cells. J Clin Invest 1997; 100:2521 - 9; http://dx.doi.org/10.1172/JCI119794; PMID: 9366566
- Newbold CI, Craig AG, Kyes S, Berendt AR, Snow RW, Peshu N, et al. PfEMP1, polymorphism and pathogenesis. Ann Trop Med Parasitol 1997; 91:551 - 7; http://dx.doi.org/10.1080/00034989760923; PMID: 9329992
- Oleinikov AV, Amos E, Frye IT, Rossnagle E, Mutabingwa TK, Fried M, et al. High throughput functional assays of the variant antigen PfEMP1 reveal a single domain in the 3D7 Plasmodium falciparum genome that binds ICAM1 with high affinity and is targeted by naturally acquired neutralizing antibodies. PLoS Pathog 2009; 5:e1000386; http://dx.doi.org/10.1371/journal.ppat.1000386; PMID: 19381252
- Smith JD, Craig AG, Kriek N, Hudson-Taylor D, Kyes S, Fagan T, et al. Identification of a Plasmodium falciparum intercellular adhesion molecule-1 binding domain: a parasite adhesion trait implicated in cerebral malaria. Proc Natl Acad Sci U S A 2000; 97:1766 - 71; http://dx.doi.org/10.1073/pnas.040545897; PMID: 10677532
- Alsam S, Kim KS, Stins M, Rivas AO, Sissons J, Khan NA. Acanthamoeba interactions with human brain microvascular endothelial cells. Microb Pathog 2003; 35:235 - 41; http://dx.doi.org/10.1016/j.micpath.2003.07.001; PMID: 14580387
- Khan NA, Siddiqui R. Acanthamoeba affects the integrity of human brain microvascular endothelial cells and degrades the tight junction proteins. Int J Parasitol 2009; 39:1611 - 6; http://dx.doi.org/10.1016/j.ijpara.2009.06.004; PMID: 19580812
- Matin A, Jeong SR, Stins M, Khan NA. Effects of human serum on Balamuthia mandrillaris interactions with human brain microvascular endothelial cells. J Med Microbiol 2007; 56:30 - 5; http://dx.doi.org/10.1099/jmm.0.46847-0; PMID: 17172513
- Matin A, Khan NA. Demonstration and partial characterization of ecto-ATPase in Balamuthia mandrillaris and its possible role in the host-cell interactions. Lett Appl Microbiol 2008; 47:348 - 54; http://dx.doi.org/10.1111/j.1472-765X.2008.02414.x; PMID: 18761612
- Othman AA, Abdel-Aleem GA, Saied EM, Mayah WW, Elatrash AM. Biochemical and immunopathological changes in experimental neurotoxocariasis. Mol Biochem Parasitol 2010; 172:1 - 8; http://dx.doi.org/10.1016/j.molbiopara.2010.03.006; PMID: 20302888