Abstract
Salmonella enterica serovars are Gram-negative bacterial pathogens responsible for human diseases including gastroenteritis and typhoid fever. After ingestion, Salmonella cross the intestinal epithelial barrier, where they are phagocytosed by macrophages and dendritic cells, which then enables their spread to systemic sites during cases of typhoid fever. Salmonella use two type 3 secretion systems encoded by Salmonella pathogenicity islands (SPI) 1 and 2 to inject virulence proteins into host cells to modify cellular functions. SPI1 is involved in host cell invasion and inflammation, whereas SPI2 is required for intracellular survival and replication within phagocytes, and systemic spread. In this study the contribution of nearly all known SPI2 effectors was examined in an in vivo model of murine typhoid fever and cell culture models of macrophage and epithelial cell infection. Unmarked, in-frame deletions of SPI2 effectors were engineered in S. enterica serovar Typhimurium and the ability of the 16 different mutants to colonize and replicate was examined. In the typhoid model, we found that ΔspvB and ΔspiC mutants were attenuated for colonization of intestinal and systemic sites, while the ΔsseF mutant was attenuated in systemic organs. In epithelial cells, all mutants replicated to the same extent as the wild-type. In macrophages, ΔspiC, ΔsteC, ΔspvB, ΔssseK1/K2/K3, ΔsifA, and ΔsifB strains replicated poorly in comparison to wild-type Salmonella. This study provides a thorough screen of the majority of the known SPI2 effectors evaluated under the same conditions in various models of infection, providing a foundation for comparative examination of the roles and interactions of these effectors.
Introduction
Salmonella enterica are Gram-negative, facultative intracellular pathogens that can cause a wide range of human illness, from enteric/typhoid fever (caused by serovar Typhi or Paratyphi) to gastroenteritis (caused by serovar Typhimurium).Citation1,Citation2 In cases of typhoid fever, after ingestion of S. enterica, the bacteria cross the intestinal barrier, predominantly through microfold cells (M-cells) in Peyer's patches, where they are taken up by CD18-expressing phagocytes. In these cells Salmonella replicates within a special phagocytic compartment termed the Salmonella-containing vacuole (SCV).Citation2–Citation4 Within the phagocyte, the bacteria disseminate to systemic sitesCitation2,Citation5–Citation8 through the reticuloendothelial system, moving primarily to the mesenteric lymph nodes (MLNs), spleen and liver.Citation2,Citation7
Type 3 secretion systems (T3SS) play an essential role in S. enterica systemic spread and pathogenesis by transporting virulence proteins, termed effectors, from the bacteria into host cells to promote bacterial invasion and survival.Citation1,Citation2,Citation4,Citation7,Citation9,Citation10 S. enterica serovar Typhimurium utilizes two T3SS, one encoded by Salmonella Pathogenicity Island (SPI) 1 and one by SPI2.Citation2,Citation4,Citation7,Citation9,Citation10 SPI1 is involved in bacterial uptake into non-phagocytic cells, such as epithelial cells, whereas SPI2 is activated within phagosomes and is required for survival within phagocytes and consequently, systemic disease.Citation1,Citation7,Citation9–Citation12 Growing evidence suggests that the roles of SPI1 and SPI2 are not as segregated as previously thought,Citation13–Citation15 including the finding that SPI2 is also activated inside the intestinal lumen.Citation16
SPI2 effectors mediate many cellular functions during Salmonella infection, including regulation and maintenance of the vacuolar membrane, increasing the motility of infected cells, targeting the SCV to the trans-Golgi network, forming an actin meshwork surrounding the SCV, and inducing the formation of Salmonella Induced Filaments (SIFs), which are long filamentous structures originating at the SCV.Citation2,Citation7,Citation17 Known roles of SPI2 effectors have been extensively reviewed (reviwed in refs. Citation2, Citation7 and Citation18). The elucidation of the role of SPI2 effectors in pathogenesis is important for our understanding of how Salmonella manipulate the host to successfully replicate and cause disease. To the best of our knowledge, no previous study has systematically compared deletion strains of SPI2 effectors in various models of infection. Most of the information about SPI2 effectors comes from individual studies examining only one or a few effectors. This work confirms the importance of SPI2 effectors in macrophage replication and systemic spread. It also enables direct comparison of the contribution of SPI2 effectors to Salmonella's ability to colonize and replicate in several different infection models.
Results
SPI2 effectors do not affect the replication of S. Typhimurium in cultured epithelial cells.
S. enterica serovar Typhimurium actively invade and replicate within epithelial cells, causing multiple changes in cellular organization.Citation19 A panel of SPI2 mutants () was generated and examined for their ability to replicate in HeLa epithelial cells. The replication index was determined by comparing colony forming units (CFU) at 2 and 6 h post-infection. All SPI2 mutants showed no significant differences in intracellular replication when compared to the wild-type strain (), confirming the lesser role for SPI2 effectors in epithelial cell interactions. The ΔssaR strain, which contains a mutation in the SPI2 apparatus that prevents translocation of SPI2 effectors,Citation20 was used as a control. This strain has been used previously as a SPI2 control strain.Citation20–Citation22
The ability of the SPI2 mutants to replicate in CaCo2 intestinal epithelial cells was also examined. Similar to what was seen in HeLa cells, no significant differences were seen between the replication index of the SPI2 mutants and wild-type Salmonella (). The ΔssaR strain was also used as a control in this model.
For both HeLa and CaCo2 cells, invasion of Salmonella mutants was examined, by looking at CFU 2 h post-infection. No significant differences were seen in CFU at this early time point (data not shown).
Multiple SPI2 effectors are required for replication in macrophages.
S. Typhimurium exploit macrophages and dendritic cells for replication and transport within the host in a SPI2-dependent manner.Citation7 Therefore, the replication of SPI2 effector mutants in the RAW264.Citation7 macrophage cell line was examined ().
Macrophages were infected with S. Typhimurium strains and harvested for colony counts. The replication index was determined by comparing CFU at 3 and 24 h post-infection. As expected, the ΔssaR strain, which is used as a control for no SPI2 effector secretion, replicated significantly less than wild-type Salmonella (p = 0.0118; ). Some SPI2 effector deletion strains were found to have lower levels of bacterial replication in the macrophages, whereas other mutations had little or no effect. The ΔsseFG double mutant, ΔsseF, ΔsseG, ΔslrP, ΔsteA, ΔsspH2, ΔsseI and ΔgogB mutant strains did not affect bacterial replication. However, the ΔsifA, ΔsseK1/K2/K3, ΔsteC, ΔspiC, ΔsifB and ΔspvB strains replicated at levels significantly lower than the wild-type (p = 0.0052, p = 0.0279, p = 0.0460, p = 0.0048, p = 0.0459, p = 0.0248, respectively; ). These data show that SifA, SseK1, SseK2, SseK3, SteC, SpiC, SifB and SpvB are important for enabling bacterial replication within cultured macrophages.
Select SPI2 effectors are required for systemic spread of S. Typhimurium in a murine typhoid fever infection model.
Salmonella colonization of intestinal organs. Because of the important role that SPI2 plays in macrophage replication and systemic spread, the SPI2 mutants were tested in a mouse model of typhoid fever. The CFU was examined in systemic and intestinal sites of C57BL/6 mice 5 d post-oral infection. As expected, the ΔssaR strain was significantly less abundant in the intestine (p < 0.0001) than the wild-type strain. Interestingly, colonization of the majority of SPI2 mutants was not significantly different from that of the wild-type at both systemic and intestinal sites (). In the colon, the colonization of the ΔspiC and ΔspvB strains was significantly lower than that of the wild-type (p = 0.0024 and p = 0.0009 respectively; ). In the cecum, the ΔspiC and ΔspvB strains also colonized at lower levels than the wild-type strain (p = 0.0006 and p = 0.0006 respectively; ). In the ileum, the ΔspiC, ΔsseI and ΔspvB strains colonized at significantly lower levels than the wild-type strain (p = 0.0166, p = 0.0405 and p = 0.0011 respectively; ). Taken together, these data suggest that the SpiC and SpvB proteins are important for S. Typhimurium colonization of intestinal sites in the typhoid fever model, while SseI appears to play a role in colonization of the ileum.
Salmonella colonization of systemic organs. Since SPI2 is required for systemic spread during typhoid fever, we examined bacterial burden at systemic locations, including the liver, spleen and mesenteric lymph nodes (MLN). The ΔssaR strain colonized at significantly lower levels than the wild-type strain in the liver, spleen and MLN (p = 0.0002, p = 0.0002 and p = 0.0004, respectively; ). In the liver, the ΔspiC and ΔspvB strains colonized at levels lower than the wild-type-infected mice (p = 0.0028 and p = 0.0023, respectively; ). In the spleen, the ΔspiC, ΔsseF and ΔspvB strains did not colonize as well as the wild-type (p = 0.0028, p = 0.0253 and p = 0.0018, respectively; ). In the MLN, the ΔspiC, ΔsseF and ΔspvB strains colonized significantly less than the wild-type (p = 0.0086, p = 0.0107 and p = 0.0102, respectively; ). Therefore, as seen in the intestinal tract, the ΔspiC and ΔspvB strains were attenuated in systemic organs. Interestingly we found that the ΔsseF strain was attenuated in the MLN and spleen, but not in the intestinal organs. This data suggests an important role for the SpiC, SpvB and SseF proteins in S. Typhimurium virulence in mice.
Salmonella colonization of bile. Salmonella has recently been shown to replicate extracellularly in the luminal bile of murine gallbladders.Citation23 The role of SPI2 effectors in bile replication has not been previously determined. Therefore, bacterial counts in the bile of infected mice were examined by extracting bile from the gallbladders and determining CFU. No statistically significant differences were found among the SPI2 effectors mutant strains in bile (). Despite the lack of significance, the results show a similar trend where the ΔssaR, ΔsseF and ΔspvB strains replicated at levels lower than wild-type Salmonella. Because oral infection was used for all gallbladder infections, it is interesting that these strains, which are attenuated in systemic sites, were not significantly attenuated in the bile. However, this apparent effect could be a result of the low proportion of gallbladders that are infected, even during wild-type infections.Citation23 Because of the large variation in CFU within replicates in this model, statistical significance was not obtained, however the potential for biological significance is evident.
Salmonella colonization during early phases of typhoid. To examine the early phases of Salmonella colonization in typhoid fever, we determined levels of colonization for three Salmonella strains, wild-type, ΔsseF and ΔspvB. The wild-type strain was used, as this is known to be invasive and replicate at high levels. We found that at 3 d post-infection the wild-type strain colonized at moderate levels in all organs tested (), the colonization at 3 d was lower than what we saw at 5 d post-infection (). The ΔspvB strain was tested as this strain is known to be severely attenuated in systemic disease.Citation18 We found that the ΔspvB strain colonized at significantly lower levels in the liver (p = 0.0079), spleen (p = 0.0159), colon (p = 0.0317) and ileum (p = 0.0317). Finally, the ΔsseF strain was also examined as the role of this effector in systemic disease is less well characterized and had a modest attenuation systemically at 5 d post-infection. We found that the ΔsseF strain only colonized at significantly lower levels in the colon (p = 0.0317), while in the cecum, ileum and MLNs there is a trend for slightly lower levels of colonization. Therefore, these data generally support what was seen at 5 d post-infection, with ΔspvB strain being attenuated, and the ΔsseF strain colonizing at slightly lower levels than wild-type.
Discussion
SPI2 effectors are known to be important for Salmonella replication within macrophages and systemic spread. This study provides a thorough and comparative analysis of SPI2 effectors in three different models of infection, namely an in vivo murine model for typhoid fever and two cell culture infection models, one using epithelial cells and the other macrophages (). Many of the studies of Salmonella effectors published to date have focused on only one or a few SPI2 effectors, thus making comparisons between studies difficult. Therefore, the aim of this study was to test many SPI2 deletion strains under the same conditions, allowing for direct comparisons of the roles played by different SPI2 effectors during infection. The data confirms previously identified phenotypes and characterizes further other previously untested effectors.
In this study the ΔspvB strain was attenuated in both the typhoid model and the macrophage replication model, which supports the well-characterized role of this effector as an ADP-ribosylating protein that transfers ADP-ribose onto monomeric actin, which is needed for virulence.Citation4,Citation24 Interestingly, the ΔspvB strain was found to colonize at statistically higher levels than the ΔssaR strain in the systemic sites, but not in the intestinal sites. This study shows that the ΔsseK1/K2/K3 triple knock-out strain was attenuated in macrophages, but not in any sites in the murine model or in the cultured epithelial cell model. This confirms previous reports that SseK1 and SseK2 are not required for systemic infection in mice.Citation25 Our macrophage results with the ΔsseK1/K2/K3 strain correlates with that found by our group previously (data not shown).Citation26 Brown et al.Citation26 did see a slight attenuation of the ΔsseK1/K2/K3 strain using a competitive index.
Because SpiC is thought to interact with proteins in the host cell, manipulating host cell function,Citation18 it was included in this screen. The ΔspiC strain was attenuated in both the typhoid and the macrophage replication model in this study. This information supports the model proposed by Yu et al.Citation7 whereby SpiC is involved in sensing the pH of the environment and permitting effector translocation. The results of our screens supports the current understanding of SpiC activity, and its importance during infection. It should also be noted that the ΔspiC strain replicated at levels significantly higher than the ΔssaR strain, which does not secrete any SPI2 effectors.Citation20 This work also highlights the importance of SpiC for bacterial replication in macrophages.
SseF and SseG are involved in the formation of SIFs and may play a role in microtubule bundling.Citation28–Citation30 This work also provides evidence for the slight attenuation of the ΔsseF strain in the mouse typhoid fever model. However, the ΔsseF, ΔsseG and ΔsseFG strains do not replicate significantly less than the wild-type strain in the macrophage model, although a slight attenuation was visible. It is striking that the ΔsseF strain was attenuated, but there was not a statistically significant attenuation in the ΔsseG and ΔsseFG strains in the typhoid model, as the SseF and SseG proteins have been shown by biochemical assays to directly interact with each other.Citation31 However, it has also been shown that while SseF and SseG frequently co-localize, they are also found separately within the cell.Citation28 Because we saw that the ΔsseF strain was attenuated in systemic organs, we complemented this mutation, and found that the complement strain replicated at levels comparable to wild-type.
It is interesting that the ΔspiC and ΔspvB strains were attenuated in both intestinal and systemic sites. This lead us to speculate that these effectors may have roles in both anatomical sites. However, it is possible that attenuation of these strains in the intestine influences their replication at systemic sites, or perhaps systemic attenuation leads to lower levels in the intestine due to low levels of intestinal re-seeding. In addition, we found that at 3 d post-infection, the ΔsseF and ΔspvB strains did not appear to be as attenuated as at 5 d, indicating these effectors may play an important role in replication at systemic sites.
Salmonella counts in the bile were examined to determine if there were correlations with the systemic sites, which could support the concept that the gallbladder is involved in the re-seeding of the intestine by systemic bacteria.Citation23,Citation32,Citation33 After examining the SPI2 deletion strains in the gallbladder, no statistically significant differences in the number of bacteria in bile were seen when compared to wild-type, likely due to the fact that only some mice had their gallbladders infected. Though not statistically significant, this data does pose some interesting trends. Firstly, the ΔssaR strain does not effectively colonize systemic sites, and is also seen at low levels in the bile. The same pattern is seen for the ΔspvB and ΔsseF strains. This supports the notion that bacteria in the gallbladder correlate to the numbers of systemic bacteria, and a threshold of Salmonella must be reached before bacteria begin to colonize the gallbladder.Citation23
It should be noted that there are limitations to a single knockout studies. In this study individual effectors were removed, therefore it is possible that more subtle phenotypes may exist. The use of double or triple mutants or the use of a competitive index would further elucidate the possible subtle phenotypes of these SPI2 effectors.
In summary, these results confirm that SPI2 effectors are not required for epithelial cell replication and that multiple SPI2 effectors are required for macrophage replication. The in vivo studies show that some SPI2 effectors may in fact play a role in intestinal colonization, as well as systemic spread of the disease. Interestingly, none of the mutants tested here were significantly attenuated for growth in bile, despite their attenuation in both systemic and intestinal sites. This study enables direct comparison between SPI2 effectors in various models of infection and provides groundwork for further studies on the roles of Salmonella SPI2 effectors.
Methods and Materials
Construction of bacterial strains, plasmids and growth conditions.
Salmonella enterica serovar Typhimurium and Escherichia coli strains were routinely grown on Luria-Bertani (LB) agar at 37°C, supplemented with the appropriate antibiotics (100 µg/mL streptomycin, 30 µg/mL chloramphenicol, 50 µg/mL kanamycin). Overnight cultures were grown in LB broth at 37°C with aeration with the appropriate antibiotics. E. coli MC1061 λpir was used for standard cloning, E. coli SM10λpir was used for conjugation and S. Typhimurium SL1344 strain was used to construct all of the in-frame deletions (). PCR was done with Pfu Turbo DNA Polymerase (Stratagene, 600252) to amplify ∼500 bp flanking regions of each gene (). PCR products were then joined using overlapping PCR.Citation34 Using restriction digestion with SacI and KpnI and T4 DNA ligation, this PCR product was cloned into the suicide vector pRE112,Citation35 which was transformed into E. coli MC1061λpir.Citation36 Following confirmation of the correct insert by DNA sequencing (BigDye Terminator v3.1 Cycle Sequencing, ABI, 4337456; NAPS unit, DNA Sequencing Laboratory, UBC), the suicide plasmids were transformed into E. coli SM10λpirCitation37 so that they could be mobilized into SL1344 by conjugation. Initial plasmid integration was identified by recovering chloramphenicol resistant colonies. Plasmid loss was selected for following growth for 4 h without chloramphenicol and by selecting for sucrose-resistant colonies. The desired genotype was confirmed by PCR and DNA sequencing.
The ΔsseF strain was complemented by first PCR amplifying the sseA promoter region and sseF (), then joining the products by overlapping PCR. BamH1 and Xho1 restriction digest followed by T4 DNA ligation was used to clone the fragment into pZA23MCS.Citation38 This plasmid (pZA23-sseF) was then electroporated into the ΔsseF Salmonella strain. The cloning was confirmed by restriction digest and DNA sequencing.
Macrophage and epithelial cell infections.
HeLa cells (American Type Culture Collection, ATCC; Manassas, VA) were seeded at 7 × 104 cells/well, CaCO2 (ATCC) were seeded at 2.5 × 105 cells/well and RAW264.7 cells (ATCC) were seeded at 1 × 105 cells/well in 24-well plates and grown for 24 h at 37°C, 5% CO2. All cells were grown in Dulbecco's modified Eagle medium (DMEM) with high glucose (Thermo Scientific, SH3024301) and 10% fetal bovine serum (Thermo Scientific, SH3039603), 1% non-essential amino acids (Gibco, 11140) and 1% GlutaMax (Gibco, 35050).
For HeLa infections, bacteria were grown overnight, sub-cultured for 3 h, then diluted and 105 bacteria were added to each well in DMEM for 10 min. DMEM with no bacteria was then added for 20 min. Cells were incubated with medium containing 50 µg/mL gentamicin for 90 min, then 10 µg/mL gentamicin for the remainder of the 2 and 6 h time points. Bacterial counts at 2 h post-infection were used as measures of bacterial invasion. Counts at 6 h were chosen to calculate the replication index as we have found that HeLa cells begin to die at later time points due to bacterial load. Bacteria were released from HeLa cells using lysis buffer containing 1% Triton X-100 (BDH, R06433) and 0.1% sodium dodecyl sulfate (Sigma-Aldrich, 151213) in phosphate-buffered saline (PBS), diluted in PBS and plated for colony counts.
For CaCo2 infections, overnight cultures were subcultured for 3 h, then 106 bacteria were added to each well in DMEM for 20 min. Cells were then incubated with 100 µg/mL gentamicin in DMEM for the remainder of the 2 h time point. Six hour samples were treated with 10 µg/mL gentamicin for the remainder of the time. Samples were washed and lysed with lysis buffer, then diluted in PBS and plated for colony counts. Calculations were done the same way as for the HeLa cell infections.
For RAW264.7 infections, 106 bacteria from an overnight culture were added to each well in DMEM for 20 min. Cells were then incubated in medium containing 100 µg/mL gentamicin for 60 min, then 10 µg/mL gentamicin for the remainder of the 3 and 24 h time points. Bacterial counts at 3 h were used as measures of invasion, and the 24 h time point was used to calculate the replication index, since it provided enough time for Salmonella to replicate, as we have found that Salmonella begin to replicate in RAW264.7 cells at 10 h post-infection. Bacteria were released from RAW264.7 cells using lysis buffer, diluted in PBS and plated for colony counts.
Mouse infections.
Overnight cultures of Salmonella were diluted in 100 mM HEPES with 0.9% sodium chloride (pH 8.0). Between 5 and 10 8-week-old female C57BL/6 mice (The Jackson Laboratory) were infected orally with 3–5 × 107 bacteria in 100 µl. All animal infections were performed in accordance with ethical requirements of the University of British Columbia's Animal Care Committee. Mice were euthanized 3 and 5 d post-infection and tissue samples, including the respective intestinal contents, were removed for bacterial quantification. Samples were homogenized using a mixer mill (MM 301, Retsch, Haan Germany) for 10 min at a frequency of 30/s. Samples were diluted in PBS and plated for colony counts. Bile counts were obtained by extracting bile from the gallbladder, bile was diluted in PBS and plated for colony counts.
Statistical analysis.
Statistical analysis was performed using GraphPad Prism, version 4.0 (GraphPad Software). Unpaired t-test was used to determine statistical significance.
Figures and Tables
Figure 1 Replication of S. Typhimurium SPI2 mutants in epithelial cells. (A) HeLa cells were infected with exponentially-growing Salmonella, with a multiplicity of infection of 14. (B) CaCO2 cells were infected with exponentially-growing Salmonella, with a multiplicity of infection of 10. Error bars represent standard errors of the means. Fold replication was determined by comparing bacterial counts at 2 and 6 h post-infection.
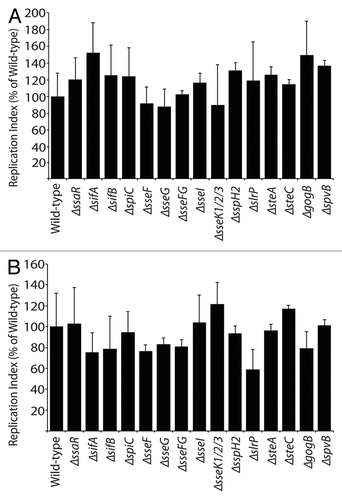
Figure 2 Replication of S. Typhimurium SPI2 mutants in RAW264.7 macrophages. Macrophages were infected with Salmonella, with a multiplicity of infection of 10. Fold replication was determined by comparing bacterial counts at 3 and 24 h post-infection. Error bars represent standard errors of the means. Asterisks indicate p < 0.05.
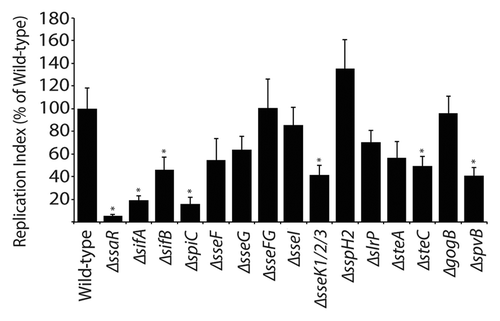
Figure 3 Bacterial counts of S. Typhimurium SPI2 mutants recovered from intestinal and systemic organs of C57BL/6 mice after oral infection. Mice were infected via oral gavage and sacrificed 5 d post-infection. Organs collected include ileum, cecum, colon, liver, spleen and mesenteric lymph nodes (MLN). Counts are given as colony-forming units (CFU) per milligram of tissue. Error bars represent standard deviation from the means. A minimum of 5 mice was used for each SPI2 mutant. Asterisks indicate **p < 0.001 or *p < 0.05.
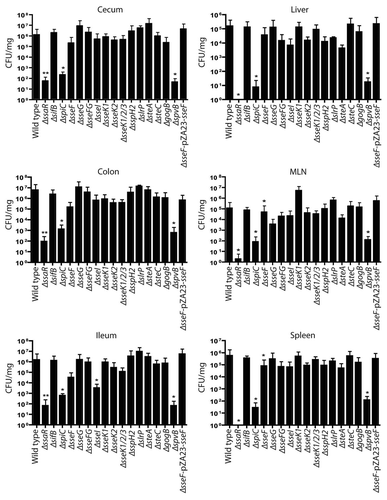
Figure 4 Bacterial counts of S. Typhimurium SPI2 mutants recovered from bile of C57BL/6 mice after oral infection. Mice were infected via oral gavage, sacrificed 5 d post-infection, and bile was extracted from gallbladders. Counts are given as colony-forming units (CFU) per microliter of bile. Error bars represent standard errors of the means. A minimum of 5 mice was used for each SPI2 mutant.
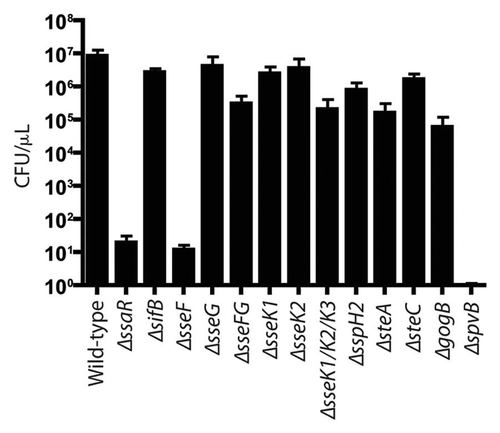
Figure 5 Bacterial count of S. Typhimurium SPI2 mutants recovered from systemic and intestinal organs of C57BL/6 mice 3 d post-infection. Mice were infected via oral gavage. Counts given represent colony forming units per milligram of tissue. Error bars represent standard errors from the means, each group contained 5 mice. Asterisks indicates *p < 0.05. Mesenteric lymph nodes (MLN).
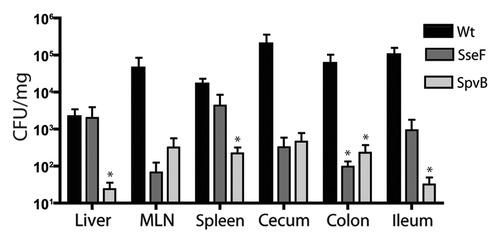
Table 1 Summary table of SPI2 mutants tested in RAW264.7 macrophage cells, HeLa and CaCo2 epithelial cells, and the murine typhoid fever model
Table 2 Bacterial strains and plasmids used in this study
Table 3 Oligonucleotides used to construct SPI2 gene deletions
Acknowledgments
We would like to thank L.C.M. Antunes and H.B. Yu for helpful guidance and members of the Finlay lab for their efforts reviewing this manuscript. This work was supported by grants from the Canadian Institutes of Health Research (CIHR), and the National Sciences and Engineering Research Council of Canada (NSERC). M.M.C.B. is supported by a graduate scholarship from NSERC. M.A.C. is supported by a Canadian Association of Gastroenterology/CIHR/Ferring Pharmaceuticals fellowship. E.T.A. is supported by University Graduate Fellowship and Armauer Hansen Memorial Fellowship. B.B.F. is an HHMI International Research Scholar and a University of British Columbia Peter Wall Distinguished Professor.
References
- Freeman JA, Ohl ME, Miller SI. The Salmonella enterica serovar typhimurium translocated effectors SseJ and SifB are targeted to the Salmonella-containing vacuole. Infect Immun 2003; 71:418 - 427
- Kuhle V, Hensel M. Cellular microbiology of intracellular Salmonella enterica: functions of the type III secretion system encoded by Salmonella pathogenicity island 2. Cell Mol Life Sci 2004; 61:2812 - 2826
- Poh J, Odendall C, Spanos A, Boyle C, Liu M, Freemont P, et al. SteC is a Salmonella kinase required for SPI-2-dependent F-actin remodelling. Cell Microbiol 2008; 10:20 - 30
- Browne SH, Hasegawa P, Okamoto S, Fierer J, Guiney DG. Identification of Salmonella SPI-2 secretion system components required for SpvB-mediated cytotoxicity in macrophages and virulence in mice. FEMS Immunol Med Microbiol 2008; 52:194 - 201
- Vazquez-Torres A, Jones-Carson J, Baumler AJ, Falkow S, Valdivia R, Brown W, et al. Extraintestinal dissemination of Salmonella by CD18-expressing phagocytes. Nature 1999; 401:804 - 808
- Jones BD, Ghori N, Falkow S. Salmonella typhimurium initiates murine infection by penetrating and destroying the specialized epithelial M cells of the Peyer's patches. J Exp Med 1994; 180:15 - 23
- Haraga A, Ohlson MB, Miller SI. Salmonellae interplay with host cells. Nat Rev Microbiol 2008; 6:53 - 66
- Kohbata S, Yokoyama H, Yabuuchi E. Cytopathogenic effect of Salmonella typhi GIFU 10007 on M cells of murine ileal Peyer's patches in ligated ileal loops: an ultrastructural study. Microbiol Immunol 1986; 30:1225 - 1237
- Coombes BK, Lowden MJ, Bishop JL, Wickham ME, Brown NF, Duong N, et al. SseL is a salmonella-specific translocated effector integrated into the SsrB-controlled salmonella pathogenicity island 2 type III secretion system. Infect Immun 2007; 75:574 - 580
- Geddes K, Worley M, Niemann G, Heffron F. Identification of new secreted effectors in Salmonella enterica serovar Typhimurium. Infect Immun 2005; 73:6260 - 6271
- Ochman H, Soncini FC, Solomon F, Groisman EA. Identification of a pathogenicity island required for Salmonella survival in host cells. Proc Natl Acad Sci USA 1996; 93:7800 - 7804
- Beuzon CR, Holden DW. Use of mixed infections with Salmonella strains to study virulence genes and their interactions in vivo. Microbes Infect 2001; 3:1345 - 1352
- Lilic M, Stebbins CE. Re-structuring the host cell: up close with Salmonella's molecular machinery. Microbes Infect 2004; 6:1205 - 1211
- Patel JC, Galan JE. Manipulation of the host actin cytoskeleton by Salmonella—all in the name of entry. Curr Opin Microbiol 2005; 8:10 - 15
- Ly KT, Casanova JE. Mechanisms of Salmonella entry into host cells. Cell Microbiol 2007; 9:2103 - 2111
- Brown NF, Vallance BA, Coombes BK, Valdez Y, Coburn BA, Finlay BB. Salmonella pathogenicity island 2 is expressed prior to penetrating the intestine. PLoS Pathog 2005; 1:32
- Brumell JH, Goosney DL, Finlay BB. SifA, a type III secreted effector of Salmonella typhimurium, directs Salmonella-induced filament (Sif) formation along microtubules. Traffic 2002; 3:407 - 415
- McGhie EJ, Brawn LC, Hume PJ, Humphreys D, Koronakis V. Salmonella takes control: effector-driven manipulation of the host. Curr Opin Microbiol 2009; 12:117 - 124
- Garcia-del Portillo F, Zwick MB, Leung KY, Finlay BB. Intracellular replication of Salmonella within epithelial cells is associated with filamentous structures containing lysosomal membrane glycoproteins. Infect Agents Dis 1993; 2:227 - 231
- Brumell JH, Rosenberger CM, Gotto GT, Marcus SL, Finlay BB. SifA permits survival and replication of Salmonella typhimurium in murine macrophages. Cell Microbiol 2001; 3:75 - 84
- Coombes BK, Wickham ME, Lowden MJ, Brown NF, Finlay BB. Negative regulation of Salmonella pathogenicity island 2 is required for contextual control of virulence during typhoid. Proc Natl Acad Sci USA 2005; 102:17460 - 17465
- Grassl GA, Valdez Y, Bergstrom KS, Vallance BA, Finlay BB. Chronic enteric salmonella infection in mice leads to severe and persistent intestinal fibrosis. Gastroenterology 2008; 134:768 - 780
- Menendez A, Arena ET, Guttman JA, Thorson L, Vallance BA, Vogl W, et al. Salmonella infection of gallbladder epithelial cells drives local inflammation and injury in a model of acute typhoid fever. J Infect Dis 2009; 200:1703 - 1713
- Lesnick ML, Reiner NE, Fierer J, Guiney DG. The Salmonella spvB virulence gene encodes an enzyme that ADP-ribosylates actin and destabilizes the cytoskeleton of eukaryotic cells. Mol Microbiol 2001; 39:1464 - 1470
- Kujat Choy SL, Boyle EC, Gal-Mor O, Goode DL, Valdez Y, Vallance BA, et al. SseK1 and SseK2 are novel translocated proteins of Salmonella enterica serovar typhimurium. Infect Immun 2004; 72:5115 - 5125
- Brown NF, Coombes BK, Bishop JL, Wickham ME, Lowden MJ, Gal-Mor O, et al. Salmonella phage ST64B encodes a member of the SseK/NleB effector family. PLoS One 2011; 6:17824
- Yu XJ, McGourty K, Liu M, Unsworth KE, Holden DW. pH sensing by intracellular Salmonella induces effector translocation. Science 2010; 328:1040 - 1043
- Kuhle V, Jackel D, Hensel M. Effector proteins encoded by Salmonella pathogenicity island 2 interfere with the microtubule cytoskeleton after translocation into host cells. Traffic 2004; 5:356 - 370
- Kuhle V, Hensel M. SseF and SseG are translocated effectors of the type III secretion system of Salmonella pathogenicity island 2 that modulate aggregation of endosomal compartments. Cell Microbiol 2002; 4:813 - 824
- Guy RL, Gonias LA, Stein MA. Aggregation of host endosomes by Salmonella requires SPI2 translocation of SseFG and involves SpvR and the fms-aroE intragenic region. Mol Microbiol 2000; 37:1417 - 1435
- Deiwick J, Salcedo SP, Boucrot E, Gilliland SM, Henry T, Petermann N, et al. The translocated Salmonella effector proteins SseF and SseG interact and are required to establish an intracellular replication niche. Infect Immun 2006; 74:6965 - 6972
- Lawley TD, Bouley DM, Hoy YE, Gerke C, Relman DA, Monack DM. Host transmission of Salmonella enterica serovar Typhimurium is controlled by virulence factors and indigenous intestinal microbiota. Infect Immun 2008; 76:403 - 416
- Everest P, Wain J, Roberts M, Rook G, Dougan G. The molecular mechanisms of severe typhoid fever. Trends Microbiol 2001; 9:316 - 320
- Croxen MA, Sisson G, Melano R, Hoffman PS. The Helicobacter pylori chemotaxis receptor TlpB (HP0103) is required for pH taxis and for colonization of the gastric mucosa. J Bacteriol 2006; 188:2656 - 2665
- Edwards RA, Keller LH, Schifferli DM. Improved allelic exchange vectors and their use to analyze 987P fimbria gene expression. Gene 1998; 207:149 - 157
- Rubires X, Saigi F, Pique N, Climent N, Merino S, Alberti S, et al. A gene (wbbL) from Serratia marcescens N28b (O4) complements the rfb-50 mutation of Escherichia coli K-12 derivatives. J Bacteriol 1997; 179:7581 - 7586
- Miller VL, Mekalanos JJ. A novel suicide vector and its use in construction of insertion mutations: osmoregulation of outer membrane proteins and virulence determinants in Vibrio cholerae requires toxR. J Bacteriol 1988; 170:2575 - 2583
- Lutz R, Bujard H. Independent and tight regulation of transcriptional units in Escherichia coli via the LacR/O, the TetR/O and AraC/I1-I2 regulatory elements. Nucleic Acids Res 1997; 25:1203 - 1210
- Hoiseth SK, Stocker BA. Aromatic-dependent Salmonella typhimurium are non-virulent and effective as live vaccines. Nature 1981; 291:238 - 239
- Stein MA, Leung KY, Zwick M, Garcia-del Portillo F, Finlay BB. Identification of a Salmonella virulence gene required for formation of filamentous structures containing lysosomal membrane glycoproteins within epithelial cells. Mol Microbiol 1996; 20:151 - 164
- Coombes BK, Wickham ME, Brown NF, Lemire S, Bossi L, Hsiao WW, et al. Genetic and molecular analysis of GogB, a phage-encoded type III-secreted substrate in Salmonella enterica serovar typhimurium with autonomous expression from its associated phage. J Mol Biol 2005; 348:817 - 830