Abstract
Contact-dependent growth inhibition (CDI) is the first contact-dependent competition system identified in bacteria. CDI is mediated by the CdiA/CdiB two-partner secretion system, and the BamA outer membrane protein serves as the CDI receptor on target cells. A small immunity protein, CdiI, is required to protect inhibitor cells from their own CDI system. Recent results from our group show that CDI systems are present in a number of important gram-negative plant and animal pathogens. The C-terminal region of CdiA (CdiA-CT) is polymorphic and contains growth inhibitory activity. The CdiA-CT from uropathogenic Esherichia coli 536 is a tRNase whereas a CdiA-CT from Dickeya dadantii 3937 has DNase activity. Accordingly, these bacteria contain distinct CdiI proteins, which specifically bind and inactivate cognate CdiA-CT. Remarkably, CdiA-CTs are modular: one CdiA "stick" can deliver different CdiA-CT toxins. We discuss these findings as well as results showing that CDI plays an important role in intra-strain bacterial competition in the natural world. A detailed mechanistic understanding of CDI could facilitate development of probiotics and antimicrobials that target specific pathogens.
Background
We first identified the contact-dependent growth inhibition (CDI) phenomenon in E. coli strain EC93, which was the predominant E. coli strain isolated from fecal pellets of a commercial rat colony.Citation1 This predominance suggested that the EC93 strain may have a competitive advantage over other E. coli strains in the rat intestine. Consistent with this hypothesis, we showed that EC93 has the ability to inhibit the growth of laboratory strains of E. coli when co-cultured in liquid media. Remarkably, this growth inhibition was not mediated by soluble factors and instead required direct cell-to-cell contact between EC93 inhibitor and target cells. Isolation of the cdi locus from EC93 revealed that only three genes—cdiB, cdiA and cdiI—are required to mediate CDI. Based on sequence homology, the CdiB and CdiA proteins are members of the two-partner secretion family. CdiB (64.5 kDa) is likely to be an outer membrane β-barrel protein involved in the secretion and assembly of CdiA onto the cell surface. CdiA is a large protein (319 kDa) with homology to filamentous hemagglutinin,Citation2 and is predicted to adopt an extended β-helical structure several hundred angstroms in length.Citation3 Thus, CdiA is thought to project from the inhibitor cell surface and interact with receptors on target cells. CdiI is a small peptide (8.9 kDa) that provides immunity to cells expressing CdiB and CdiA, and is required to prevent cell suicide.
The cdi locus is constitutively expressed in EC93 and CDI is a very effective system. A single EC93 cell is able to inhibit the growth of several hundred target cells within a few hours of co-culture.Citation1 EC93-mediated growth inhibition requires continuous protein synthesis in the inhibitor cell, but not in the target cell. Isolation of CDI-resistant (CDIR) mutants identified some of the components of the CDI pathway.Citation4,Citation5 These include the CDI receptor, BamA (previously named YaeT), an essential outer membrane protein and central component of the β-barrel assembly machine,Citation6 and AcrB, an inner membrane protein that functions with AcrA and TolC to export antibiotics and other small hydrophobic molecules.Citation7 Notably, the other components of the Bam and AcrB machines are not required for CDI.Citation5 Expression of CdiA in the absence of CdiI immunity function results in dissipation of the proton gradient and a concomitant decrease in respiration. AcrB-dependent export of ethidium bromide is also reduced upon induction of CdiA expression.Citation8 These results showed that cellular physiology was altered by CdiA, but it was unclear whether these effects account for growth inhibition during EC93-mediated CDI.
Recent Work
The recent work by our groups,Citation9 in collaboration with P. Cotter (University of North Carolina), has shed light on the CDI mechanism and its potential importance to bacterial biology. Deletion analysis showed that the C-terminal 250 residues of EC93 CdiA (CdiA-CTEC93) contains toxin activity. Sequence alignment of the CdiA proteins encoded by other CDI systems revealed a conserved peptide motif, (VE)NN, located approximately 200–250 residues from the C-terminus. The VENN motif demarcates a sharp transition from relatively conserved N-terminal sequences to polymorphic CdiA-CT sequences. The predicted CdiI proteins encoded by CDI systems are also polymorphic. These observations suggest that CdiA is a modular protein that can be loaded with a variety of toxic CdiA-CT domains. Indeed, functional CdiA chimeras can be produced by fusing heterologous CdiA-CTs onto the CdiA “stick,” which corresponds to the large filamentous region N-terminal to the VENN motif. The CdiA-CT from Yersinia pestis CO92 is readily delivered to target cells by the CdiA stick of uropathogenic E. coli strain 536 (UPEC 536). Immunity to the CdiAUPEC536-CTCO92 chimera is conferred by CdiI from Y. pestis CO92 and not from UPEC 536. These results indicate that CDI systems are functional in a number of bacterial species besides E. coli, that they are modular and interchangeable and that the CdiI immunity is specific to the CdiA-CT.
Further insight into the CDI mechanism came from analysis of CdiA-CT activities. The EC93 CdiA-CT does not appear to be soluble and its associated CdiI immunity protein is undetectable by immunoblotting using epitope tags (unpublished data). However, other CdiA-CTs were found to be soluble and their activities were identified. The UPEC 536 CdiA-CT was shown to have tRNase activity whereas the CdiA-CT23937 from D. dadantii 3937 has DNase activity. Further work has shown that several other CdiA-CTs have a variety of tRNase activities, and that RNase activity may be the most common inhibitory mechanism amongst CDI systems (manuscript in preparation). These results suggested the possibility that CdiI might confer immunity to growth inhibition by binding to the CdiA-CT and blocking its enzymatic activity. This was tested using nickel affinity chromatography of hexahistidine-tagged CdiI proteins mixed with cognate and non-cognate CdiA-CTs. These assays showed that each CdiI bound specifically to its cognate CdiA-CT, and that CdiI binding neutralized CdiA-CT nuclease activity. Although these results are clear for soluble CdiA-CTs, we do not know how CdiA-CTEC93, CdiA-CTCO92 and other insoluble CdiA-CTs act to inhibit cell growth. One possibility is that CdiA-CTEC93 forms a membrane pore, which is consistent with the observation that this CDI system causes a loss in the proton gradient. Additionally, the CdiIEC93 and CdiICO92 peptides are exceptionally hydrophobic and could be targeted to the inner membrane where they would block pore formation by binding to their cognate CdiA-CT. The molecular targets (DNA, RNA and lipid membranes) of CdiA-CTs are fundamental components of all cells, and these toxins could conceivably be deployed against a wide variety of bacterial species.
Raison d'etre of CDI
CDI systems were so named because although EC93 blocked the growth of target cells, there was no unequivocal evidence of cell killing. Target cells did not take up propidium iodide (a hallmark of cell death) and at least some of the targets appeared to recover after addition of an energy source.Citation8 This raised the possibility that CDI may function as a “viable but not culturable” phenomenon,Citation10 signaling neighbor cells to enter a quiescent state. However, it is possible that under normal physiological conditions the EC93 system would be toxic. AcrB function is blocked in EC93 inhibited cells, and this activity is required for resistance to the bile salts encountered by E. coli in its intestinal environment. In this instance, CDI may be used for competition with other E. coli or possibly other bacterial species in the intestines. We tested the hypothesis that CDI is used for intra-strain competition using E. coli EC93 and D. dadantii 3937, a well-studied plant pathogen that contains two CDI systems. E. coli EC93 lacking its CDI system was outcompeted over 100-fold by wild-type CDI+ EC93 in liquid medium. Deletion of the cdi-1 system (but not cdi-2) in D. dadantii 3937 reduced the ability of the mutant to compete with wild-type D. dadantii on chicory.Citation9 These results demonstrate that CDI systems function in intra-species competition, both in laboratory media and in natural environments. The reason why the D. dadantii 3937 cdi-2 system does not confer a growth advantage is not known, but could be due to lack of expression on chicory. Alternatively, the DNase toxin deployed by this system may target other bacteria besides Dickeya species. Many bacterial strains contain more than one cdi locus, suggesting that differential expression and/or target cell selection may be a common theme.
Are CDI systems active against other species? The D. dadantii 3937 cdi-1 system is expressed on plants but not laboratory media,Citation9 making it difficult to test its activity against E. coli. However, preliminary results suggest that the EC93 CDI system is not active against a few related enteric bacterial species including Salmonella enterica Typhimurium and Enterobacter aerogenes (unpublished data). This could be due to an evolutionary selection for competition solely with bacteria occupying the same niche, but further work needs to be done to assess the range of different CDI systems.
Speculations
The finding that CdiA-CTs possess toxic nuclease activities implies that these domains are translocated into the target cell cytosol after contact. This hypothesis predicts that CdiA-CT toxins are both necessary and sufficient for growth inhibition. The tRNase activity of CdiAUPEC536 is required for CDI (unpublished results), and we are currently testing other enzymatically inactive CdiAs to determine the effects on growth inhibition. Delivery of CdiA-CTs into cells is also consistent with our previous results indicating that protein synthesis is required and that one CDI+ cell can inhibit the growth of many target cells.Citation1 Once a CdiA-CT is delivered, the CdiA “stick” is presumably inactive, requiring new CdiA synthesis. It is yet unknown how many CdiA-CT molecules are required to inhibit target cell growth, but it is possible that only a few may be required because many of these toxins are enzymes.Citation9
CDI systems are present in a number of important animal (Moraxella catarrhalis), plant (Dickeya spp., Erwinia pyrifoliae, Pectobacterial spp.), and human pathogens including uropathogenic E. coli, enterohemorrhagic E. coli, Neisseria meningitides, Yersinia spp. including pestis, Klebsiella pneumoniae and Acinetobacter baumanii (). Based on our results with D. dadantii 3937, we speculate that CDI systems also play roles in growth competition in these pathogens. This could occur within a species (intra-strain) or between species (inter-strain). Thus far, we have only shown intra-species growth competition for CDI systems (). This is in contrast to recent results with Type VI secretion-associated systems, which appear to function in inter-species bacteria competition.Citation11,Citation12 The diverse collection of CdiA-CT domains and accompanying CdiI proteins found throughout the proteobacteria (), raises the question of what drives CDI diversity? Why evolve many distinct nucleases with different primary amino acid sequences? We think a likely possibility is that the acquisition of a novel CDI system would confer a growth advantage over other bacteria occupying the same niche. Of course, because cdi loci are very large, there is a fitness cost to this strategy. However, this penalty is presumably mitigated by tight control of CDI expression.
Future Research
CDI systems are the first contact-dependent growth inhibition systems described in bacteria. Although a few CdiA-CTs share homology with members of the colicin family (e.g., the CdiA-CT from Burkholderia pseudomallei K96243 and ColE5), they act very differently from colicins, requiring direct cell-to-cell contact for delivery. The mechanism(s) by which CdiA-CTs are cleaved from CdiA and presumably traverse the outer and inner membranes is not known. This could occur through contact-dependent autoproteolysis or cleavage by a target cell protein. It is possible that the translocation mechanism could be exploited to deliver other toxic peptides into bacteria. Very little is known about the regulation of cdi gene expression. Preliminary evidence suggests that the EC93 cdi gene cluster is under cAMP regulatory control because disruption of the cya gene downregulates the normally constitutive CDI activity. However, all other CDI systems we have studied have very low to no expression under standard laboratory conditions, and much work remains to determine how they are regulated. It is likely that different CDI systems will respond to different environmental cues, as has been found in D. dadantii 3937. In this case, we found that the cdi-1 cluster was active on chicory but inactive on rich or minimal media in the laboratory. For animal pathogens it seems likely that different cues will be sensed to activate CDI systems required for competition in the intestines (enteric bacteria), nasopharynx (meningococci), or possibly in arthropod vectors (Y. pestis). The roles of CDI systems in growth competition, both intra- and inter-species, need to be tested for these and other pathogenic bacteria. Such knowledge would illuminate the evolution of pathogens and might be useful in the design of probiotics and CDI inhibitors to prevent specific pathogens from outcompeting the normal flora.
Figures and Tables
Figure 1 Gram-negative pathogens encode polymorphic CdiA-CT domains. Multiple sequence alignment of the VENN junction region of CdiA proteins from Gram-negative pathogens. The VENN motif is shown in boldface and regions of sequence identity are indicated by underscoring and gray/black shading.
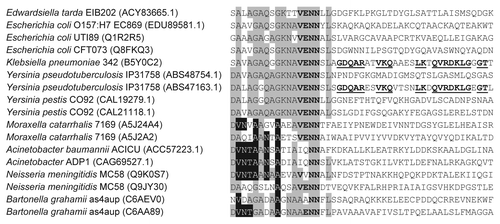
Figure 2 CDI plays roles in intra-strain growth competition. (1) CDI+ (olive and blue) and CDI− (gray) cells interact in environmental niches, such as plant leaves.Citation9 (2) CdiA contacts a neighboring cell and delivers the toxic CdiA-CT domain. (3) Growth is inhibited unless the target cell expresses the cognate CdiI immunity protein, which binds to and inactivates the toxin.

Acknowledgments
We thank A. Charkowski and R. Christoffersen for helpful advice. We acknowledge support from the National Science Foundation grant 0642052 (D.A.L.), a Tri-Counties Blood Bank Postdoctoral Fellowship (S.K.A.) and National Institutes of Health grants GM078634 (C.S.H.) and U54AI065359 (D.A.L. and C.S.H.). The content is the sole responsibility of the authors and does not necessarily represent the official views of the National Institute of Allergy and Infectious Diseases or the National Institutes of Health.
Addendum to:
References
- Aoki SK, Pamma R, Hernday AD, Bickham JE, Braaten BA, Low DA. Contact-dependent inhibition of growth in Escherichia coli. Science 2005; 309:1245 - 1248
- Mazar J, Cotter PA. New insight into the molecular mechanisms of two-partner secretion. Trends Microbiol 2007; 15:508 - 515
- Makhov AM, Hannah JH, Brennan MJ, Trus BL, Kocsis E, Conway JF, et al. Filamentous hemagglutinin of Bordetella pertussis. A bacterial adhesin formed as a 50-nm monomeric rigid rod based on a 19-residue repeat motif rich in beta strands and turns. J Mol Biol 1994; 241:110 - 124
- Hayes CS, Aoki SK, Low DA. Bacterial contact-dependent delivery systems. Annu Rev Genet 2010; 44:71 - 90
- Aoki SK, Malinverni JC, Jacoby K, Thomas B, Pamma R, Trinh BN, et al. Contact-dependent growth inhibition requires the essential outer membrane protein BamA (YaeT) as the receptor and the inner membrane transport protein AcrB. Mol Microbiol 2008; 70:323 - 340
- Kim S, Malinverni JC, Sliz P, Silhavy TJ, Harrison SC, Kahne D. Structure and function of an essential component of the outer membrane protein assembly machine. Science 2007; 317:961 - 964
- Nikaido H, Zgurskaya HI. AcrAB and related multidrug efflux pumps of Escherichia coli. J Mol Microbiol Biotechnol 2001; 3:215 - 218
- Aoki SK, Webb JS, Braaten BA, Low DA. Contact-dependent growth inhibition causes reversible metabolic downregulation in Escherichia coli. J Bacteriol 2009; 191:1777 - 1786
- Aoki SK, Diner EJ, de Roodenbeke CT, Burgess BR, Poole SJ, Braaten BA, et al. A widespread family of polymorphic contact-dependent toxin delivery systems in bacteria. Nature 468:439 - 442
- Hayes CS, Low DA. Signals of growth regulation in bacteria. Curr Opin Microbiol 2009; 12:667 - 673
- MacIntyre DL, Miyata ST, Kitaoka M, Pukatzki S. The Vibrio cholerae type VI secretion system displays antimicrobial properties. Proc Natl Acad Sci USA 107:19520 - 19254
- Schwarz S, West TE, Boyer F, Chiang WC, Carl MA, Hood RD, et al. Burkholderia type VI secretion systems have distinct roles in eukaryotic and bacterial cell interactions. PLoS Pathog 6:1001068