Abstract
Temperature change is a ubiquitous environmental signal, which exerts powerful control over the development and virulence of microbial pathogens. For Candida albicans, the leading fungal pathogen of humans, temperature influences mating, phenotypic switching, resistance to antifungal drugs and the morphogenetic transition from yeast to filamentous growth. C. albicans morphogenesis is profoundly influenced by temperature, and most filament-inducing cues depend on a concurrent increase in temperature to 37°C before morphogenesis can occur, although the molecular mechanisms underpinning this temperature-dependent developmental transition remain largely unknown. We established that the thermally responsive molecular chaperone Hsp90 orchestrates temperature-dependent morphogenesis, via previously uncharacterized cellular circuitry, comprised of the cyclin-dependent kinase Pho85, the cyclin Pcl1 and the transcriptional regulator Hms1. Here we elaborate on Hsp90's pleiotropic effects on temperature-dependent morphogenetic circuitry, and highlight how changes in protein form and function in response to stress complements the diverse repertoire of mechanisms of microbial temperature sensing.
All organisms must contend with environmental perturbations and fluctuations, including exposure to variations in temperature. The capacity to integrate temperature cues and respond appropriately is shared among organisms ranging from mammals to bacteria and fungi, and plays a particularly vital role for fungal pathogens. Of the estimated 5 million fungal species,Citation1 only approximately 150 are thought to be able to cause disease in endothermic mammalian species, and this can largely be attributed to the overwhelming majority of fungi losing their capacity for growth above ambient temperature.Citation2 The ability to sense and respond to temperature change is critical for fungal pathogens to thrive, and the circuitry governing responses to thermal stress is one of the most highly conserved protective responses in nature. Temperature can control fungal development and virulence, as illustrated by the dimorphic fungal pathogens for which a temperature-induced morphological transition is required for virulence. These dimorphic fungi, including Blastomyces dermatitidis, Coccidioides immitis and Histoplasma capsulatum, grow as filamentous molds in the soil at ambient temperature and convert to pathogenic yeast after infectious spores are inhaled into the lungs of a mammalian host.Citation3 Candida albicans, the leading fungal pathogen of humans, is an opportunistic pathogen that is frequently associated with its warm-blooded mammalian hosts, and has no known environmental reservoir.Citation4 Although C. albicans occupies a thermally buffered niche within its mammalian hosts, it retains a functional heat shock response,Citation5 and temperature fluctuations, which may be encountered through exposure to ambient temperature or during host febrile episodes, influence nearly all facets of C. albicans biology, including mating, phenotypic switching, resistance to antifungal drugs and morphogenesis.Citation6
Temperature fluctuations can induce morphogenetic change in C. albicans, which undergoes reversible transitions between yeast and filaments, including pseudohyphal and hyphal morphologies.Citation6 The capacity to transition between yeast and filamentous growth is strongly correlated with virulence, although there remain circumstances where these traits may be uncoupled.Citation7 Most C. albicans mutants locked in either yeast or filamentous forms are attenuated in virulence.Citation6 The transition from yeast to filamentous growth is induced by diverse environmental cues including serum, nutrient limitation, carbon dioxide and pH.Citation6 Many inducing cues, such as serum and carbon dioxide, depend on a concurrent increase in temperature to 37°C, and further elevated temperature of 39°C can serve as an independent inducing cue. The cellular response to morphogenetic cues is governed by complex signaling cascades.Citation6 Our previous work implicated the molecular chaperone Hsp90 as a central regulator of temperature-dependent morphogenesis.Citation8 We established that Hsp90 has pleiotropic effects on morphogenesis, such that it exerts a repressive effect on morphogenesis from yeast to filaments via distinct cellular signaling pathways, including the cAMP-protein kinase A (PKA) cascade (),Citation8 and cell cycle pathways mediated by the checkpoint protein Bub2 ().Citation9 Elevated temperature induces problems in protein folding and can overwhelm Hsp90 function, thereby relieving Hsp90-mediated repression of morphogenesis and inducing filamentation.Citation8 Notably, under certain conditions, C. albicans filamentation can occur at lower temperatures, including under embedded growth conditions,Citation10 and upon infection of the nematode Caenorhabditis elegans,Citation11 indicating that Hsp90 function may already be partially compromised or that Hsp90-independent pathways may mediate morphogenesis under these specific conditions.
Figure 1. Hsp90's pleiotropic effects on morphogenetic circuitry. (A) Hsp90 regulates temperature-dependent morphogenesis via negative regulation of the cAMP-PKA signaling cascade. (B) Hsp90 interacts with the cyclin-dependent kinase Cdc28 and controls morphogenesis via the mitotic exit network pathways mediated by the checkpoint protein Bub2. (C) Hsp90 orchestrates C. albicans temperature-dependent morphogenesis via novel circuitry comprised of the cyclin-dependent kinase Pho85, the cyclin Pcl1 and the transcriptional regulator Hms1.
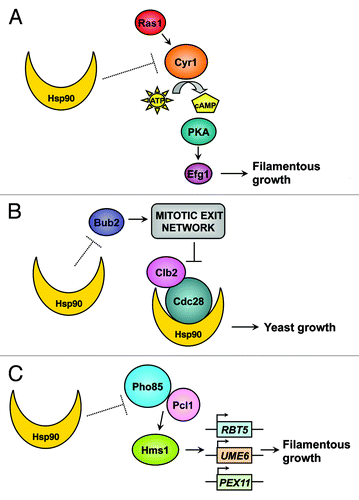
Most recently, we uncovered a new mechanism through which Hsp90 orchestrates C. albicans temperature-dependent morphogenesis, via the cyclin-dependent kinase Pho85, the cyclin Pcl1 and the transcriptional regulator Hms1 ().Citation12 Based on a genetic screen of transcription factor mutants to identify those blocked in the morphogenetic response to Hsp90 inhibition, we discovered a novel, uncharacterized transcriptional regulator, Hms1, which specifically governs filamentation induced by Hsp90 inhibition, as well as in response to elevated temperature stress.Citation12 Hms1 does not function downstream of the PKA signaling cascade, but rather, it is regulated by the cyclin Pcl1 and the cyclin-dependent kinase Pho85, both of which are also required for filamentation induced by compromised Hsp90 function or by temperature stress.Citation12 Upon inhibition of Hsp90, Hms1 is recruited to DNA elements associated with genes involved in filamentation, including the filament-specific transcripts UME6 and RBT5, and regulates their expression.Citation12 Consistent with the importance of morphogenesis for C. albicans virulence, deletion of HMS1 impairs temperature-dependent morphogenesis and attenuates virulence in an invertebrate model of C. albicans infection.Citation12
The discovery of the dependence of morphogenesis induced by elevated temperature or compromised Hsp90 on Pho85-Pcl1-Hms1 signaling highlights Hsp90's pleiotropic effects on morphogenetic circuitry (). The mechanisms by which Hsp90 represses Pho85-Pcl1-Hms1 signaling remain enigmatic, as are the mechanisms by which Hsp90 represses cAMP-PKA signaling, which is also required for morphogenesis induced by compromised Hsp90 function or by other stimuli such as serum.Citation8,Citation13 Filamentation induced by Hsp90 depletion is contingent on upstream signaling components of the cAMP-PKA pathway including the GTPase Ras1, the adenylyl Cyr1 and the PKA complex, but occurs independently of the terminal transcriptional regulator Efg1, implicating additional transcription factors in this process ().Citation8 Our screen of transcription factor mutants that identified Hms1 as crucial for filamentation induced by compromised Hsp90 function, also identified other transcriptional regulators required for filamentation induced by Hsp90 inhibition, including Cph2, Stp2 and Hap5.Citation12 It remains to be determined whether these transcription factors function in concert with known morphogenetic circuitry, or whether they operate within distinct signaling cascades yet to be implicated in regulation of morphogenesis. Notably, filaments induced by Hsp90 depletion also exhibit a delay in mitotic exit, which is mediated by the cell cycle checkpoint protein Bub2 (),Citation9 providing additional complexity to the circuitry through which Hsp90 controls temperature-dependent C. albicans morphogenesis. Hsp90’s pleiotropic effects on morphogenetic signaling could be mediated through direct effects on the activation of client proteins that it stabilizes or through more indirect effects. Given that Hsp90 interacts with ~10% of the proteome in S. cerevisiaeCitation14 and an equally complex network of interactors in C. albicans,Citation15 future studies are likely to reveal complex layers of regulatory control.
Our study uncovers the first specialized circuitry controlling temperature-dependent C. albicans morphogenesis, composed of the cyclin-dependent kinase Pho85, cyclin Pcl1 and transcription factor Hms1. Although none of these factors have been implicated in fungal morphogenesis in response to temperature stress, several have roles in morphogenetic programs in response to different environmental cues in other fungal species. In the model yeast Saccharomyces cerevisiae, the Hms1 ortholog regulates pseudohyphal growth in response to nitrogen limitation, as deletion of HMS1 reduces filamentation on solid synthetic low ammonia dextrose (SLAD) mediumCitation16 while overexpression of Hms1 target genes enhances pseudohyphal growth.Citation17 Further, in the filamentous fungus Aspergillus nidulans, the Pho85-like kinases PHOA and PHOB regulate polarized growth and sexual development in response to phosphate limitation.Citation18,Citation19 Expression of the Pho85 ortholog PhoSs fluctuates in response to calcium-mediated yeast to mycelium morphogenesis in the dimorphic fungal pathogen Sporothrix schenckii,Citation20 and the Pho85-related protein Cdk5 influences cell polarity and virulence and the corn smut fungus Ustilago maydis.Citation21 This suggests that, although these proteins play a conserved role in the morphogenetic transitions of diverse fungi, the cellular circuitry mediating these transitions can ultimately become rewired to respond to divergent environmental signals. Further analysis is required to elucidate the precise mechanism by which Hsp90 represses Pho85-Pcl1-Hms1 signaling. Hsp90 may bind to and directly inactivate one of the components of this pathway, or alternatively it may repress the pathway via an indirect mechanism. Hsp90 may directly regulate function of the cyclin-dependent kinase Pho85, as Hsp90, along with the co-chaperone Cdc37, regulates stability and function of numerous protein kinases, including several cyclin-dependent kinasesCitation22; and in S. cerevisiae, Cdc37 interacts with Pho85.Citation23 Thus, Hsp90 may regulate filamentation through interaction with Pho85 or other targets.
Hsp90's capacity to regulate a key developmental program in response to temperature change provides a new mechanism that complements the elegant repertoire that organisms utilize to sense temperature. Temperature sensing is key not only for survival of diverse fungal species faced by environmental challenge, but also for coordinating the induction of virulence traits in response to host physiological temperatures. Among the limited number of thermotolerant fungal species able to thrive within mammalian hosts, temperature plays a central role in their biology, and the ability to survive at elevated temperature (37°C) is crucial for the success of fungal pathogens. For the fungal pathogen Cryptococcus neoformans, mutants that are unable to grow in vitro at 37°C are avirulent in mouse models of infection,Citation24 and mutants that exhibit virulence defects are frequently found to have defects in growth at elevated temperature.Citation25 Several signaling factors involved in virulence, including Ras1 and calcineurin are dispensable for growth at ambient temperature, but required for growth at elevated host temperature, and consequently necessary for virulence of C. neoformans.Citation26,Citation27 For the filamentous fungal pathogen Aspergillus fumigatus, factors that confer thermotolerance may also contribute to virulence; for instance, the ribosome biogenesis protein CgrA has important roles in survival at elevated temperatures, growth, and virulence of A. fumigatus.Citation28 Similarly, the ability to grow at elevated temperatures up to 42°C is a characteristic that distinguishes clinical isolates of Saccharomyces cerevisiae from standard laboratory or industrial strains,Citation29 thus reinforcing the importance of thermotolerance in mediating fungal pathogenicity.
Temperature can influence developmental programs in fungal species, as well as virulence. This is most strikingly illustrated by the dimorphic fungal pathogens for which a temperature-induced morphological transition is required for virulence. These dimorphic fungi, including Blastomyces dermatitidis, Coccidioides immitis, Histoplasma capsulatum and Sporothrix schenckii, grow as filamentous molds in the soil at ambient temperature and convert to pathogenic yeast after infectious spores are exposed to elevated temperature upon inhalation into the lungs of a mammalian host.Citation3 In vitro, transitioning these fungi from ambient temperature to an elevated temperature of 37°C is sufficient to induce this morphogenetic switch.Citation3 In B. dermatitidis and H. capsulatum, the histidine kinase DRK1 functions as an environmental sensor of temperature, and controls morphogenesis, as well as adaptation to environmental stress within the mammalian host.Citation30 DRK1 is further required for the expression of virulence genes as well as fungal pathogenicity in vivo, reinforcing the relationship between fungal thermotolerance and virulence.Citation30 In H. capsulatum the Ryp family of proteins also play a key role in temperature-dependent morphogenesis,Citation31 and in S. schenckii, the calcium/calmodulin kinase SSCMK1 controls thermal tolerance as well as the dimorphic transition in response to growth at elevated temperature.Citation32
Despite its confinement within its endothermic mammalian host, C. albicans is responsive to fluctuations in temperature, and retains cellular signaling pathways associated with temperature response. Given that temperature controls the C. albicans morphogenetic transition between yeast and filamentous growth via circuitry comprised of Hsp90 and Pho85-Pcl1-Hms1 signaling, it is likely that these factors may influence other temperature-dependent traits in C. albicans or other fungal species. Temperature also governs another C. albicans cellular transition, phenotypic switching between the white and opaque cellular growth states.Citation33 At 37°C within the human host, C. albicans exists primarily as white cells, while opaque cells are more proficient at colonizing the skin, where host temperature is lower (~32°C).Citation34 When opaque cells are transitioned to elevated temperature, they rapidly convert to white cells, after approximately two cellular doublings,Citation33 although anaerobic conditions can stabilize the opaque state at 37°C.Citation35 Temperature also influences resistance to antifungal drugs, as elevated temperature abrogates fluconazole resistance of C. albicans clinical isolates.Citation36 Whether the Pho85-Pcl1-Hms1 circuitry has more global impact on the myriad of temperature-dependent traits in C. albicans, or whether it influences temperature-dependent developmental process in dimorphic fungi remains to be determined. Deciphering the molecular mechanisms underpinning these processes will reveal how fungi sense and respond to changes in temperature, which is crucial for the success of fungal pathogens.
Acknowledgments
R.S.S. is supported by a Natural Sciences and Engineering Research Council of Canada (NSERC) CGS-D Award and L.E.C. by a Career Award in the Biomedical Sciences from the Burroughs Wellcome Fund, by a Canada Research Chair in Microbial Genomics and Infectious Disease, and by CIHR Grant MOP-86452.
References
- Blackwell M. The fungi: 1, 2, 3 ... 5.1 million species?. Am J Bot 2011; 98:426 - 38; http://dx.doi.org/10.3732/ajb.1000298; PMID: 21613136
- Robert VA, Casadevall A. Vertebrate endothermy restricts most fungi as potential pathogens. J Infect Dis 2009; 200:1623 - 6; http://dx.doi.org/10.1086/644642; PMID: 19827944
- Klein BS, Tebbets B. Dimorphism and virulence in fungi. Curr Opin Microbiol 2007; 10:314 - 9; http://dx.doi.org/10.1016/j.mib.2007.04.002; PMID: 17719267
- Calderone R. Candida and Candidiasis. Washington, D.C.: ASM Press, 2002.
- Nicholls S, Leach MD, Priest CL, Brown AJ. Role of the heat shock transcription factor, Hsf1, in a major fungal pathogen that is obligately associated with warm-blooded animals. Mol Microbiol 2009; 74:844 - 61; http://dx.doi.org/10.1111/j.1365-2958.2009.06883.x; PMID: 19818013
- Shapiro RS, Robbins N, Cowen LE. Regulatory circuitry governing fungal development, drug resistance, and disease. Microbiol Mol Biol Rev 2011; 75:213 - 67; http://dx.doi.org/10.1128/MMBR.00045-10; PMID: 21646428
- Noble SM, French S, Kohn LA, Chen V, Johnson AD. Systematic screens of a Candida albicans homozygous deletion library decouple morphogenetic switching and pathogenicity. Nat Genet 2010; 42:590 - 8; http://dx.doi.org/10.1038/ng.605; PMID: 20543849
- Shapiro RS, Uppuluri P, Zaas AK, Collins C, Senn H, Perfect JR, et al. Hsp90 orchestrates temperature-dependent Candida albicans morphogenesis via Ras1-PKA signaling. Curr Biol 2009; 19:621 - 9; http://dx.doi.org/10.1016/j.cub.2009.03.017; PMID: 19327993
- Senn H, Shapiro RS, Cowen LE. Cdc28 provides a molecular link between Hsp90, morphogenesis, and cell cycle progression in Candida albicans. Mol Biol Cell 2012; 23:268 - 83; http://dx.doi.org/10.1091/mbc.E11-08-0729; PMID: 22090345
- Brown DH Jr., Giusani AD, Chen X, Kumamoto CA. Filamentous growth of Candida albicans in response to physical environmental cues and its regulation by the unique CZF1 gene. Mol Microbiol 1999; 34:651 - 62; http://dx.doi.org/10.1046/j.1365-2958.1999.01619.x; PMID: 10564506
- Pukkila-Worley R, Peleg AY, Tampakakis E, Mylonakis E. Candida albicans hyphal formation and virulence assessed using a Caenorhabditis elegans infection model. Eukaryot Cell 2009; 8:1750 - 8; http://dx.doi.org/10.1128/EC.00163-09; PMID: 19666778
- Shapiro RS, Sellam A, Tebbji F, Whiteway M, Nantel A, Cowen LE. Pho85, Pcl1, and Hms1 signaling governs Candida albicans morphogenesis induced by high temperature or Hsp90 compromise. Curr Biol 2012; 22:461 - 70; http://dx.doi.org/10.1016/j.cub.2012.01.062; PMID: 22365851
- Xu XL, Lee RT, Fang HM, Wang YM, Li R, Zou H, et al. Bacterial peptidoglycan triggers Candida albicans hyphal growth by directly activating the adenylyl cyclase Cyr1p. Cell Host Microbe 2008; 4:28 - 39; http://dx.doi.org/10.1016/j.chom.2008.05.014; PMID: 18621008
- Zhao R, Davey M, Hsu YC, Kaplanek P, Tong A, Parsons AB, et al. Navigating the chaperone network: an integrative map of physical and genetic interactions mediated by the Hsp90 chaperone. Cell 2005; 120:715 - 27; http://dx.doi.org/10.1016/j.cell.2004.12.024; PMID: 15766533
- Diezmann S, Michaut M, Shapiro RS, Bader GD, Cowen LE. Mapping the Hsp90 genetic interaction network in Candida albicans reveals environmental contingency and rewired circuitry. PLoS Genet 2012; 8:e1002562; http://dx.doi.org/10.1371/journal.pgen.1002562; PMID: 22438817
- Lorenz MC, Heitman J. Regulators of pseudohyphal differentiation in Saccharomyces cerevisiae identified through multicopy suppressor analysis in ammonium permease mutant strains. Genetics 1998; 150:1443 - 57; PMID: 9832522
- Chua G, Morris QD, Sopko R, Robinson MD, Ryan O, Chan ET, et al. Identifying transcription factor functions and targets by phenotypic activation. Proc Natl Acad Sci U S A 2006; 103:12045 - 50; http://dx.doi.org/10.1073/pnas.0605140103; PMID: 16880382
- Bussink HJ, Osmani SA. A cyclin-dependent kinase family member (PHOA) is required to link developmental fate to environmental conditions in Aspergillus nidulans. EMBO J 1998; 17:3990 - 4003; http://dx.doi.org/10.1093/emboj/17.14.3990; PMID: 9670015
- Dou X, Wu D, An W, Davies J, Hashmi SB, Ukil L, et al. The PHOA and PHOB cyclin-dependent kinases perform an essential function in Aspergillus nidulans. Genetics 2003; 165:1105 - 15; PMID: 14668368
- de Jesús-Berríos M, Rodríguez-del Valle N. Expression of a Pho85 cyclin-dependent kinase is repressed during the dimorphic transition in Sporothrix schenckii. Fungal Genet Biol 2002; 37:39 - 48; http://dx.doi.org/10.1016/S1087-1845(02)00031-2; PMID: 12223188
- Castillo-Lluva S, Alvarez-Tabarés I, Weber I, Steinberg G, Pérez-Martín J. Sustained cell polarity and virulence in the phytopathogenic fungus Ustilago maydis depends on an essential cyclin-dependent kinase from the Cdk5/Pho85 family. J Cell Sci 2007; 120:1584 - 95; http://dx.doi.org/10.1242/jcs.005314; PMID: 17405809
- Taipale M, Jarosz DF, Lindquist S. HSP90 at the hub of protein homeostasis: emerging mechanistic insights. Nat Rev Mol Cell Biol 2010; 11:515 - 28; http://dx.doi.org/10.1038/nrm2918; PMID: 20531426
- Mandal AK, Lee P, Chen JA, Nillegoda N, Heller A, DiStasio S, et al. Cdc37 has distinct roles in protein kinase quality control that protect nascent chains from degradation and promote posttranslational maturation. J Cell Biol 2007; 176:319 - 28; http://dx.doi.org/10.1083/jcb.200604106; PMID: 17242065
- Kwon-Chung KJ, Polacheck I, Popkin TJ. Melanin-lacking mutants of Cryptococcus neoformans and their virulence for mice. J Bacteriol 1982; 150:1414 - 21; PMID: 6804444
- Liu OW, Chun CD, Chow ED, Chen C, Madhani HD, Noble SM. Systematic genetic analysis of virulence in the human fungal pathogen Cryptococcus neoformans. Cell 2008; 135:174 - 88; http://dx.doi.org/10.1016/j.cell.2008.07.046; PMID: 18854164
- Alspaugh JA, Cavallo LM, Perfect JR, Heitman J. RAS1 regulates filamentation, mating and growth at high temperature of Cryptococcus neoformans. Mol Microbiol 2000; 36:352 - 65; http://dx.doi.org/10.1046/j.1365-2958.2000.01852.x; PMID: 10792722
- Odom A, Muir S, Lim E, Toffaletti DL, Perfect J, Heitman J. Calcineurin is required for virulence of Cryptococcus neoformans. EMBO J 1997; 16:2576 - 89; http://dx.doi.org/10.1093/emboj/16.10.2576; PMID: 9184205
- Bhabhra R, Richie DL, Kim HS, Nierman WC, Fortwendel J, Aris JP, et al. Impaired ribosome biogenesis disrupts the integration between morphogenesis and nuclear duplication during the germination of Aspergillus fumigatus. Eukaryot Cell 2008; 7:575 - 83; http://dx.doi.org/10.1128/EC.00412-07; PMID: 18296619
- McCusker JH, Clemons KV, Stevens DA, Davis RW. Saccharomyces cerevisiae virulence phenotype as determined with CD-1 mice is associated with the ability to grow at 42 degrees C and form pseudohyphae. Infect Immun 1994; 62:5447 - 55; PMID: 7960125
- Nemecek JC, Wüthrich M, Klein BS. Global control of dimorphism and virulence in fungi. Science 2006; 312:583 - 8; http://dx.doi.org/10.1126/science.1124105; PMID: 16645097
- Nguyen VQ, Sil A. Temperature-induced switch to the pathogenic yeast form of Histoplasma capsulatum requires Ryp1, a conserved transcriptional regulator. Proc Natl Acad Sci U S A 2008; 105:4880 - 5; http://dx.doi.org/10.1073/pnas.0710448105; PMID: 18339808
- Rodriguez-Caban J, Gonzalez-Velazquez W, Perez-Sanchez L, Gonzalez-Mendez R, Valle NR. Calcium/calmodulin kinase1 and its relation to thermotolerance and HSP90 in Sporothrix schenckii: an RNAi and yeast two-hybrid study. BMC Microbiol 2011; 11:162; http://dx.doi.org/10.1186/1471-2180-11-162; PMID: 21745372
- Lohse MB, Johnson AD. White-opaque switching in Candida albicans. Curr Opin Microbiol 2009; 12:650 - 4; http://dx.doi.org/10.1016/j.mib.2009.09.010; PMID: 19853498
- Lachke SA, Lockhart SR, Daniels KJ, Soll DR. Skin facilitates Candida albicans mating. Infect Immun 2003; 71:4970 - 6; http://dx.doi.org/10.1128/IAI.71.9.4970-4976.2003; PMID: 12933839
- Dumitru R, Navarathna DH, Semighini CP, Elowsky CG, Dumitru RV, Dignard D, et al. In vivo and in vitro anaerobic mating in Candida albicans. Eukaryot Cell 2007; 6:465 - 72; http://dx.doi.org/10.1128/EC.00316-06; PMID: 17259544
- Cowen LE, Lindquist S. Hsp90 potentiates the rapid evolution of new traits: drug resistance in diverse fungi. Science 2005; 309:2185 - 9; http://dx.doi.org/10.1126/science.1118370; PMID: 16195452