Abstract
Suppression during the early phases of the immune system often correlates directly with a fatal outcome for the host. The ebolaviruses, some of the most lethal viruses known, appear to cripple initial stages of the host defense network via multiple distinct paths. Two of the eight viral proteins are critical for immunosuppression. One of these proteins is VP35, which binds double-stranded RNA and antagonizes several antiviral signaling pathways.Citation1,Citation2 The other protein is VP24, which binds transporter molecules to prevent STAT1 translocation.Citation3 A more recent discovery is that VP24 also binds STAT1 directly,Citation4 suggesting that VP24 may operate in at least two separate branches of the interferon pathway. New crystal structures of VP24 derived from pathogenic and nonpathogenic ebolaviruses reveal its novel, pyramidal fold, upon which can be mapped sites required for virulence and for STAT1 binding. These structures of VP24, and new information about its direct binding to STAT1, provide avenues by which we may explore its many roles in the viral life cycle, and reasons for differences in pathogenesis among the ebolaviruses.
In The Art of War (6th century BCE), Sun Tzu wrote, “It is the rule in war: if ten times the enemy’s strength, surround them; if five times, attack them; if double, be able to divide them; if equal, engage them; if fewer, be able to evade them; if weaker, be able to avoid them.” The ebolaviruses are among the most lethal viruses known. Recent studies are revealing how these viruses encode multiple strategies to surround, attack, evade and/or avoid human immune defenses. Of course, the relative “strengths” of a virus and the host immune system vary by viral strain, host and environmental factors alike, and as a result, the interplay between these factors is multifactorial and complex.
The ebolaviruses and their cousins, the marburgviruses, are members of the filovirus family. These viruses are enveloped, non-segmented, negative-strand RNA viruses that cause severe hemorrhagic fever in both humans and nonhuman primates. Of the five antigenically distinct ebolaviruses, Ebola virus (EBOV; formerly known as Zaire ebolavirus) and Sudan virus (SUDV; formerly known as Sudan ebolavirus) are the most pathogenic, causing 50–90% lethality. Also among them, Reston virus (RESTV; formerly known as Reston ebolavirus) curiously is uniquely non-pathogenic to humans, although it is highly lethal to nonhuman primates. Microarray analyses performed on human cells suggest that RESTV has a reduced ability to suppress host cellular interferon (IFN) α/β and interferon (IFN) γ responses.Citation5
IFNα/β and IFNγ responses play key roles in protection of host cells against foreign invaders. Early suppression of cellular IFN production and signaling by key viral proteins serves as a critical turning point in the course of disease.Citation6,Citation7 VP24 is one of two proteins of the ebolaviruses known to antagonize IFN responsesCitation3; the other is VP35.Citation8 VP24 inhibits signaling downstream of both IFNα/β and IFNγ, by trapping karyopherin α proteins (α1, α5 and α6)Citation3 in the cytoplasm. Binding to these proteins prevents them from shuttling otherwise activated, phosphorylated STAT1 to the nucleus.Citation3,Citation9,Citation10 VP24 also prevents phosphorylation of p38 mitogen-activated protein (MAP) kinase,Citation11 thwarts the heterogeneous nuclear ribonuclear protein complex C1/C2 (hnRNP C1/C2, a complex primarily involved in host mRNA transcription) from relocating into the nucleus by karyopherin α proteins,Citation12 associates with host membranesCitation13,Citation14 and is required for assembly of the viral ribonucleoprotein complex.Citation15-Citation17 The second protein, VP35, blocks production of IFNα/βCitation18 by binding dsRNA, a trademark of viral infection, and shielding it from recognition by host immune sensors such as RIG-I and MDA-5.Citation19,Citation20 VP35 also inhibits phosphorylation of IRF3/7Citation21 by interacting with the kinase domain of TBK-1/IKKε,Citation22 suppresses secretion of tumor necrosis factor α,Citation23 suppresses RNA silencingCitation24 and is similarly required for assembly and function of the viral ribonucleoprotein complex.Citation1,Citation25
STAT1 belongs to the STAT family of transcription factors, plays key roles in immune signaling, and as a result, is a common target of viral proteins (for an excellent review, please see ref. Citation26). In a healthy cell, STAT1 predominately exists in an unphosphorylated form (U-STAT1). During viral infection, production of interferons and cytokines such as IFNα/β, IFNγ, tumor necrosis factor (TNF) α, IL-6 and IL-10 cause STAT1 to be phosphorylated (P-STAT1) by the Janus family kinases (JAKs). After activation by IFNα/β or IFNγ, P-STAT1 either forms a complex with STAT2 and IRF-9 or else P-STAT1 homodimerizes. Subsequently, these STAT1-containing complexes are transported to the nucleus by karyopherin α proteins where they regulate genes involved in the immune response.
Certain proteins of the Nipah and Hendra viruses, measles virus, vaccinia virus, Japanese encephalitis virus and mumps virus each antagonize the STAT1 complex by directly interacting with STAT1 at one or multiple points along its signaling pathway.Citation26 In contrast, the ebolavirus VP24 protein was shown to employ an alternative method and indirectly affect STAT1, by binding to karyopherin α1 and preventing it from translocating P-STAT1 to the nucleus.Citation3,Citation9,Citation10
Since multiple other viral proteins interact directly with STAT1 at several points along the STAT1 pathway, we wanted to know if VP24 could also bind STAT1 directly. We recently found that indeed, it does. By ELISA, we note that purified VP24 from EBOV and SUDV binds directly to purified STAT1 protein that is truncated prior to its phosphorylation site (STAT11–683).Citation4 Here, in this manuscript, we show that purified VP24 from Reston virus (RESTV) also binds to STAT1 at levels roughly equivalent to VP24 of EBOV and SUDV ().
Figure 1. VP24 key features. (A) Purified EBOV1–233 VP24, SUDV1–233 VP24 and RESTV11–237 VP24 bind to purified STAT11–683 by ELISA. VP24s were coated onto the ELISA plate at 0.02 mg/ml for overnight incubation at room temperature. STAT11–683 was incubated on the plates, binding was detected with HRP-conjugated secondary antibody and the resulting O.D. was read at 450 nm. BSA was used as a negative control. (B and C) The overall shape of VP24 resembles a three-sided pyramid with Faces 1 and 3 as illustrated. A hydrophobic cavity in Face 1 and a polar cavity in Face 3 are indicated by arrows. A ribbon model of SUDV1–233 VP24 illustrates the underlying secondary structural elements, while a surface representation of SUDV1–233 VP24 indicates the sequence conservation. Navy indicates residues that are absolutely conserved among the ebola- and marburgviruses, while red indicates residues that are least conserved among these viruses. Face 2 is the least conserved of the three faces and is not illustrated. Sequence identity is calculated using Homolmapper.Citation37 The accession codes for SUDV1–233, SUDV11–233 and RESTV11–237 are 3VNE, 3VNF and 4D9O, respectively.
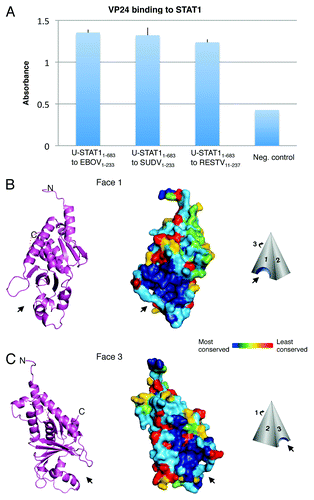
VP24 has no significant sequence homology to any other known protein and the molecular structure(s) and mechanism(s) by which it suppresses immune signaling and contributes to RNP assembly were not well understood. In order to illuminate the structural basis for its action, we determined X-ray crystal structures of VP24 from two different species within the Ebolavirus genus: Sudan virus, which is pathogenic to humans, and Reston virus, which is not pathogenic to humans. Two versions of VP24 from SUDV were crystallized: SUDV11–233 (which contains a ten-amino acid truncation from the N-terminus and a 18 amino acid truncation from the C-terminus to improve solubility) and subsequently SUDV1–233 (which contains only the C-terminal 18 amino acid truncation) (). One version of VP24 from RESTV was crystallized, RESTV11–237. VP24 from EBOV did not produce diffraction quality crystals, but was used in accompanying functional studies.
VP24 from all three constructs is a single domain, α/β structure with an overall shape resembling a triangular pyramid (). The three sides of the pyramid are termed Faces 1, 2 and 3. At the bottom of Faces 1 and 3 are located two pockets that are highly conserved across the filoviruses. The narrower of the two pockets is on Face 1 and is lined with a series of hydrophobic residues. The wider of the two pockets is on Face 3, is lined with polar residues and is shallower in depth. Although the functions of these conserved pockets remain elusive, they constitute tantalizing possibilities for anchor sites of the many viral and host binding partners of VP24.
Deuterium exchange mass spectrometry (DXMS), which measures the ability of polypeptide main-chain amide hydrogens to exchange hydrogen for solvent deuterium, allows mapping of protein footprints by identifying regions of a protein that exchange more slowly when complexed to a binding partner than when free. Using DXMS, we identified a putative area of interaction between VP24 and STAT11–683. Amino acids 96–98 and 106–121 of VP24 demonstrate slower H/D exchange kinetics when in complex with STAT-1, suggesting a site of protein-protein interaction. By contrast, amino acids 71–79 and 181–198 of VP24 demonstrate increased H/D exchange in the presence of STAT11–683, suggesting possible conformational change upon binding that results in enhanced flexibility of those regions. The slower-exchanging peptides, 96–98 and 106–121, are located at the top of the conserved portion of Face 3 (). The faster exchanging peptides, 71–79 and 181–198, map to the polar cavity at the bottom of Face 3. All of the faster- and slower-exchanging peptides, but 113–121 and 182–187, are conserved among both the ebola- and marburgviruses. Interestingly, these two sites are conserved among the ebolaviruses, but are different in the marburgviruses. They may be important sites as only VP24 from the ebolaviruses is immunosuppressive, not VP24 from the marburgviruses.Citation27,Citation28
Figure 2. Possible STAT-1 interaction sites and RESTV-specific differences. (A) Results from deuterium exchange mass spectrometry analysis are mapped onto the structure of SUDV1–233 VP24. Peptidic segments colored blue exhibit slower H/D exchange, while segments colored red exhibit increased H/D exchange when in the presence of STAT11–683. Segments colored white exhibit no change. Segments colored gray were not observed in these experiments. Experimental details can be found in Zhang et al.Citation4 (B) Sequence conservation among only ebolaviruses (not marburgviruses) mapped onto SUDV1–233 VP24. Circles indicate the sites of change in H/D exchange upon binding to STAT11–683. (C–E) Sites where RESTV encodes amino acids different from the SUDV and EBOV are mapped in red onto the structure of RESTV11–237. It is unclear which of these differences are important. We note that many exist on the unconserved Face 2. Others map to sites already shown to be important for virulence. A cyan circle on Faces 2 and 3 outlines a region of VP24 shown to interact with karyopherin α1 (cyan) that is flanked by RESTV-specific residues R139 and S140. Black ovals on Face 3 outline regions of faster or slower H/D exchange in the presence of STAT1. Inside these regions are RESTV-specific residues T116, L107, S184 and T185. Residue S50, which has been shown to increase virulence of EBOV in mice,Citation36 is located on Face 1. Green arrows indicate the location of the Face 1 and 3 cavities.
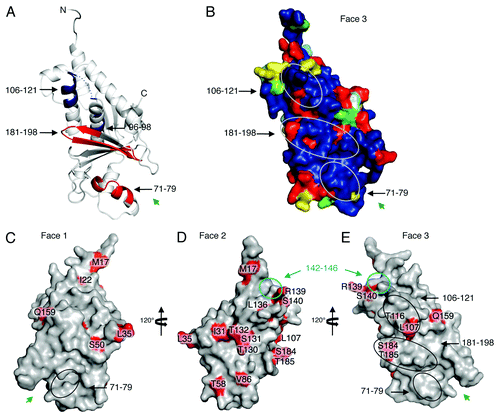
It is not yet clear if VP24 binds both phosphorylated STAT1 (P-STAT1) and unphosphorylated STAT1 (U-STAT1) in the context of viral infection (note that the purified STAT1–683 is unphosphorylated). However, binding and inhibition of either are likely important in immunosuppression. The significance of P-STAT1 to the immune response has been well established.Citation26 U-STAT1 is also important in regulation of the immune response as well, but it functions in different ways than its phosphorylated counterpart. For example, U-STAT1 is transported into the nucleusCitation29,Citation30 by direct interaction with nucleoporinsCitation31 and does not require transporter proteins as does P-STAT1. Once inside the nucleus, U-STAT1 activates and prolongs the expression of a number of IFN-induced immune regulatory genes like IFI27, IFI44, OAS and BST2.Citation32 Since U-STAT1 functions independently from P-STAT1, the set of genes on which U-STAT1 operates can be distinct from those of P-STAT1.Citation29 Further, U-STAT1 and P-STAT1 also differ temporally: the phosphorylation of STAT1 lasts for several hours but the presence of U-STAT1 persists for several days.Citation32,Citation33 In this way, U-STAT1 is likely to be able to prolong an antiviral state.
Hence, both P-STAT1 and U-STAT1 play multiple roles in antiviral defense and may play somewhat different roles in different cell types. By affecting both P-STAT1 (by binding karyopherins and/or possibly by forming a karyopherin-STAT1-VP24 tertiary complex) and U-STAT1 (if it binds full-length U-STAT1 as well as unphosphorylated STAT11–683), VP24 could prevent or dampen antiviral responses through multiple routes ().
Figure 3. Excerpts from the ebolavirus operation plan. (A) Upon interferon activation, STAT1 becomes phosphorylated by JAK and forms either an ISG3 complex (STAT1-STAT2-IRF9) or a homodimer. The STAT1 complex is then translocated to the nucleus by the carrier protein karyopherin α1. VP24 targets karyopherin α1 to sequester STAT1 in the cytoplasm. VP24 may also be able to target STAT1 directly (interactions shown here in dashed lines), although the downstream result of this binding is not yet known. Inset shows that VP24 also interferes in the MKK3/6-p38 mitogen-activated protein (MAP) kinase pathway,Citation11 by blocking IFNβ stimulated phosphorylation of p38-α. (B) The presence of double-stranded RNA is detected by the sensors RIG-I and MDA-5 that then trigger signaling of mitochondrial antiviral signaling proteins (MAVS), also known as virus-induced signaling adapter (VISA), IPS-1 and Cardif to activate Tank binding kinase-1 (TBK-1) and I-Kappa-B kinase epsilon (IKKε). With the aid of TNF receptor-associated factor 3 (TRAF3), activated TBK-1 and IKKε then phosphorylate interferon regulatory factor (IRF) 3 and IRF7. Upon phosphorylation, IRF3 and IRF7 homodimerize, translocate to the nucleus, and subsequently initiate production of type I IFNs. VP35 binds and masks dsRNA to prevent this signaling and downstream expression of IFNs. In addition, VP35 can also inhibit the phosphorylation of IRF3Citation21 by interacting with the kinase domain of IKKε22. In this way, both VP35 and VP24 may interfere at multiple steps in their respective pathways.
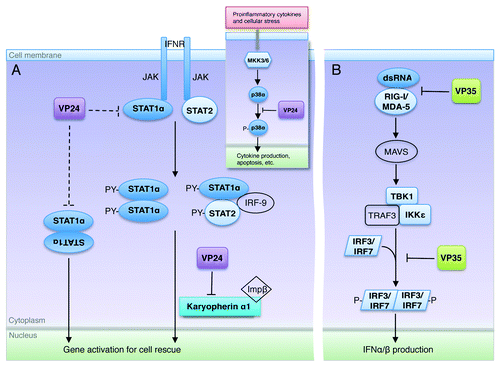
The combination of both ebolavirus VP24 and VP35 in the viral armamentarium offers greater coverage of the different pathways by which antiviral responses occur. It is interesting to note that plasmocytoid dendritic cells (pDCs), which are major producers of type I interferon,Citation34 are insensitive to VP35 inhibition.Citation23 It is conceivable that VP35 and VP24 exert a synergistic effect and/or VP24 functions in cells like pDCs where VP35 does not. Nevertheless, in tandem, VP24 and VP35 offer better coverage of immune signaling pathways that would be achievable by either of the proteins alone ().
A remaining, fascinating question is why RESTV is nonpathogenic toward humans, when the remaining ebolavirus species are pathogenic. The answer may be complex, as multiple host and viral factors (such as VP24 and VP35) are likely involved in modulating pathogenicity and that RESTV itself exhibits a reduced ability to suppress host immune responses.Citation5 The importance of VP24 to virulence suggests that some clues to the Reston-pathogenicity puzzle might lie in key amino acid differences between Reston and the pathogenic ebolaviruses (). In VP24, these sequence differences tend to either cluster around likely binding sites for karyopherin α1 or STAT1, or else, they lie in positions previously implicated in passage studies that conferred EBOV lethality to rodents. For example, one cluster of Reston-specific residues in VP24 is L136, R139 and S140. This cluster is located next to the 142–146 loop, thought to be important for binding karyopherin α1Citation10 and to residue L147, for which mutation confers virulence in guinea pig models of Ebola virus infection.Citation35 A second cluster of Reston-specific residues (L107 and T116) exists within the 106–121 polypeptide that exhibits decreased H/D exchange in the presence of STAT11–683. A third cluster of Reston-specific residues (S184 and T185) lies in the 181–198 polypeptide that exhibits increased H/D exchange in the presence of STAT11–683. Note that this cluster includes residues H186 and T187 for which mutation confers virulence of Ebola virus in guinea pig models.Citation35 A fourth site is the Reston-specific residue S50, which was also previously implicated in a different serial passage study to confer lethality of Ebola virus to mice.Citation36 It is not known which site(s) is/are responsible for the unique Reston virus phenotype, or even if these sites lie in VP24 or in VP24 alone. Note, however, that VP24 is a critical and common site for lethality-conferring mutations found in passage studies, and that as few as two or three amino acid changes is enough to confer lethality of Ebola virus to rodent models.Citation35,Citation36 Hence, these structures of RESTV and SUDV VP24 provide a three-dimensional framework by which we may begin to dissect differences between them.
New discoveries on the structure and function of VP24 have now illuminated a possible additional mechanism by which this protein suppresses the innate immune system in virus infection. This pathway is added to the list of other known anti-immune functions of VP24 and VP35, and suggests that these proteins likely work as a team to coordinate parallel schemes of immune silencing. Sun Tzu also wrote, “When the enemy has made a plan of attack against us, we must anticipate him by delivering our own attack first.” It is clear that pathogens like the ebolaviruses have developed a coordinated, multipronged strategy to defeat host immune molecules as or before they launch.
Acknowledgments
We thank Dr John M. Dye Jr for his gift of SUDV VP24 cDNA, Dr Viktor Volchkov for his gift of EBOV VP24 cDNA and Dr Christopher Basler (MSSM) for his gift of STAT1 cDNA. This work was supported by The Skaggs Institute for Chemical Biology (EOS), NIH Grants AI081982 and AI2008031 (E.O.S. and V.L.W.) and GM020501, GM066170, NS070899, GM093325 and RR029388 (V.L.W.). This is manuscript 21778 from The Scripps Research Institute. A.P.P.Z. was supported by NIH postdoctoral training grant 5P32 AI007244 to the TSRI Dept. of Immunology and Microbial Science.
References
- Leung DW, Prins KC, Basler CF, Amarasinghe GK. Ebolavirus VP35 is a multifunctional virulence factor. Virulence 2010; 1:526 - 31; http://dx.doi.org/10.4161/viru.1.6.12984; PMID: 21178490
- Hastie KM, Bale S, Kimberlin CR, Saphire EO. Hiding the evidence: two strategies for innate immune evasion by hemorrhagic fever viruses. Curr Opin Virol 2012; 2:151 - 6; http://dx.doi.org/10.1016/j.coviro.2012.01.003; PMID: 22482712
- Reid SP, Leung LW, Hartman AL, Martinez O, Shaw ML, Carbonnelle C, et al. Ebola virus VP24 binds karyopherin alpha1 and blocks STAT1 nuclear accumulation. J Virol 2006; 80:5156 - 67; http://dx.doi.org/10.1128/JVI.02349-05; PMID: 16698996
- Zhang AP, Bornholdt ZA, Liu T, Abelson DM, Lee DE, Li S, et al. The ebola virus interferon antagonist VP24 directly binds STAT1 and has a novel, pyramidal fold. PLoS Pathog 2012; 8:e1002550; http://dx.doi.org/10.1371/journal.ppat.1002550; PMID: 22383882
- Kash JC, Mühlberger E, Carter V, Grosch M, Perwitasari O, Proll SC, et al. Global suppression of the host antiviral response by Ebola- and Marburgviruses: increased antagonism of the type I interferon response is associated with enhanced virulence. J Virol 2006; 80:3009 - 20; http://dx.doi.org/10.1128/JVI.80.6.3009-3020.2006; PMID: 16501110
- Wauquier N, Becquart P, Padilla C, Baize S, Leroy EM. Human fatal zaire ebola virus infection is associated with an aberrant innate immunity and with massive lymphocyte apoptosis. PLoS Negl Trop Dis 2010; 4:e837; http://dx.doi.org/10.1371/journal.pntd.0000837; PMID: 20957152
- Baize S, Leroy EM, Georges-Courbot MC, Capron M, Lansoud-Soukate J, Debré P, et al. Defective humoral responses and extensive intravascular apoptosis are associated with fatal outcome in Ebola virus-infected patients. Nat Med 1999; 5:423 - 6; http://dx.doi.org/10.1038/7422; PMID: 10202932
- Basler CF, Wang X, Mühlberger E, Volchkov V, Paragas J, Klenk HD, et al. The Ebola virus VP35 protein functions as a type I IFN antagonist. Proc Natl Acad Sci U S A 2000; 97:12289 - 94; http://dx.doi.org/10.1073/pnas.220398297; PMID: 11027311
- Reid SP, Valmas C, Martinez O, Sanchez FM, Basler CF. Ebola virus VP24 proteins inhibit the interaction of NPI-1 subfamily karyopherin alpha proteins with activated STAT1. J Virol 2007; 81:13469 - 77; http://dx.doi.org/10.1128/JVI.01097-07; PMID: 17928350
- Mateo M, Reid SP, Leung LW, Basler CF, Volchkov VE. Ebolavirus VP24 binding to karyopherins is required for inhibition of interferon signaling. J Virol 2010; 84:1169 - 75; http://dx.doi.org/10.1128/JVI.01372-09; PMID: 19889762
- Halfmann P, Neumann G, Kawaoka Y. The Ebolavirus VP24 protein blocks phosphorylation of p38 mitogen-activated protein kinase. J Infect Dis 2011; 204:Suppl 3 S953 - 6; http://dx.doi.org/10.1093/infdis/jir325; PMID: 21987775
- Shabman RS, Gulcicek EE, Stone KL, Basler CF. The Ebola virus VP24 protein prevents hnRNP C1/C2 binding to karyopherin α1 and partially alters its nuclear import. J Infect Dis 2011; 204:Suppl 3 S904 - 10; http://dx.doi.org/10.1093/infdis/jir323; PMID: 21987768
- Bamberg S, Kolesnikova L, Möller P, Klenk HD, Becker S. VP24 of Marburg virus influences formation of infectious particles. J Virol 2005; 79:13421 - 33; http://dx.doi.org/10.1128/JVI.79.21.13421-13433.2005; PMID: 16227263
- Han Z, Boshra H, Sunyer JO, Zwiers SH, Paragas J, Harty RN. Biochemical and functional characterization of the Ebola virus VP24 protein: implications for a role in virus assembly and budding. J Virol 2003; 77:1793 - 800; http://dx.doi.org/10.1128/JVI.77.3.1793-1800.2003; PMID: 12525613
- Noda T, Halfmann P, Sagara H, Kawaoka Y. Regions in Ebola virus VP24 that are important for nucleocapsid formation. J Infect Dis 2007; 196:Suppl 2 S247 - 50; http://dx.doi.org/10.1086/520596; PMID: 17940956
- Watanabe S, Noda T, Halfmann P, Jasenosky L, Kawaoka Y. Ebola virus (EBOV) VP24 inhibits transcription and replication of the EBOV genome. J Infect Dis 2007; 196:Suppl 2 S284 - 90; http://dx.doi.org/10.1086/520582; PMID: 17940962
- Hoenen T, Jung S, Herwig A, Groseth A, Becker S. Both matrix proteins of Ebola virus contribute to the regulation of viral genome replication and transcription. Virology 2010; 403:56 - 66; http://dx.doi.org/10.1016/j.virol.2010.04.002; PMID: 20444481
- Cárdenas WB, Loo YM, Gale M Jr., Hartman AL, Kimberlin CR, Martínez-Sobrido L, et al. Ebola virus VP35 protein binds double-stranded RNA and inhibits alpha/beta interferon production induced by RIG-I signaling. J Virol 2006; 80:5168 - 78; http://dx.doi.org/10.1128/JVI.02199-05; PMID: 16698997
- Kimberlin CR, Bornholdt ZA, Li S, Woods VL Jr., MacRae IJ, Saphire EO. Ebolavirus VP35 uses a bimodal strategy to bind dsRNA for innate immune suppression. Proc Natl Acad Sci U S A 2010; 107:314 - 9; http://dx.doi.org/10.1073/pnas.0910547107; PMID: 20018665
- Leung DW, Prins KC, Borek DM, Farahbakhsh M, Tufariello JM, Ramanan P, et al. Structural basis for dsRNA recognition and interferon antagonism by Ebola VP35. Nat Struct Mol Biol 2010; 17:165 - 72; http://dx.doi.org/10.1038/nsmb.1765; PMID: 20081868
- Basler CF, Mikulasova A, Martinez-Sobrido L, Paragas J, Mühlberger E, Bray M, et al. The Ebola virus VP35 protein inhibits activation of interferon regulatory factor 3. J Virol 2003; 77:7945 - 56; http://dx.doi.org/10.1128/JVI.77.14.7945-7956.2003; PMID: 12829834
- Prins KC, Cárdenas WB, Basler CF. Ebola virus protein VP35 impairs the function of interferon regulatory factor-activating kinases IKKepsilon and TBK-1. J Virol 2009; 83:3069 - 77; http://dx.doi.org/10.1128/JVI.01875-08; PMID: 19153231
- Leung LW, Park MS, Martinez O, Valmas C, López CB, Basler CF. Ebolavirus VP35 suppresses IFN production from conventional but not plasmacytoid dendritic cells. Immunol Cell Biol 2011; 89:792 - 802; http://dx.doi.org/10.1038/icb.2010.169; PMID: 21263462
- Haasnoot J, de Vries W, Geutjes EJ, Prins M, de Haan P, Berkhout B. The Ebola virus VP35 protein is a suppressor of RNA silencing. PLoS Pathog 2007; 3:e86; http://dx.doi.org/10.1371/journal.ppat.0030086; PMID: 17590081
- Noda T, Aoyama K, Sagara H, Kida H, Kawaoka Y. Nucleocapsid-like structures of Ebola virus reconstructed using electron tomography. J Vet Med Sci 2005; 67:325 - 8; http://dx.doi.org/10.1292/jvms.67.325; PMID: 15805739
- Najjar I, Fagard R. STAT1 and pathogens, not a friendly relationship. Biochimie 2010; 92:425 - 44; http://dx.doi.org/10.1016/j.biochi.2010.02.009; PMID: 20159032
- Valmas C, Grosch MN, Schümann M, Olejnik J, Martinez O, Best SM, et al. Marburg virus evades interferon responses by a mechanism distinct from ebola virus. PLoS Pathog 2010; 6:e1000721; http://dx.doi.org/10.1371/journal.ppat.1000721; PMID: 20084112
- Valmas C, Basler CF. Marburg virus VP40 antagonizes interferon signaling in a species-specific manner. J Virol 2011; 85:4309 - 17; http://dx.doi.org/10.1128/JVI.02575-10; PMID: 21325424
- Chatterjee-Kishore M, Wright KL, Ting JP, Stark GR. How Stat1 mediates constitutive gene expression: a complex of unphosphorylated Stat1 and IRF1 supports transcription of the LMP2 gene. EMBO J 2000; 19:4111 - 22; http://dx.doi.org/10.1093/emboj/19.15.4111; PMID: 10921891
- Meyer T, Gavenis K, Vinkemeier U. Cell type-specific and tyrosine phosphorylation-independent nuclear presence of STAT1 and STAT3. Exp Cell Res 2002; 272:45 - 55; http://dx.doi.org/10.1006/excr.2001.5405; PMID: 11740864
- Meyer T, Vinkemeier U. Nucleocytoplasmic shuttling of STAT transcription factors. Eur J Biochem 2004; 271:4606 - 12; http://dx.doi.org/10.1111/j.1432-1033.2004.04423.x; PMID: 15606747
- Cheon H, Stark GR. Unphosphorylated STAT1 prolongs the expression of interferon-induced immune regulatory genes. Proc Natl Acad Sci U S A 2009; 106:9373 - 8; http://dx.doi.org/10.1073/pnas.0903487106; PMID: 19478064
- Lehtonen A, Matikainen S, Julkunen I. Interferons up-regulate STAT1, STAT2, and IRF family transcription factor gene expression in human peripheral blood mononuclear cells and macrophages. J Immunol 1997; 159:794 - 803; PMID: 9218597
- Fitzgerald-Bocarsly P. Human natural interferon-alpha producing cells. Pharmacol Ther 1993; 60:39 - 62; http://dx.doi.org/10.1016/0163-7258(93)90021-5; PMID: 8127923
- Volchkov VE, Chepurnov AA, Volchkova VA, Ternovoj VA, Klenk HD. Molecular characterization of guinea pig-adapted variants of Ebola virus. Virology 2000; 277:147 - 55; http://dx.doi.org/10.1006/viro.2000.0572; PMID: 11062045
- Ebihara H, Takada A, Kobasa D, Jones S, Neumann G, Theriault S, et al. Molecular determinants of Ebola virus virulence in mice. PLoS Pathog 2006; 2:e73; http://dx.doi.org/10.1371/journal.ppat.0020073; PMID: 16848640
- Rockwell NC, Lagarias JC. Flexible mapping of homology onto structure with homolmapper. BMC Bioinformatics 2007; 8:123; http://dx.doi.org/10.1186/1471-2105-8-123; PMID: 17428344