Abstract
Recent evidence suggests that loss of endothelial barrier function and resulting microvascular leak play important mechanistic roles in the pathogenesis of infection-related end-organ dysfunction and failure. Several distinct therapeutic strategies, designed to prevent or limit infection-related microvascular endothelial activation and permeability, thereby mitigating end-organ injury/dysfunction, have recently been investigated in pre-clinical models. In this review, these potential therapeutic strategies, namely, VEGFR2/Src antagonists, sphingosine-1-phosphate agonists, fibrinopeptide Bβ15–42, slit2N, secinH3, angiopoietin-1/tie-2 agonists, angiopoietin-2 antagonists, statins, atrial natriuretic peptide, and mesenchymal stromal (stem) cells, are discussed in terms of their translational potential for the management of clinical infectious diseases.
Introduction
Microvascular leak caused by compromised vascular barrier function plays an important role in the pathogenesis and disease progression for a range of infectious syndromes, including sepsis,Citation1 acute lung injury,Citation2 dengue hemorrhagic fever and shock syndrome,Citation3 viral hemorrhagic fevers,Citation4 and hantavirus pulmonary syndrome.Citation5 The main component of the vascular barrier is the endothelial cell monolayer, which is comprised of endothelial cells themselves and associated endothelial cell–cell junctions, including both adherens junctions and tight junctions, as well as a variety of extracellular components (e.g., the glycocalyx and the basement membrane).
Tight junctions, also referred to as zonula occludens, are predominantly composed of occludins and claudins, and are commonly located at the apical surface of the inter-endothelial cell cleft. Vascular surfaces that require tight regulation of endothelial cell permeability, such as the blood–brain barrier, are typically comprised of well-developed tight junctions. Adherens junctions are predominantly composed of vascular endothelial cadherin (VE-cadherin). VE-cadherin contains an extracellular domain that connects to adjacent endothelial cells and an intracellular domain that connects to the actin cytoskeleton via a family of catenins (α-, β-, γ-, and p120 catenins).Citation6 VE-cadherin is regulated by the Rho family of GTPases, including Rho1, Rac1, and Cdc42. Specifically, Rho mediates endothelial cell permeability and junction disassembly, while Rac enhances vascular endothelial cell barrier integrity.Citation7
The semipermeable endothelial barrier allows for transport of fluids and solutes from blood vessels into tissues. However, in pathological states, increased endothelial cell permeability results in excess transit of proteins and solutes between endothelial cells (paracellular leak), thereby causing edema. Such gaps in the vascular barrier are predominantly regulated by VE-cadherin,Citation8,Citation9 but may also be regulated by additional components of the adherens junctions, as well as modification of tight junctions and distortion of the endothelial cell structure due to cytoskeletal remodeling.
Recent evidence suggests that preventing microvascular leak may represent a viable therapeutic strategy to decrease infection-related end-organ injury/dysfunction in infectious diseases, thereby improving clinical outcome. A number of therapeutic strategies have emerged that are intended to strengthen vascular barrier integrity ( and ). The focus of this review is to summarize these emerging therapeutic strategies and highlight their reported effects in pre-clinical models of infectious diseases.
Table 1. Emerging microvascular barrier-enhancing agents
Figure 1. (A) Endothelial cell barrier dysfunction. During severe infections, endothelial activation and dysfunction may lead to the loss of microvascular endothelial barrier integrity, resulting in edema, multiple organ failure and death. Phosphorylation of adherens junction protein p120 catenin precipitates VE-cadherin internalization/junction disassembly. Endothelial integrity may also be compromised by rearrangements/degradation of the actin cytoskeleton. Molecular pathways implicated in this response include MYD88-ARNO binding that results in enhanced ARF6 signaling and decreased VE-cadherin localization at the cell surface. Endothelial activation may also cause an increase in the Ang-1 antagonist, Ang-2. Ang-2 binds to its cognate receptor, Tie2, and impedes the vascular stabilizing effects of Ang-1 by promoting proinflammatory endothelial responses and upregulating cell surface adhesion molecules. Vascular permeabilizing VEGF binds to VEGFR2 resulting in increased dissociation of VE-cadherin from the adherens junction through a VEGFR2-Src-VE-cadherin signaling pathway. (B) Endothelial cell barrier enhancement. SecinH3, a GEF (e.g., ARNO) inhibitor, inhibits ARF6-induced VE-cadherin internalization. Ang-1/Tie2 agonists activate Tie2 resulting in increased vascular quiescence via strengthening of endothelial cell junctions, downregulation of surface adhesion molecules and transdominate blockade of VEGR2 signaling. Fibrinopeptide Bβ15–42 provides barrier protection via maintenance of membrane VE-cadherin and inhibition of actin degradation via RhoA signaling inhibition. Upon binding its receptor Robo4, Slit2N reduces p120 catenin phosphorylation and inhibits ARF6, thereby increasing VE-cadherin retention at the cell surface. ANP administration decreases microvascular permeability via inhibition of RhoA-induced actin degradation and NFκB/P38 MAPK inhibition. S1P agonist administration reinforces the endothelial barrier via Rac1 and αvβ3 integrin signaling, resulting in formation and stabilization of cortical actin. Administration of S1P agonist enhances cortical actin formation (via Rac1 and αvβ3 integrin signaling) and downregulates IFN-α, thereby decreasing cytokine/chemokine production and enhancing endothelial cell barrier stability.
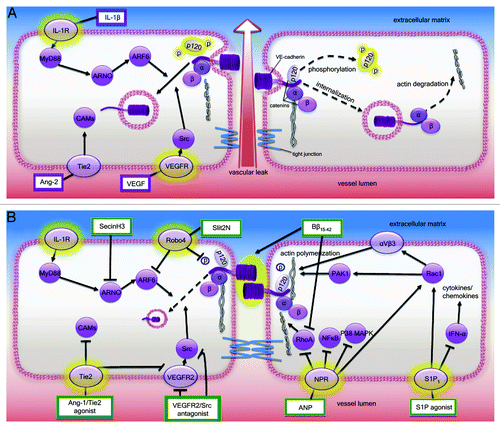
VEGFR2/Src Antagonists
Vascular endothelial growth factor (VEGF) is a glycoprotein that is generated and released by endothelial cells, lung epithelial cells, platelets, and leukocytes. VEGF is a well-established regulator of vascular permeability and exerts its effects through binding endothelial cell-specific membrane tyrosine kinase receptors, VEGFR1–3.Citation10 Upon activation by its cognate ligand, VEGFR2 increases vascular permeability by promoting dissociation of VE-cadherin from the adherens junction through a VEGFR2-Src-VE-cadherin signaling pathway.Citation11,Citation12
In sepsis, elevated plasma soluble VEGFR1 (sVEGFR1) levels have been reported to predict 28-d mortality and multi-organ dysfunction.Citation13 It has been hypothesized that increased production of sVEGFR2 promotes binding and neutralization of VEGF, thereby strengthening the endothelial barrier. However, the precise function of VEGF in sepsis is controversial, with studies implicating contrasting roles for VEGF in the pathophysiology of sepsis.Citation13-Citation17 An ongoing clinical trial evaluating the effects of a neutralizing anti-VEGF antibody (bevacizumab) in patients with septic shock should provide further insight regarding the potential use of VEGF-targeted therapeutics in sepsis.Citation18
Targeting the VEGF pathway has also been of interest in the treatment of viral hemorrhagic fever syndromes, including hantavirus-induced hemorrhagic fever with renal syndrome and hantavirus pulmonary syndrome. Administration of inhibitory VEGFR2 antibody decreased hantavirus-directed endothelial cell permeability in vitroCitation19 via inhibition of VEGF-induced VE-cadherin internalization.Citation5 Similarly, pazopanib and dasatinib, FDA-approved inhibitors of VEGFR2 and Src family kinases, respectively, decreased endothelial permeability induced by pathogenic hantavirus in vitro, via inhibition of VE-cadherin internalization.Citation20 These results suggest the possibility that targeting the VEGF pathway may be a therapeutic strategy for multiple infectious diseases characterized by endothelial barrier disruption.
Sphingosine-1-Phosphate (S1P) Agonists
Sphingosine-1-phosphate (S1P) is a signaling sphingolipid that is released from platelets and binds to G-protein-coupled S1P receptors, S1P1–5 (formerly known as Edg1–5).Citation21-Citation25 Plasma S1P contributes to the maintenance of microvascular integrity by signaling through SIP1 on endothelial cells.Citation26 S1P1 signals through the Rho family GTPase Rac, which activates αvβ3 integrins, to increase cortical actin formation, thereby enhancing stability of the endothelial cytoskeleton.Citation27,Citation28 Decreased plasma S1P has been demonstrated in several pathological conditions associated with vascular barrier dysfunction and microvascular leak, including cerebral malaria in children.Citation29
Protease-activated receptor-1 (PAR-1) is an important mediator of S1P1 signaling that contributes to both endothelial barrier stability and dysfunction.Citation30 PAR-1 activation by the serine protease thrombin can lead to increased endothelial cell permeability, while conversely, PAR-1 activation by activated protein C (APC) can lead to endothelial cell barrier protection.Citation30 This finding suggests that APC may serve as an effective endothelial stabilizing therapeutic agent. However, in a murine model of hyperoxic lung injury, prophylactic or therapeutic administration of recombinant murine APC was unable to ameliorate lung injury.Citation31 More importantly, several clinical trials in patients with severe sepsis or septic shock failed to demonstrate a therapeutic benefit of recombinant human APC on 28-d mortality, including one study in which recombinant human APC administration was associated with a higher risk of bleeding.Citation32-Citation36 These studies precipitated the withdrawal of recombinant human APC (drotecogin alfa) from the worldwide market.Citation36 A modified APC variant with minimal anticoagulant activity but preserved cell signaling functionality (5A-APC) was capable of reducing mortality by approximately 40% after bacterial infection or LPS challenge in murine models of sepsis,Citation37 suggesting further testing of anticoagulant APC variants may be of interest.
Administration of S1P or pharmacological analogs has been demonstrated to preserve or enhance vascular integrity in a number of infectious diseases in which microvascular leak plays an important pathologic role. In vitro, addition of S1P to Andes virus (hantavirus)-infected cells blocked VE-cadherin internalization in response to VEGF, thereby increasing endothelial integrity.Citation20 S1P administration has also been shown to stabilize the microvascular endothelium in several pre-clinical animal models. In a murine model of ventilator-induced lung injury and a canine model of LPS-induced ventilator associated acute lung injury, S1P administration attenuated lung vascular leak as documented by decreased Evans blue dye extravasation in the lung, decreased protein level in bronchoalveolar lavage fluid and decreased lung tissue volume (i.e., decreased “wet-to-dry” lung weight).Citation38 Furthermore, S1P antagonism has been reported to increase vascular leak in vivo under physiological conditions.Citation39
Recently, the pharmacologic agent FTY720, a potent S1P receptor agonistCitation40,Citation41 licensed as an experimental drug (Gilenya™) by the FDA, was evaluated in phase III clinical trials of multiple sclerosis.Citation42,Citation43 Phosphorylated FTY720 (FTY720-P) and its analog (R)-AAL ([R]-AFD) exert similar effects to S1P. In vitro, both agents induced β-catenin and localization of VE-cadherin to adherens junctions, as well as antagonized VEGF-induced endothelial cell permeability.Citation44 Similar effects were confirmed in vivo in a murine model of VEGF-induced vascular leak.Citation44
In a murine model of influenza, (R)-AAL administration one hour after influenza virus inoculation decreased pulmonary edema and inflammation.Citation45 Notably, (R)-AAL therapy exerted a greater therapeutic benefit than oseltamivir, the most widely used antiviral drug for the specific treatment of influenza. Furthermore, administration of (R)-AAL in combination with oseltamivir provided additional benefit over (R)-AAL administration alone. A follow-up study by the same group demonstrated that the benefits of S1P agonist administration ([R]-AAL, CYM-5442, or RP-002) were due to its ability to downregulate excessive cytokine/chemokine production by the host, an immunopathological feature of severe influenza disease, in response to decreased IFN-α production, an upstream regulator of early cytokine production.Citation46 In a murine model of cerebral malaria, FTY720 was also shown to improve clinical outcome when administered therapeutically, either alone or as an adjunctive therapy in combination with the anti-malarial drug artesunate.Citation29 This effect was attributed to increased integrity of the blood-brain barrier and enhanced endothelial stability, demonstrated by decreased Evans blue extravasation in the brain, reduced plasma sICAM-1 (a marker of endothelial activation), and increased angiopoietin-1 (a marker of endothelial stability).Citation29
In contrast, Puneet et al.Citation47 reported that blockade of sphingosine kinase 1 (sphk1) protected mice from experimental sepsis by enhancing bacterial clearance without altering systemic S1P levels required to maintain vascular barrier integrity. Intriguingly, because S1P receptor stimulation can lead to subsequent receptor downregulation, S1P agonists can potentially serve as functional S1P1 antagonists in certain circumstances.Citation48 Thus, it is likely that S1P-targeted agents can exert both deleterious and beneficial effects depending on the contextual pathophysiological state. Taken together, current pre-clinical evidence warrants further evaluation of Sphk1/S1P modifying therapeutic agents in the treatment of infectious disorders associated with excessive cytokine production and consequent microvascular leak, such as influenza and sepsis.
Fibrinopeptide Bβ15–42
The fibrin N-terminal peptide Bβ15–42 is a 28 amino acid cleavage product of fibrin that binds VE-cadherin and stabilizes interendothelial junctions.Citation49,Citation50 Because of its endothelial barrier stabilizing properties, the therapeutic use of fibrinopeptide Bβ15–42 (also known as FX06) has recently been investigated. In murine models of vascular leak, including pneumonitis and shock (intranasal LPS and intravenous LPS administration, respectively), FX06 administration attenuated capillary leak in the lungs.Citation51 In addition, FX06 administration improved survival by approximately 40% in a murine model of dengue shock.Citation51 These effects were mediated by FX06-induced dissociation of the Src kinase Fyn from VE-cadherin, in parallel with Fyn association with p190RhoGAP, a RhoA antagonist. In a murine model of polymicrobial sepsis (cecal ligation and puncture), treatment with FX06 attenuated leukocyte infiltration and reduced proinflammatory cytokines in the lung, liver, and blood.Citation52 Decreased tissue inflammation was attributed to FX06-sustained vascular integrity, thereby suppressing vascular leakage and subsequent inflammatory cell trafficking into the lungs. In support of this hypothesis, FX06 pretreatment of macrophages and endothelial cells was unable to reduce TLR2- and TLR4- induced inflammation; however, microvascular permeability was not specifically investigated in this study.Citation52 These data suggest that FX06 therapy may represent a novel and effective adjunctive therapy to increase vascular stability, thereby preventing end-organ inflammation, edema, and dysfunction in disorders associated with vascular activation/dysfunction and microvascular leak.
Slit2N
Binding of the ligand Slit to its cognate endothelial receptor Robo4 inhibits inflammation-induced endothelial permeability by strengthening adherens junctions and modulating cytoskeletal dynamics.Citation53-Citation56 Endothelial cell monolayer permeability induced by several mediators in vitro, including VEGF, LPS, TNF, and IL-1β, was counteracted by treatment with Slit2N, the active fragment of Slit.Citation54,Citation55 This effect correlated with increased cell surface expression of VE-cadherin. Specifically, Slit2N increased p120-catenin-VE-cadherin association by reducing p120-catenin phosphorylation.Citation55 Slit2N-Robo4 signaling may also increase cell surface VE-cadherin via inhibition of ARF6 signaling.Citation54,Citation57 These findings were substantiated in several experimental models of infectious diseases characterized by microvascular activation/dysfunction, including sepsis, LPS-induced lung injury, and H5N1 avian influenza. In each of these pre-clinical models, administration of Slit2N enhanced microvasculature integrity and improved survival without dampening inflammation (or altering viral load in H5N1 experimental avian influenza).Citation55 These findings suggest that therapeutic targeting of Robo receptors represents a promising strategy to improve clinical outcome in infectious diseases associated with endothelial dysregulation and microvascular leak.
SecinH3
Recently, Zhu et al.Citation57 described a novel cytokine-mediated pathway involved in endothelial barrier stability that functions independently of MYD88-induced NFκB signaling. In vitro, various NFκB pathway inhibitors failed to rescue IL-1β-induced endothelial permeability or endothelial cell surface VE-cadherin internalization. The results from a series of elegant experiments demonstrated a requirement for the adaptor protein MYD88 in IL-1β induced endothelial permeability, suggesting that MYD88 mediates a distinct NFκB-independent pathway involved in endothelial activation/dysfunction.Citation57 The investigators implicated a pathway involving MYD88 activation of the ARF guanine nucleotide-exchange factor inhibitor (GEF) ARNO, based on the observations that ARNO-ARF6 signaling decreased cell surface VE-cadherin and increased vascular permeability. Furthermore, administration of SecinH3, a GEF inhibitor, restored endothelial barrier function in murine models of inflammatory arthritis and acute inflammation, without affecting global cytokine expression.Citation57 SecinH3 administration has also been shown to decrease endothelial leak in a murine model of vascular eye disease.Citation54 Therapeutic agents that target the ARNO-ARF6 pathway, such as Slit2N and SecinH3, are emerging as important potential therapies for conditions associated with vascular destabilization.
Angiopoietin-1/Tie-2 Agonists
Angiopoietin-1 (Ang-1) is a ligand for the endothelial-specific receptor tyrosine kinase Tie2,Citation58 a potent mediator of angiogenesis that functions post-development to prevent vascular leakage and promote vascular quiescence via strengthening of endothelial cell junctions and downregulation of surface adhesion molecules, such as vascular cell adhesion molecule-1 (VCAM-1) and E-selectin.Citation59-Citation62 The Ang-1/Tie2 axis also transdominantly blocks VEGFR2-mediated microvascular permeability.Citation63 A variety of Ang-1/Tie2 targeted strategies have been investigated in pre-clinical models to reduce complications of infectious diseases. Both cell- and viral vector-based Ang-1 gene therapeutic strategies, including Ang-1 specifically engineered to potently induce Tie2 phosphorylation,Citation64 have been reported to reduce microvascular leak in murine models of acute lung injury and acute kidney injury.Citation2,Citation64-Citation67
A synthetic Tie2 agonist peptide known as vasculotide (VT) has been shown to protect against vascular leak and mortality, with subsequent improvement in end-organ function, when administered in either a prophylactic or therapeutic regimen in a murine model of polymicrobial sepsis.Citation68 VT has also been shown to prevent lung vascular leak and improve survival by 30–40% in a murine model of LPS-induced lung injury (intraperitoneal LPS administration).Citation69 Another study by Alfieri et al.Citation70 evaluated the effects of an Ang-1 mimetic, MAT.Ang-1, in experimental LPS-induced sepsis in mice. Therapeutic administration of a single dose of MAT.Ang.1, 20 h after LPS administration, decreased vascular leak without altering vascular resistance. A growing number of Ang-1/Tie2 agonists represent promising agents for the prevention or amelioration of infection-induced endothelial activation/dysfunction and consequential microvascular leak.
Angiopoietin-2 Antagonists
Angiopoietin-2 (Ang-2), a functional Ang-1 antagonist that binds to Tie2, counterbalances the vascular stabilizing effects of Ang-1 by promoting proinflammatory endothelial responses and disrupting endothelial barrier function.Citation71,Citation72 Current evidence suggests that the overall status of Tie2 phosphorylation is largely controlled by the relative ratio of Ang-1:Ang-2. Elevated Ang-2 levels have been reported in septic patients and have been associated with increased vascular leak, severity of illness and adverse outcomes.Citation71,Citation73-Citation75
In both cecal ligation and puncture (CLP)- and LPS-induced murine models of sepsis, Ang-2+/− heterozygous mice were relatively protected against vascular leak, acute lung injury, acute kidney injury and death compared with wild-type littermate controls.Citation75 Notably, this study also reported elevated circulating levels of Ang-2 in a cohort of patients with sepsis within one hour of their presentation to the emergency department. The level of Ang-2 elevation correlated with disease severity and predicted subsequent development of shock or death. Serum from affected patients disrupted endothelial barrier function in vitro, via a mechanism inhibited by treatment with an antagonistic Ang-2 monoclonal antibody. These findings suggest that Ang-2 upregulation occurs early in the course of sepsis and precedes the development of end-organ dysfunction. In contrast, recombinant Ang-2 increased survival when administered two hours prior to Escherichia coli-induced murine experimental sepsis or 30 min after Pseudomonas aeruginosa-induced murine experimental sepsis.Citation76 However, it is possible that circulating Ang-2 levels in experimental mice exceeded the physiological concentration of Ang-2 in patients with septic shock, a finding which has previously been associated with increased Tie2 phosphorylation.Citation77 Overall, these studies suggest that Ang-2 inhibition strategies merit further investigation for the treatment of sepsis.
Statins
HMG-CoA reductase inhibitors, commonly known as statins, are widely used for the treatment of hyperlipidemia and ischemic heart disease.Citation78 Based on recently recognized anti-inflammatory properties, statins have received increasing interest for their potential role(s) in the treatment of inflammation-related diseases.Citation79-Citation81 These effects include modulation of endothelial cell adhesion molecule expression (including downregulation of P-selectinCitation82 and ICAM-1Citation83), downregulation of NFκB-dependent gene expressionCitation84 and decreased mononuclear inflammatory cell infiltrates in target tissues.Citation85
Preliminary evidence suggests that prolonged statin use may decrease endothelial activation and improve outcomes in experimental bacterial pneumonia.Citation85 Mice receiving high dose simvastatin for several weeks prior to infection developed less bacteremia and inflammatory cell infiltrates in the lung, which were associated with decreased expression of MCP-1 and ICAM-1.Citation85 However, overall mortality was similar between simvastatin-treated animals and controls.
Atrial Natriuretic Peptide
Atrial natriuretic peptide (ANP) plays an important physiological role in the maintenance of arterial blood pressure and volume. These effects are mediated by binding to natriuretic peptide receptors (NPR) A–C. NPRs are guanylyl cyclase-linked, regulated by cGMP synthesis, and are highly expressed in the vascular endothelium. Vascular endothelial-specific NPR-A knockout mice developed systemic hypertension and cardiac hypertrophy, yet maintained a direct vasodilatory response to ANP.Citation86 Pre-treatment with ANP reduced paracellular endothelial gaps and stabilized VE-cadherin in TNF-activated endothelial cells.Citation87 These observations suggest that ANP moderates arterial blood pressure and volume via its effects on vascular permeability.
ANP modulates signaling pathways important for the production of proinflammatory cytokines and remodeling of the endothelial cell cytoskeleton. In vitro, endothelial cells pre-treated with ANP followed by LPS stimulation displayed significantly attenuated p38 MAPK, NFκB, and Rho-dependent signaling 6 h post-LPS stimulation.Citation88 In a murine model of LPS-induced lung injury, ANP modulated vasculature stability via increased Rac-dependent p21-activated kinase (PAK1) phosphorylation, resulting in increased endothelial cell barrier integrity.Citation87 PAK1 is a cytoskeletal Rac effector protein that initiates peripheral actin polymerization.Citation89
Increased levels of ANP have been observed in patients with septic shock,Citation90 and in murine models of lung injury.Citation87 Moreover, ANP−/− mice have been reported to develop more severe LPS-induced lung injury and vascular leak compared with wild-type mice,Citation87 suggesting a potential mechanistic role for ANP in sepsis and acute lung injury. Experimental acute lung injury-associated pulmonary edema induced by multiple stimuli—including thrombin, VEGF, LPS, peptidoglycan, and lipoteichoic acid—is reduced by concomitant or prophylactic ANP administration.Citation87,Citation88,Citation91-Citation94
Evaluation of therapeutic ANP administration in patients with lung injury is limited. In a cohort of 40 individuals with acute lung injury requiring mechanical ventilation with positive end-expiratory pressure (PEEP), ANP administration improved lung injury and oxygenation.Citation95 In contrast, Bindels et al.Citation96 observed no difference in pulmonary gas exchange in ten patients with acute respiratory distress syndrome who received ANP. Large, randomized controlled trials are warranted to evaluate the use of exogenous ANP as a potential therapeutic agent for the treatment of infection-induced lung injury.
Mesenchymal Stromal (Stem) Cells
Mesenchymal stromal (stem) cells (MSCs) represent a heterogenous subset of non-hematopoeitic pluripotent stromal cells with multi-lineage potential that can be isolated from various tissues (e.g., adult bone marrow).Citation97 While initial interest in MSCs was focused on their potential in regenerative medicine, a growing interest in their potential in immunomodulatory therapy has evolved based on their capacity to modulate the host response in diseases and syndromes associated with inflammation. It has been recently recognized that MSCs secrete an array of growth factors, cytokines, and lipid mediators that modulate host inflammation, improve pulmonary alveolar fluid clearance, and strengthen endothelial integrity thereby improving organ function and decreasing mortality in pre-clinical models of infectious diseases including LPS-induced acute lung injury and sepsis following cecal ligation and puncture.Citation2,Citation98-Citation106
MSC-mediated effects on the vascular endothelium have been investigated in vitro and in vivo. VEGF-treated pulmonary endothelial cells exposed to MSC-conditioned media preserved adherens junctions (β-catenin and VE-cadherin) leading to increased endothelial barrier stability.Citation106 This finding was confirmed in a rat model of mild hemorrhagic shock (removal of 2 ml/100 g of blood over 10 min followed by resuscitation 1 h post-shock), where MSC administration decreased pulmonary edema, in part, by increasing adherens junction and tight junction protein expression, including VE-cadherin, claudin-1, and occludin-1.Citation106 A network analysis of experimental sepsis-induced MSC-mediated effects on common transcriptional responses in major target organs identified coordinated expression of transcriptional programs involved in preserving endothelial/vascular integrity, including upregulation of genes associated with gap junction tightening, calcium signaling, focal adhesion, and the Ang-1/Tie-2 pathway.Citation107
Ang-1 and keratinocyte growth factor (KGF) are thought to play important roles in the induction of MSC-mediated therapeutic effects.Citation2,Citation102,Citation108 In vitro, MSC-mediated alveolar cell permeability was attributed to Ang-1 production.Citation108 Moreover, in a murine model of LPS-induced lung injury, Ang-1 transfected MSCs were more effective at restoring lung/vascular integrity than non-transfected MSCs.Citation2 KGF production by MSCs was implicated in the restoration of alveolar fluid clearance in LPS-treated ex vivo-perfused human lungs.Citation102
Future Directions and Limitations
Emerging pre-clinical therapeutic strategies that target microvascular endothelial barrier activation/dysfunction represent promising approaches to prevent and/or limit end-organ dysfunction/injury in infectious diseases (summarized in ). In addition to the strategies discussed in this review, αvβ3 integrin regulators such as Fibulen5, NRP1, and Syndecan1 warrant pre-clinical evaluation for possible applications to limit infection-related microvascular leak.Citation109-Citation111
Table 2. Experimental results of selected pharmacological agents that have been investigated for their ability to enhance endothelial barrier integrity and reduce vascular leak. Agents are categorized by infectious disease/infectious agent/model of vascular leak
Given that a variety of viral infections may mediate endothelial dysregulation, therapeutic agents that target the host endothelium could potentially have relatively broad utility in the management of serious viral infections. It will also be important to assess new barrier-enhancing agents in combination with established therapeutic modalities (e.g., in combination with oseltamivir for the specific treatment of influenza). This complementary and robust treatment approach should allow for optimal therapeutic efficacy by targeting the infectious agent and the deleterious host responses in concert. For example, endothelial-stabilizing agents may be more effective when antimicrobial replication is diminished.
Given the failure of multiple therapeutic strategies showing promise in pre-clinical testing to yield positive results in clinical trials for the treatment of sepsis,Citation35,Citation36,Citation112 one must remain cautious when considering the therapeutic potential of endothelial stabilizing agents. It is also important to recognize that murine models, such as experimental sepsis, may fail to replicate important pathophysiological features of human disease.Citation113,Citation114 Well-designed, controlled clinical trials will be necessary to determine whether endothelial stabilization strategies will be effective in reducing infection-related morbidity and mortality associated with endothelial activation/dysfunction and associated microvascular leak.
Abbreviations: | ||
VEGFR2 | = | vascular endothelial growth factor receptor 2 |
S1P | = | sphingosine-1-phosphate |
ARF6 | = | ADP ribosylation factor 6 |
ARNO | = | ARF nucleotide binding site opener |
CAMs | = | cellular adhesion molecules |
Ang-1/2 | = | angiopoietin-1/2 |
ANP | = | atrial natriuretic peptide |
NPR | = | natriuretic peptide receptor |
PAK1 | = | Rac-dependent p21-activated kinase |
IFN-α | = | interferon-α |
Acknowledgments
Grant support provided to support this work by a Canada Research Chair in Infectious Diseases and Inflammation from the Canadian Institutes of Health Research (WCL).
Submitted
04/01/13
Revised
07/11/13
Accepted
07/12/13
Disclosure of Potential Conflicts of Interest
No potential conflicts of interest were disclosed.
References
- Goldenberg NM, Steinberg BE, Slutsky AS, Lee WL. Broken barriers: a new take on sepsis pathogenesis. Sci Transl Med 2011; 3:88ps25; http://dx.doi.org/10.1126/scitranslmed.3002011; PMID: 21697528
- Mei SH, McCarter SD, Deng Y, Parker CH, Liles WC, Stewart DJ. Prevention of LPS-induced acute lung injury in mice by mesenchymal stem cells overexpressing angiopoietin 1. PLoS Med 2007; 4:e269; http://dx.doi.org/10.1371/journal.pmed.0040269; PMID: 17803352
- Dalrymple NA, Mackow ER. Endothelial cells elicit immune-enhancing responses to dengue virus infection. J Virol 2012; 86:6408 - 15; http://dx.doi.org/10.1128/JVI.00213-12; PMID: 22496214
- Peters CJ, Zaki SR. Role of the endothelium in viral hemorrhagic fevers. Crit Care Med 2002; 30:Suppl S268 - 73; http://dx.doi.org/10.1097/00003246-200205001-00016; PMID: 12004247
- Shrivastava-Ranjan P, Rollin PE, Spiropoulou CF. Andes virus disrupts the endothelial cell barrier by induction of vascular endothelial growth factor and downregulation of VE-cadherin. J Virol 2010; 84:11227 - 34; http://dx.doi.org/10.1128/JVI.01405-10; PMID: 20810734
- Yuan SY, Rigor RR. Regulation of endothelial barrier function. San Rafael, CA: Morgan and Claypool Life Sciences, 2010.
- Wójciak-Stothard B, Potempa S, Eichholtz T, Ridley AJ. Rho and Rac but not Cdc42 regulate endothelial cell permeability. J Cell Sci 2001; 114:1343 - 55; PMID: 11257000
- Vestweber D, Broermann A, Schulte D. Control of endothelial barrier function by regulating vascular endothelial-cadherin. Curr Opin Hematol 2010; 17:230 - 6; http://dx.doi.org/10.1097/MOH.0b013e328338664b; PMID: 20393283
- Vandenbroucke E, Mehta D, Minshall R, Malik AB. Regulation of endothelial junctional permeability. Ann N Y Acad Sci 2008; 1123:134 - 45; http://dx.doi.org/10.1196/annals.1420.016; PMID: 18375586
- Olsson AK, Dimberg A, Kreuger J, Claesson-Welsh L. VEGF receptor signalling - in control of vascular function. Nat Rev Mol Cell Biol 2006; 7:359 - 71; http://dx.doi.org/10.1038/nrm1911; PMID: 16633338
- Gavard J, Gutkind JS. VEGF controls endothelial-cell permeability by promoting the beta-arrestin-dependent endocytosis of VE-cadherin. Nat Cell Biol 2006; 8:1223 - 34; http://dx.doi.org/10.1038/ncb1486; PMID: 17060906
- Gavard J, Patel V, Gutkind JS. Angiopoietin-1 prevents VEGF-induced endothelial permeability by sequestering Src through mDia. Dev Cell 2008; 14:25 - 36; http://dx.doi.org/10.1016/j.devcel.2007.10.019; PMID: 18194650
- Yang KY, Liu KT, Chen YC, Chen CS, Lee YC, Perng RP, et al. Plasma soluble vascular endothelial growth factor receptor-1 levels predict outcomes of pneumonia-related septic shock patients: a prospective observational study. Crit Care 2011; 15:R11; http://dx.doi.org/10.1186/cc9412; PMID: 21219633
- Mankhambo LA, Banda DL, Jeffers G, White SA, Balmer P, Nkhoma S, et al, IPD Study Group. The role of angiogenic factors in predicting clinical outcome in severe bacterial infection in Malawian children. Crit Care 2010; 14:R91; http://dx.doi.org/10.1186/cc9025; PMID: 20492647
- Pickkers P, Sprong T, Eijk Lv, Hoeven Hv, Smits P, Deuren Mv. Vascular endothelial growth factor is increased during the first 48 hours of human septic shock and correlates with vascular permeability. Shock 2005; 24:508 - 12; http://dx.doi.org/10.1097/01.shk.0000190827.36406.6e; PMID: 16317379
- van der Flier M, van Leeuwen HJ, van Kessel KP, Kimpen JL, Hoepelman AI, Geelen SP. Plasma vascular endothelial growth factor in severe sepsis. Shock 2005; 23:35 - 8; http://dx.doi.org/10.1097/01.shk.0000150728.91155.41; PMID: 15614129
- Shapiro NI, Aird WC. Sepsis and the broken endothelium. Crit Care 2011; 15:135; http://dx.doi.org/10.1186/cc10044; PMID: 21457513
- Pilot Study of Bevacizumab (Avastin) in Patients With Septic Shock. Available at: http://clinicaltrials.gov/ct2/show/NCT01063010 Accessed March 10, 2013.
- Gavrilovskaya IN, Gorbunova EE, Mackow NA, Mackow ER. Hantaviruses direct endothelial cell permeability by sensitizing cells to the vascular permeability factor VEGF, while angiopoietin 1 and sphingosine 1-phosphate inhibit hantavirus-directed permeability. J Virol 2008; 82:5797 - 806; http://dx.doi.org/10.1128/JVI.02397-07; PMID: 18367532
- Gorbunova EE, Gavrilovskaya IN, Pepini T, Mackow ER. VEGFR2 and Src kinase inhibitors suppress Andes virus-induced endothelial cell permeability. J Virol 2011; 85:2296 - 303; http://dx.doi.org/10.1128/JVI.02319-10; PMID: 21177802
- Yatomi Y, Ohmori T, Rile G, Kazama F, Okamoto H, Sano T, et al. Sphingosine 1-phosphate as a major bioactive lysophospholipid that is released from platelets and interacts with endothelial cells. Blood 2000; 96:3431 - 8; PMID: 11071638
- Schaphorst KL, Chiang E, Jacobs KN, Zaiman A, Natarajan V, Wigley F, et al. Role of sphingosine-1 phosphate in the enhancement of endothelial barrier integrity by platelet-released products. Am J Physiol Lung Cell Mol Physiol 2003; 285:L258 - 67; PMID: 12626332
- Pyne S, Pyne NJ. Sphingosine 1-phosphate signalling in mammalian cells. Biochem J 2000; 349:385 - 402; http://dx.doi.org/10.1042/0264-6021:3490385; PMID: 10880336
- Lee MJ, Thangada S, Liu CH, Thompson BD, Hla T. Lysophosphatidic acid stimulates the G-protein-coupled receptor EDG-1 as a low affinity agonist. J Biol Chem 1998; 273:22105 - 12; http://dx.doi.org/10.1074/jbc.273.34.22105; PMID: 9705355
- Lee MJ, Thangada S, Claffey KP, Ancellin N, Liu CH, Kluk M, et al. Vascular endothelial cell adherens junction assembly and morphogenesis induced by sphingosine-1-phosphate. Cell 1999; 99:301 - 12; http://dx.doi.org/10.1016/S0092-8674(00)81661-X; PMID: 10555146
- Camerer E, Regard JB, Cornelissen I, Srinivasan Y, Duong DN, Palmer D, et al. Sphingosine-1-phosphate in the plasma compartment regulates basal and inflammation-induced vascular leak in mice. J Clin Invest 2009; 119:1871 - 9; PMID: 19603543
- Garcia JGN, Liu F, Verin AD, Birukova A, Dechert MA, Gerthoffer WT, et al. Sphingosine 1-phosphate promotes endothelial cell barrier integrity by Edg-dependent cytoskeletal rearrangement. J Clin Invest 2001; 108:689 - 701; PMID: 11544274
- Su G, Atakilit A, Li JT, Wu N, Bhattacharya M, Zhu J, et al. Absence of integrin αvβ3 enhances vascular leak in mice by inhibiting endothelial cortical actin formation. Am J Respir Crit Care Med 2012; 185:58 - 66; http://dx.doi.org/10.1164/rccm.201108-1381OC; PMID: 21980034
- Finney CA, Hawkes CA, Kain DC, Dhabangi A, Musoke C, Cserti-Gazdewich C, et al. S1P is associated with protection in human and experimental cerebral malaria. Mol Med 2011; 17:717 - 25; http://dx.doi.org/10.2119/molmed.2010.00214; PMID: 21556483
- Feistritzer C, Riewald M. Endothelial barrier protection by activated protein C through PAR1-dependent sphingosine 1-phosphate receptor-1 crossactivation. Blood 2005; 105:3178 - 84; http://dx.doi.org/10.1182/blood-2004-10-3985; PMID: 15626732
- Looney MR, Esmon CT, Matthay MA. Role of coagulation pathways and treatment with activated protein C in hyperoxic lung injury. Thorax 2009; 64:114 - 20; http://dx.doi.org/10.1136/thx.2008.099135; PMID: 19008297
- Abraham E, Laterre PF, Garg R, Levy H, Talwar D, Trzaskoma BL, et al, Administration of Drotrecogin Alfa (Activated) in Early Stage Severe Sepsis (ADDRESS) Study Group. Drotrecogin alfa (activated) for adults with severe sepsis and a low risk of death. N Engl J Med 2005; 353:1332 - 41; http://dx.doi.org/10.1056/NEJMoa050935; PMID: 16192478
- Bernard GR, Vincent JL, Laterre PF, LaRosa SP, Dhainaut JF, Lopez-Rodriguez A, et al, Recombinant human protein C Worldwide Evaluation in Severe Sepsis (PROWESS) study group. Efficacy and safety of recombinant human activated protein C for severe sepsis. N Engl J Med 2001; 344:699 - 709; http://dx.doi.org/10.1056/NEJM200103083441001; PMID: 11236773
- Nadel S, Goldstein B, Williams MD, Dalton H, Peters M, Macias WL, et al, REsearching severe Sepsis and Organ dysfunction in children: a gLobal perspective (RESOLVE) study group. Drotrecogin alfa (activated) in children with severe sepsis: a multicentre phase III randomised controlled trial. Lancet 2007; 369:836 - 43; http://dx.doi.org/10.1016/S0140-6736(07)60411-5; PMID: 17350452
- Martí-Carvajal AJ, Solà I, Gluud C, Lathyris D, Cardona AF. Human recombinant protein C for severe sepsis and septic shock in adult and paediatric patients. Cochrane Database Syst Rev 2012; 12:CD004388; PMID: 23235609
- Ranieri VM, Thompson BT, Barie PS, Dhainaut J-F, Douglas IS, Finfer S, et al, PROWESS-SHOCK Study Group. Drotrecogin alfa (activated) in adults with septic shock. N Engl J Med 2012; 366:2055 - 64; http://dx.doi.org/10.1056/NEJMoa1202290; PMID: 22616830
- Kerschen EJ, Fernandez JA, Cooley BC, Yang XV, Sood R, Mosnier LO, et al. Endotoxemia and sepsis mortality reduction by non-anticoagulant activated protein C. J Exp Med 2007; 204:2439 - 48; http://dx.doi.org/10.1084/jem.20070404; PMID: 17893198
- McVerry BJ, Peng X, Hassoun PM, Sammani S, Simon BA, Garcia JGN. Sphingosine 1-phosphate reduces vascular leak in murine and canine models of acute lung injury. Am J Respir Crit Care Med 2004; 170:987 - 93; http://dx.doi.org/10.1164/rccm.200405-684OC; PMID: 15282202
- Sanna MG, Wang SK, Gonzalez-Cabrera PJ, Don A, Marsolais D, Matheu MP, et al. Enhancement of capillary leakage and restoration of lymphocyte egress by a chiral S1P1 antagonist in vivo. Nat Chem Biol 2006; 2:434 - 41; http://dx.doi.org/10.1038/nchembio804; PMID: 16829954
- Mandala S, Hajdu R, Bergstrom J, Quackenbush E, Xie J, Milligan J, et al. Alteration of lymphocyte trafficking by sphingosine-1-phosphate receptor agonists. Science 2002; 296:346 - 9; http://dx.doi.org/10.1126/science.1070238; PMID: 11923495
- Brinkmann V, Davis MD, Heise CE, Albert R, Cottens S, Hof R, et al. The immune modulator FTY720 targets sphingosine 1-phosphate receptors. J Biol Chem 2002; 277:21453 - 7; http://dx.doi.org/10.1074/jbc.C200176200; PMID: 11967257
- Brinkmann V, Billich A, Baumruker T, Heining P, Schmouder R, Francis G, et al. Fingolimod (FTY720): discovery and development of an oral drug to treat multiple sclerosis. Nat Rev Drug Discov 2010; 9:883 - 97; http://dx.doi.org/10.1038/nrd3248; PMID: 21031003
- Pyne S, Pyne NJ. Translational aspects of sphingosine 1-phosphate biology. Trends Mol Med 2011; 17:463 - 72; http://dx.doi.org/10.1016/j.molmed.2011.03.002; PMID: 21514226
- Sanchez T, Estrada-Hernandez T, Paik JH, Wu MT, Venkataraman K, Brinkmann V, et al. Phosphorylation and action of the immunomodulator FTY720 inhibits vascular endothelial cell growth factor-induced vascular permeability. J Biol Chem 2003; 278:47281 - 90; http://dx.doi.org/10.1074/jbc.M306896200; PMID: 12954648
- Walsh KB, Teijaro JR, Rosen H, Oldstone MB. Quelling the storm: utilization of sphingosine-1-phosphate receptor signaling to ameliorate influenza virus-induced cytokine storm. Immunol Res 2011; 51:15 - 25; http://dx.doi.org/10.1007/s12026-011-8240-z; PMID: 21901448
- Teijaro JR, Walsh KB, Cahalan S, Fremgen DM, Roberts E, Scott F, et al. Endothelial cells are central orchestrators of cytokine amplification during influenza virus infection. Cell 2011; 146:980 - 91; http://dx.doi.org/10.1016/j.cell.2011.08.015; PMID: 21925319
- Puneet P, Yap CT, Wong L, Lam Y, Koh DR, Moochhala S, et al. SphK1 regulates proinflammatory responses associated with endotoxin and polymicrobial sepsis. Science 2010; 328:1290 - 4; http://dx.doi.org/10.1126/science.1188635; PMID: 20522778
- Gonzalez-Cabrera PJ, Hla T, Rosen H. Mapping pathways downstream of sphingosine 1-phosphate subtype 1 by differential chemical perturbation and proteomics. J Biol Chem 2007; 282:7254 - 64; http://dx.doi.org/10.1074/jbc.M610581200; PMID: 17218309
- Bach TL, Barsigian C, Yaen CH, Martinez J. Endothelial cell VE-cadherin functions as a receptor for the beta15-42 sequence of fibrin. J Biol Chem 1998; 273:30719 - 28; http://dx.doi.org/10.1074/jbc.273.46.30719; PMID: 9804847
- Petzelbauer P, Zacharowski PA, Miyazaki Y, Friedl P, Wickenhauser G, Castellino FJ, et al. The fibrin-derived peptide Bbeta15-42 protects the myocardium against ischemia-reperfusion injury. Nat Med 2005; 11:298 - 304; http://dx.doi.org/10.1038/nm1198; PMID: 15723073
- Gröger M, Pasteiner W, Ignatyev G, Matt U, Knapp S, Atrasheuskaya A, et al. Peptide Bbeta(15-42) preserves endothelial barrier function in shock. PLoS One 2009; 4:e5391; http://dx.doi.org/10.1371/journal.pone.0005391; PMID: 19401765
- Jennewein C, Mehring M, Tran N, Paulus P, Ockelmann PA, Habeck K, et al. The fibrinopeptide bβ15-42 reduces inflammation in mice subjected to polymicrobial sepsis. Shock 2012; 38:275 - 80; http://dx.doi.org/10.1097/SHK.0b013e318264b95d; PMID: 22777114
- Jones CA, London NR, Chen H, Park KW, Sauvaget D, Stockton RA, et al. Robo4 stabilizes the vascular network by inhibiting pathologic angiogenesis and endothelial hyperpermeability. Nat Med 2008; 14:448 - 53; http://dx.doi.org/10.1038/nm1742; PMID: 18345009
- Jones CA, Nishiya N, London NR, Zhu W, Sorensen LK, Chan AC, et al. Slit2-Robo4 signalling promotes vascular stability by blocking Arf6 activity. Nat Cell Biol 2009; 11:1325 - 31; http://dx.doi.org/10.1038/ncb1976; PMID: 19855388
- London NR, Zhu W, Bozza FA, Smith MCP, Greif DM, Sorensen LK, et al. Targeting Robo4-dependent Slit signaling to survive the cytokine storm in sepsis and influenza. Sci Transl Med 2010; 2:23ra19; http://dx.doi.org/10.1126/scitranslmed.3000678; PMID: 20375003
- Anand AR, Zhao H, Nagaraja T, Robinson LA, Ganju RK. N-terminal Slit2 inhibits HIV-1 replication by regulating the actin cytoskeleton. Retrovirology 2013; 10:2; http://dx.doi.org/10.1186/1742-4690-10-2; PMID: 23294842
- Zhu W, London NR, Gibson CC, Davis CT, Tong Z, Sorensen LK, et al. Interleukin receptor activates a MYD88-ARNO-ARF6 cascade to disrupt vascular stability. Nature 2012; 492:252 - 5; http://dx.doi.org/10.1038/nature11603; PMID: 23143332
- Yancopoulos GD, Davis S, Gale NW, Rudge JS, Wiegand SJ, Holash J. Vascular-specific growth factors and blood vessel formation. Nature 2000; 407:242 - 8; http://dx.doi.org/10.1038/35025215; PMID: 11001067
- Davis S, Aldrich TH, Jones PF, Acheson A, Compton DL, Jain V, et al. Isolation of angiopoietin-1, a ligand for the TIE2 receptor, by secretion-trap expression cloning. Cell 1996; 87:1161 - 9; http://dx.doi.org/10.1016/S0092-8674(00)81812-7; PMID: 8980223
- Thurston G, Suri C, Smith K, McClain J, Sato TN, Yancopoulos GD, et al. Leakage-resistant blood vessels in mice transgenically overexpressing angiopoietin-1. Science 1999; 286:2511 - 4; http://dx.doi.org/10.1126/science.286.5449.2511; PMID: 10617467
- Thurston G, Rudge JS, Ioffe E, Zhou H, Ross L, Croll SD, et al. Angiopoietin-1 protects the adult vasculature against plasma leakage. Nat Med 2000; 6:460 - 3; http://dx.doi.org/10.1038/74725; PMID: 10742156
- Gamble JR, Drew J, Trezise L, Underwood A, Parsons M, Kasminkas L, et al. Angiopoietin-1 is an antipermeability and anti-inflammatory agent in vitro and targets cell junctions. Circ Res 2000; 87:603 - 7; http://dx.doi.org/10.1161/01.RES.87.7.603; PMID: 11009566
- Jain RK, Munn LL. Leaky vessels? Call Ang1!. Nat Med 2000; 6:131 - 2; http://dx.doi.org/10.1038/72212; PMID: 10655092
- Kim DH, Jung YJ, Lee AS, Lee S, Kang KP, Lee TH, et al. COMP-angiopoietin-1 decreases lipopolysaccharide-induced acute kidney injury. Kidney Int 2009; 76:1180 - 91; http://dx.doi.org/10.1038/ki.2009.387; PMID: 19812542
- Witzenbichler B, Westermann D, Knueppel S, Schultheiss HP, Tschope C. Protective role of angiopoietin-1 in endotoxic shock. Circulation 2005; 111:97 - 105; http://dx.doi.org/10.1161/01.CIR.0000151287.08202.8E; PMID: 15611372
- McCarter SD, Mei SH, Lai PF, Zhang QW, Parker CH, Suen RS, et al. Cell-based angiopoietin-1 gene therapy for acute lung injury. Am J Respir Crit Care Med 2007; 175:1014 - 26; http://dx.doi.org/10.1164/rccm.200609-1370OC; PMID: 17322110
- Huang YQ, Sauthoff H, Herscovici P, Pipiya T, Cheng J, Heitner S, et al. Angiopoietin-1 increases survival and reduces the development of lung edema induced by endotoxin administration in a murine model of acute lung injury. Crit Care Med 2008; 36:262 - 7; http://dx.doi.org/10.1097/01.CCM.0000297955.02633.A4; PMID: 18007265
- Kumpers P, Gueler F, David S, Slyke PV, Dumont DJ, Park JK, et al. The synthetic tie2 agonist peptide vasculotide protects against vascular leakage and reduces mortality in murine abdominal sepsis. Crit Care 2011; 15:R261; http://dx.doi.org/10.1186/cc10523; PMID: 22040774
- David S, Ghosh CC, Kümpers P, Shushakova N, Van Slyke P, Khankin EV, et al. Effects of a synthetic PEG-ylated Tie-2 agonist peptide on endotoxemic lung injury and mortality. Am J Physiol Lung Cell Mol Physiol 2011; 300:L851 - 62; http://dx.doi.org/10.1152/ajplung.00459.2010; PMID: 21421750
- Alfieri A, Watson JJ, Kammerer RA, Tasab M, Progias P, Reeves K, et al. Angiopoietin-1 variant reduces LPS-induced microvascular dysfunction in a murine model of sepsis. Crit Care 2012; 16:R182; http://dx.doi.org/10.1186/cc11666; PMID: 23036162
- Parikh SM, Mammoto T, Schultz A, Yuan HT, Christiani D, Karumanchi SA, et al. Excess circulating angiopoietin-2 may contribute to pulmonary vascular leak in sepsis in humans. PLoS Med 2006; 3:e46; http://dx.doi.org/10.1371/journal.pmed.0030046; PMID: 16417407
- Fiedler U, Reiss Y, Scharpfenecker M, Grunow V, Koidl S, Thurston G, et al. Angiopoietin-2 sensitizes endothelial cells to TNF-alpha and has a crucial role in the induction of inflammation. Nat Med 2006; 12:235 - 9; http://dx.doi.org/10.1038/nm1351; PMID: 16462802
- Kümpers P, Lukasz A, David S, Horn R, Hafer C, Faulhaber-Walter R, et al. Excess circulating angiopoietin-2 is a strong predictor of mortality in critically ill medical patients. Crit Care 2008; 12:R147; http://dx.doi.org/10.1186/cc7130; PMID: 19025590
- Kümpers P, Hafer C, David S, Hecker H, Lukasz A, Fliser D, et al. Angiopoietin-2 in patients requiring renal replacement therapy in the ICU: relation to acute kidney injury, multiple organ dysfunction syndrome and outcome. Intensive Care Med 2010; 36:462 - 70; http://dx.doi.org/10.1007/s00134-009-1726-7; PMID: 19956923
- David S, Mukherjee A, Ghosh CC, Yano M, Khankin EV, Wenger JB, et al. Angiopoietin-2 may contribute to multiple organ dysfunction and death in sepsis. Crit Care Med 2012; 40:3034 - 41; http://dx.doi.org/10.1097/CCM.0b013e31825fdc31; PMID: 22890252
- Tzepi IM, Giamarellos-Bourboulis EJ, Carrer DP, Tsaganos T, Claus RA, Vaki I, et al. Angiopoietin-2 enhances survival in experimental sepsis induced by multidrug-resistant Pseudomonas aeruginosa. J Pharmacol Exp Ther 2012; 343:278 - 87; http://dx.doi.org/10.1124/jpet.112.195180; PMID: 22859861
- Daly C, Pasnikowski E, Burova E, Wong V, Aldrich TH, Griffiths J, et al. Angiopoietin-2 functions as an autocrine protective factor in stressed endothelial cells. Proc Natl Acad Sci U S A 2006; 103:15491 - 6; http://dx.doi.org/10.1073/pnas.0607538103; PMID: 17030814
- Greenwood J, Mason JC. Statins and the vascular endothelial inflammatory response. Trends Immunol 2007; 28:88 - 98; http://dx.doi.org/10.1016/j.it.2006.12.003; PMID: 17197237
- Bickel C, Rupprecht HJ, Blankenberg S, Espiniola-Klein C, Schlitt A, Rippin G, et al, AtheroGene Investigators. Relation of markers of inflammation (C-reactive protein, fibrinogen, von Willebrand factor, and leukocyte count) and statin therapy to long-term mortality in patients with angiographically proven coronary artery disease. Am J Cardiol 2002; 89:901 - 8; http://dx.doi.org/10.1016/S0002-9149(02)02236-1; PMID: 11950425
- Ravi LI, Li L, Wong PS, Sutejo R, Tan BH, Sugrue RJ. Lovastatin treatment mitigates the pro-inflammatory cytokine response in respiratory syncytial virus infected macrophage cells. Antiviral Res 2013; 98:332 - 43; http://dx.doi.org/10.1016/j.antiviral.2013.03.015; PMID: 23523944
- Darwish I, Mubareka S, Liles WC. Immunomodulatory therapy for severe influenza. Expert Rev Anti Infect Ther 2011; 9:807 - 22; http://dx.doi.org/10.1586/eri.11.56; PMID: 21810053
- Omi H, Okayama N, Shimizu M, Fukutomi T, Imaeda K, Okouchi M, et al. Statins inhibit high glucose-mediated neutrophil-endothelial cell adhesion through decreasing surface expression of endothelial adhesion molecules by stimulating production of endothelial nitric oxide. Microvasc Res 2003; 65:118 - 24; http://dx.doi.org/10.1016/S0026-2862(02)00033-X; PMID: 12686169
- Takeuchi S, Kawashima S, Rikitake Y, Ueyama T, Inoue N, Hirata K, et al. Cerivastatin suppresses lipopolysaccharide-induced ICAM-1 expression through inhibition of Rho GTPase in BAEC. Biochem Biophys Res Commun 2000; 269:97 - 102; http://dx.doi.org/10.1006/bbrc.2000.2238; PMID: 10694484
- Dichtl W, Dulak J, Frick M, Alber HF, Schwarzacher SP, Ares MP, et al. HMG-CoA reductase inhibitors regulate inflammatory transcription factors in human endothelial and vascular smooth muscle cells. Arterioscler Thromb Vasc Biol 2003; 23:58 - 63; http://dx.doi.org/10.1161/01.ATV.0000043456.48735.20; PMID: 12524225
- Boyd AR, Hinojosa CA, Rodriguez PJ, Orihuela CJ. Impact of oral simvastatin therapy on acute lung injury in mice during pneumococcal pneumonia. BMC Microbiol 2012; 12:73; http://dx.doi.org/10.1186/1471-2180-12-73; PMID: 22587610
- Sabrane K, Kruse MN, Fabritz L, Zetsche B, Mitko D, Skryabin BV, et al. Vascular endothelium is critically involved in the hypotensive and hypovolemic actions of atrial natriuretic peptide. J Clin Invest 2005; 115:1666 - 74; http://dx.doi.org/10.1172/JCI23360; PMID: 15931395
- Birukova AA, Xing J, Fu P, Yakubov B, Dubrovskyi O, Fortune JA, et al. Atrial natriuretic peptide attenuates LPS-induced lung vascular leak: role of PAK1. Am J Physiol Lung Cell Mol Physiol 2010; 299:L652 - 63; http://dx.doi.org/10.1152/ajplung.00202.2009; PMID: 20729389
- Xing J, Birukova AA. ANP attenuates inflammatory signaling and Rho pathway of lung endothelial permeability induced by LPS and TNFalpha. Microvasc Res 2010; 79:56 - 62; http://dx.doi.org/10.1016/j.mvr.2009.11.006; PMID: 19931545
- Bishop AL, Hall A. Rho GTPases and their effector proteins. Biochem J 2000; 348:241 - 55; http://dx.doi.org/10.1042/0264-6021:3480241; PMID: 10816416
- Hama N, Itoh H, Shirakami G, Suga S, Komatsu Y, Yoshimasa T, et al. Detection of C-type natriuretic peptide in human circulation and marked increase of plasma CNP level in septic shock patients. Biochem Biophys Res Commun 1994; 198:1177 - 82; http://dx.doi.org/10.1006/bbrc.1994.1166; PMID: 8117275
- Baron DA, Lofton CE, Newman WH, Currie MG. Atriopeptin inhibition of thrombin-mediated changes in the morphology and permeability of endothelial monolayers. Proc Natl Acad Sci U S A 1989; 86:3394 - 8; http://dx.doi.org/10.1073/pnas.86.9.3394; PMID: 2524068
- Pedram A, Razandi M, Levin ER. Deciphering vascular endothelial cell growth factor/vascular permeability factor signaling to vascular permeability. Inhibition by atrial natriuretic peptide. J Biol Chem 2002; 277:44385 - 98; http://dx.doi.org/10.1074/jbc.M202391200; PMID: 12213803
- Klinger JR, Tsai SW, Green S, Grinnell KL, Machan JT, Harrington EO. Atrial natriuretic peptide attenuates agonist-induced pulmonary edema in mice with targeted disruption of the gene for natriuretic peptide receptor-A. J Appl Physiol 2013; 114:307 - 15; http://dx.doi.org/10.1152/japplphysiol.01249.2011; PMID: 23195629
- Xing J, Moldobaeva N, Birukova AA. Atrial natriuretic peptide protects against Staphylococcus aureus-induced lung injury and endothelial barrier dysfunction. J Appl Physiol 2011; 110:213 - 24; http://dx.doi.org/10.1152/japplphysiol.00284.2010; PMID: 21051573
- Mitaka C, Hirata Y, Nagura T, Tsunoda Y, Amaha K. Beneficial effect of atrial natriuretic peptide on pulmonary gas exchange in patients with acute lung injury. Chest 1998; 114:223 - 8; http://dx.doi.org/10.1378/chest.114.1.223; PMID: 9674473
- Bindels AJGH, van der Hoeven JG, Groeneveld PHP, Frölich M, Meinders AE. Atrial natriuretic peptide infusion and nitric oxide inhalation in patients with acute respiratory distress syndrome. Crit Care 2001; 5:151 - 7; http://dx.doi.org/10.1186/cc1015; PMID: 11353932
- Bianco P, Gehron Robey P. Marrow stromal stem cells. J Clin Invest 2000; 105:1663 - 8; http://dx.doi.org/10.1172/JCI10413; PMID: 10862779
- Rojas M, Xu J, Woods CR, Mora AL, Spears W, Roman J, et al. Bone marrow-derived mesenchymal stem cells in repair of the injured lung. Am J Respir Cell Mol Biol 2005; 33:145 - 52; http://dx.doi.org/10.1165/rcmb.2004-0330OC; PMID: 15891110
- Ortiz LA, Gambelli F, McBride C, Gaupp D, Baddoo M, Kaminski N, et al. Mesenchymal stem cell engraftment in lung is enhanced in response to bleomycin exposure and ameliorates its fibrotic effects. Proc Natl Acad Sci U S A 2003; 100:8407 - 11; http://dx.doi.org/10.1073/pnas.1432929100; PMID: 12815096
- Xu J, Woods CR, Mora AL, Joodi R, Brigham KL, Iyer S, et al. Prevention of endotoxin-induced systemic response by bone marrow-derived mesenchymal stem cells in mice. Am J Physiol Lung Cell Mol Physiol 2007; 293:L131 - 41; http://dx.doi.org/10.1152/ajplung.00431.2006; PMID: 17416739
- Gupta N, Su X, Popov B, Lee JW, Serikov V, Matthay MA. Intrapulmonary delivery of bone marrow-derived mesenchymal stem cells improves survival and attenuates endotoxin-induced acute lung injury in mice. J Immunol 2007; 179:1855 - 63; PMID: 17641052
- Lee JW, Fang X, Gupta N, Serikov V, Matthay MA. Allogeneic human mesenchymal stem cells for treatment of E. coli endotoxin-induced acute lung injury in the ex vivo perfused human lung. Proc Natl Acad Sci U S A 2009; 106:16357 - 62; http://dx.doi.org/10.1073/pnas.0907996106; PMID: 19721001
- Lee JW, Gupta N, Serikov V, Matthay MA. Potential application of mesenchymal stem cells in acute lung injury. Expert Opin Biol Ther 2009; 9:1259 - 70; http://dx.doi.org/10.1517/14712590903213651; PMID: 19691441
- Mei SHJ, Haitsma JJ, Dos Santos CC, Deng Y, Lai PFH, Slutsky AS, et al. Mesenchymal stem cells reduce inflammation while enhancing bacterial clearance and improving survival in sepsis. Am J Respir Crit Care Med 2010; 182:1047 - 57; http://dx.doi.org/10.1164/rccm.201001-0010OC; PMID: 20558630
- Németh K, Leelahavanichkul A, Yuen PST, Mayer B, Parmelee A, Doi K, et al. Bone marrow stromal cells attenuate sepsis via prostaglandin E(2)-dependent reprogramming of host macrophages to increase their interleukin-10 production. Nat Med 2009; 15:42 - 9; http://dx.doi.org/10.1038/nm.1905; PMID: 19098906
- Pati S, Gerber MH, Menge TD, Wataha KA, Zhao Y, Baumgartner JA, et al. Bone marrow derived mesenchymal stem cells inhibit inflammation and preserve vascular endothelial integrity in the lungs after hemorrhagic shock. PLoS One 2011; 6:e25171; http://dx.doi.org/10.1371/journal.pone.0025171; PMID: 21980392
- dos Santos CC, Murthy S, Hu P, Shan Y, Haitsma JJ, Mei SH, et al. Network analysis of transcriptional responses induced by mesenchymal stem cell treatment of experimental sepsis. Am J Pathol 2012; 181:1681 - 92; http://dx.doi.org/10.1016/j.ajpath.2012.08.009; PMID: 23083833
- Fang X, Neyrinck AP, Matthay MA, Lee JW. Allogeneic human mesenchymal stem cells restore epithelial protein permeability in cultured human alveolar type II cells by secretion of angiopoietin-1. J Biol Chem 2010; 285:26211 - 22; http://dx.doi.org/10.1074/jbc.M110.119917; PMID: 20554518
- Wang L, Zeng H, Wang P, Soker S, Mukhopadhyay D. Neuropilin-1-mediated vascular permeability factor/vascular endothelial growth factor-dependent endothelial cell migration. J Biol Chem 2003; 278:48848 - 60; http://dx.doi.org/10.1074/jbc.M310047200; PMID: 14514674
- Beauvais DM, Ell BJ, McWhorter AR, Rapraeger AC. Syndecan-1 regulates alphavbeta3 and alphavbeta5 integrin activation during angiogenesis and is blocked by synstatin, a novel peptide inhibitor. J Exp Med 2009; 206:691 - 705; http://dx.doi.org/10.1084/jem.20081278; PMID: 19255147
- Beauvais DM, Rapraeger AC. Syndecan-1 couples the insulin-like growth factor-1 receptor to inside-out integrin activation. J Cell Sci 2010; 123:3796 - 807; http://dx.doi.org/10.1242/jcs.067645; PMID: 20971705
- Wheeler DS. Another potential therapeutic agent for the management of critically ill patients with sepsis syndrome: same old story, same old result?. Crit Care Med 2012; 40:1012 - 3; http://dx.doi.org/10.1097/CCM.0b013e31823c8b42; PMID: 22343861
- Dyson A, Singer M. Animal models of sepsis: why does preclinical efficacy fail to translate to the clinical setting?. Crit Care Med 2009; 37:Suppl S30 - 7; http://dx.doi.org/10.1097/CCM.0b013e3181922bd3; PMID: 19104223
- Seok J, Warren HS, Cuenca AG, Mindrinos MN, Baker HV, Xu W, et al, Inflammation and Host Response to Injury, Large Scale Collaborative Research Program. Genomic responses in mouse models poorly mimic human inflammatory diseases. Proc Natl Acad Sci U S A 2013; 110:3507 - 12; http://dx.doi.org/10.1073/pnas.1222878110; PMID: 23401516