Figures & data
Figure 1 The interaction between the receptor-binding domain in the spike of an emerging virus and the corresponding receptor on the target cell.
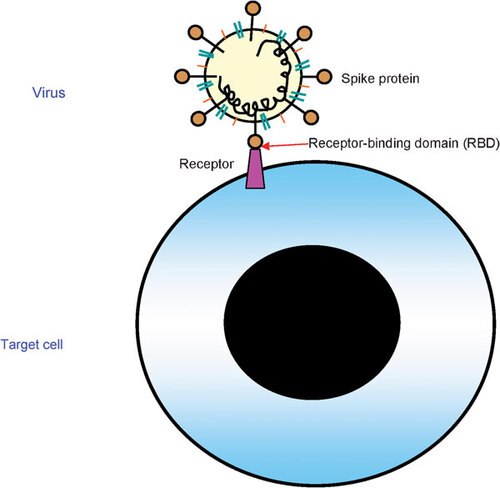
Figure 2 The RBDs in the envelope glycoproteins of SARS-CoV, influenza virus and Hendra virus. (A) RBD in the SARS-CoV spike protein.Citation14,Citation17,Citation79 The residue numbers of each region represent their positions in the S protein of SARS-CoV. (B) RBD in influenza virus HA. Taking H5N1 as an example, HA consists of a globular ‘head’ HA1 subunit containing the receptor-binding domain RBD and a membrane proximal HA2 subunit with α-helical stalk region (FP). The leading sequence (SP) is located at the N-terminus of HA1. There is a protease cleavage site between HA1 and HA2 subunits characterized by specific amino acid sequences, e.g., RERRRKKR. HA1 and HA2 subunits were connected by interchain disulfide bond from HA1-aa14 to HA2-aa137.Citation80 (C) RBD in the Hendra virus G glycoprotein. There are many glycosylate sites in the extracellular domain located in 72, 159, 306, 378, 417, 481 and 529, respectively. Residues from aa 439 to aa 468, which are located in RBD domain, are an important domain within G for receptor interaction. CP, cytoplasm domain; EC, extracellular domain; FP, fusion peptide; HA, hemagglutinin; HR, heptad repeat; RBM, receptor-binding motif; TM, transmembrane domain; SP, signal peptide.
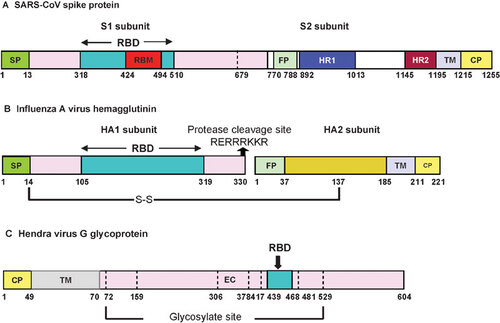
Figure 3 The SARS-CoV life cycle in host cells and its S protein structure. Life cycle of SARS-CoV. SARS-CoV begins its life cycle when its S protein binds to the cellular receptor ACE2. After receptor binding, the conformation change in the S protein facilitates viral envelope fusion with the cell membrane through the endosomal pathway. Then SARS-CoV releases RNA into the host cell. Genome RNA is translated into viral replicase polyproteins pp1a and 1ab, which are then cleaved into small products by viral proteinases. At the same time, polymerase, which produces a series of subgenomic mRNAs by discontinuous transcription, is finally translated into relevant viral proteins. Viral proteins and genome RNA are subsequently assembled into virions in the ER and Golgi, which are budding into the lumen of the ERGIC and then transported via vesicles and released out of the cell. ACE2, angiotensin-converting enzyme 2; ER, endoplasmic reticulum; ERGIC, ER–Golgi intermediate compartment.
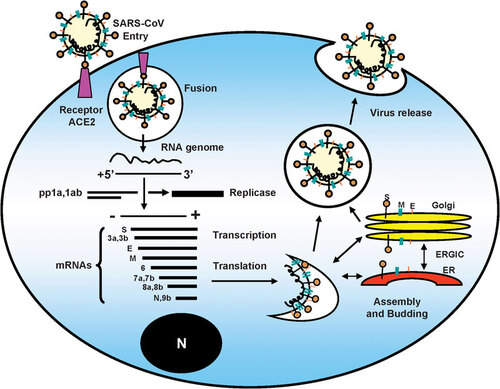
Figure 4 The structure and natural reservoirs of HeV. The principal natural reservoirs for HeV are fruit bats. Recent evidence of Hendra infection has indicated that flying foxes can also be natural reservoirs. The secondary vectors can be laboratory rodents, cats, dogs and rabbits. The dead-end hosts are horse and man. HeV has six major structural proteins. They are nucleocapsid protein (N), phosphorprotein (P), matrix protein (M), fusion protein (F), glycoprotein (G) and large protein (L). HeV, Hendra virus.
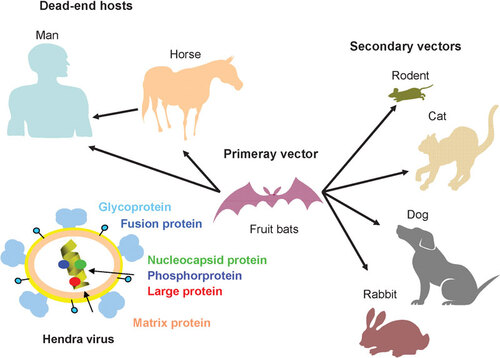