Figures & data
Figure 1. Schematic illustration of the two-step in vivo tumor-targeting strategy for nanoparticles via metabolic glycoengineering and click chemistry. Reproduced with permission from ACS 2014 (Lee et al., Citation2014).
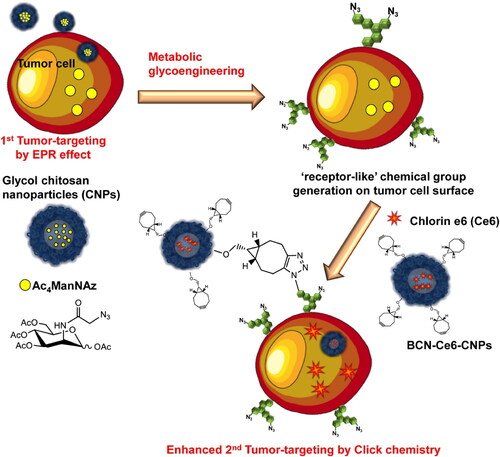
Figure 2. The tricarboxylic acid (TCA) cycle and the glutaminolysis pathway interact in cancer, and both are frequently dysregulated. The metabolism of glutamine is essential for the survival and growth of numerous types of cancer cells. Increased expression of the oncogene MYC results in an increase in glutamine absorption and glutaminolysis in cancer cells. Both the TCA cycle and glutaminolysis contribute to an increase in citrate and acetyl-CoA, which ultimately results in a rise in the fatty acid pool. Reactions that are upregulated in cancer cells are shown by thicker black arrows, and upregulated enzymes are highlighted in bold font. Red indicates current clinical or preclinical cancer medicines that target enzymes related to lipogenesis, the TCA cycle, or glutaminolysis. Cancer cell oncogenes that stimulate lipogenesis, the TCA cycle, or glutaminolysis are denoted in blue. Reproduce with permission from ACS 2018 (Counihan et al., Citation2018).
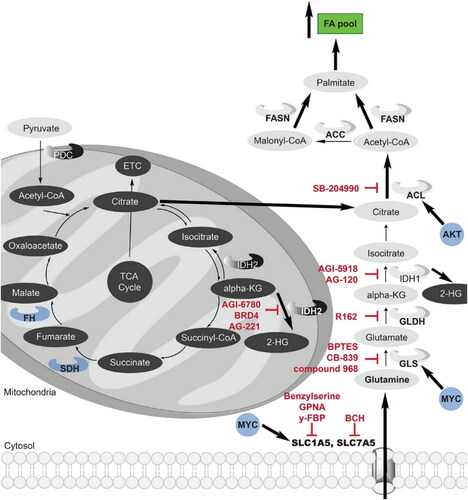
Figure 3. A historical look at the use of nanotechnology in the development of drug delivery systems, with a focus on a few key innovations. Reproduced with permission from ACS 2010 (Shi et al., Citation2010).
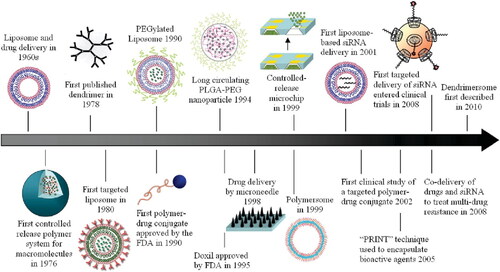
Figure 4. A synopsis of currently available nanomedicines for the treatment of cancer. Clinical studies are looking into a wide range of platforms as nanocarriers, such as drug-conjugated NPs, viral NPs, inorganic NPs, polymer-based NPs, and lipid-based NPs. Reproduced with permission from reference (Aghebati-Maleki et al., Citation2020).
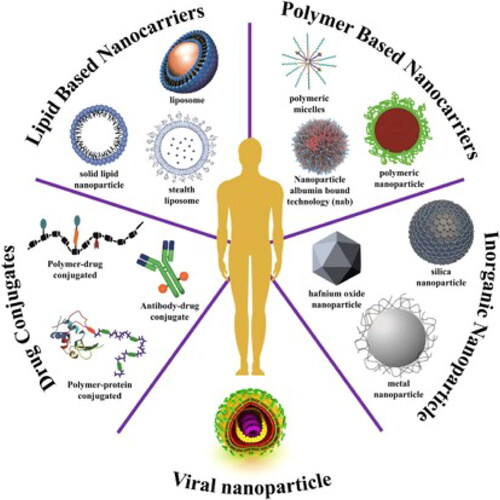
Figure 5. Schematic depiction of JX06-NPs inhibiting the expression of PDK1 in combination with metformin to target EC and modify glucose metabolism for a combinatorial anticancer action. In addition to lowering blood glucose levels, metformin could potentially block oxidative phosphorylation and mitochondrial complex I. JX06-NPs might then release JX06 after being entered into cancer cells, thereby downregulating the PDK1 expression and inhibiting glycolysis. Hence, the combination of JX06-NPs and metformin could expedite EC apoptosis and suppress tumor growth (Yang et al., Citation2022).
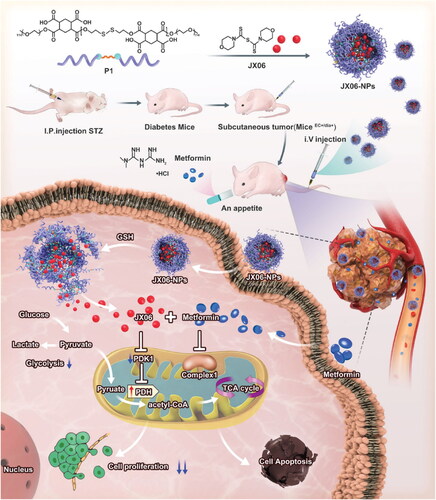
Figure 6. To counteract the effects of medication resistance and cancer spread, this diagram shows how CD-DOX-NO-DA NPs are synthesized and manufactured. A) A schematic representation of the CD-DOX-NO-DA NPs manufacturing process. B) A schematic representation of CD-DOX-NO-DA NPs delivering NO to mitochondria (Deng et al., Citation2020).
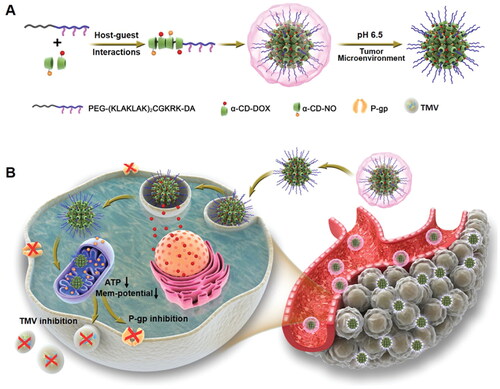
Figure 7. Proposed signaling pathways that are involved in the protection afforded by SeNPs (Amani et al., Citation2019).
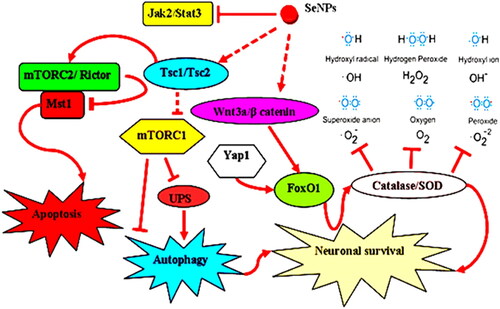
Figure 8. The model demonstrates how PSMA aptamer coupled PBM-NPs can suppress prostate cancer carcinogenesis by targeting the Hh pathway. Regulating the transcription of the ABC transporter gene, the Hh pathway produces chemoresistance. In addition, the Hh pathway involves the activation of SHH, which binds to the receptor PTCH1, followed by inhibition of SMO and, finally, stimulation of GLI-dependent transcription. The suppressor of fused (SUFU) is a transcription factor that binds to the GLIs (GLI-1, -2, and -3) and regulates the expression of their downstream proteins. Modeling the binding of thymoquinone encapsulated A10 aptamer-conjugated PBM-NPs to the PSMA receptor, expressed on the cell surface, revealed the method by which it may be achieved. The aptamer-conjugated nanoparticles blocked the ABC drug efflux protein after being taken up by the cell via endocytosis and transported inside (MDR1). PBM-NPs inhibit cell growth and modify other features of PCa cells by binding to the SHH protein (Singh et al., Citation2020).
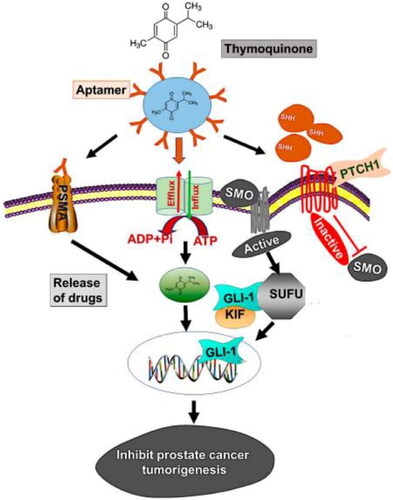
Figure 9. Schematic Illustration of Biodegradable CoSx QDs for PTT and Hyperthermal-Enhanced CDT of Tumors. Reproduced with permission from ACS 2022 (Zhu et al., Citation2022).
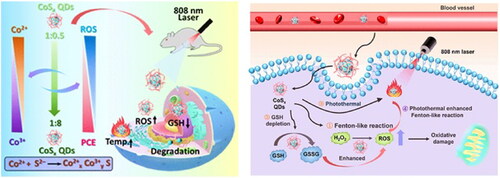
Table 1. Examples of various NPs and their cancer therapeutic potential in targeting cancer metabolism.
Availability of data and materials
Not applicable.