Figures & data
Figure 1. HDAC5 knockdown induces HIF-1α degradation. (A) Confirmation of HDAC knockdown in Hep3B. 42 h after siRNAs transfection, total RNA were collected and qRT-PCR was performed to determine the mRNA levels of HDACs. siR: siRNA.(B) HDAC5 knockdown attenuates hypoxia-triggered HIF-1α accumulation. Hep3B transfected with siRNA were exposed to 1% O2 for 6 h. Protein levels of HIF-1α, HDACs and α-tubulin (loading control) were determined by Western. (C) HDAC5 overexpression rescues TSA-induced HIF-1α degradation. Hep3B cells were transfected with 0.25, 0.5, 2 μg of Flag-HDAC5 and cultured in 1% O2 with TSA (300 nM) or DMSO (control) for 6 h. HIF-1α and Flag-HDAC5 were detected by Western blotting. (D) MG132 blocks HDAC5 knockdown-caused decrease of HIF-1α. Hep3B were transfected with HDAC5 or control siRNA, exposed to 1% O2 in the presence or absence of MG132 (5 μM) for 6 h. (E-H) HDAC5 knockdown blocks HIF-1α accumulation in HeLa (E-F) and MCF7 (G-H) cells. Procedures are the same as in (A) and (B). The mRNA levels of target genes were examined by qRT-PCR, and HIF-1α levels determined by Western blotting.
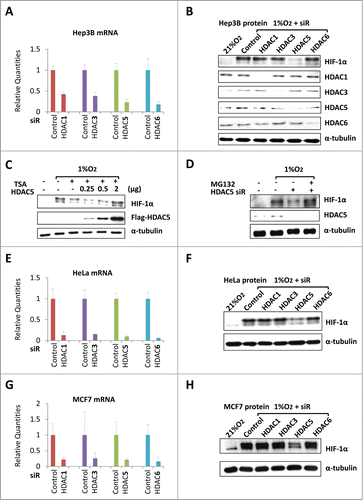
Figure 2. HDAC5 specific inhibitor LMK235 impairs hypoxic accumulation of HIF-1α by ubiquitination-independent pathway. (A) Dose dependent effects of LMK235 on HIF-1α. Hep3B cells were treated with 0, 25 or 50 nM of LMK235 and exposed to 1% O2 for 6 h prior to analysis. (B) Effects of LMK235 on hypoxia-induced accumulation of HIF-1α. Hep3B cells were cultured with DMSO as control (−) or 50 nM of LMK235 (+) in 1% O2 for 0 to 6 h, HIF-1α were examined by Western blotting. (C) MG132 blocks LMK235-triggered reduction of HIF-1α. Hep3B cells were cultured in 1% O2 for 6 h in the presence of 25 nM of LMK235, with or without MG132 (5 μM). (D) LMK235-induced degradation of HIF-1α is hydroxylation-independent. Hep3B cells were treated with DFX (100 μM) for 0 to 4 h in the presence or absence of LMK235 (25 nM). (E) LMK235-triggers ubiquitination-indepdendent degradation of HIF-1α. TS20 cells with temperature sensitive ubiquitin activating enzyme E1 were cultured at 39°C for 6 h with or without LMK235. The LMK235-triggered degradation was blocked by MG132 (5 μM). (F-G) Dose-dependent effect of HDACIs on HIF-1α in cardiomyocyte. H9c2 cells were cultured with 0, 500 or 1000 nM of TSA (F), or 0, 25 or 50 nM of LMK235 (G), and exposed to 1% O2 for 6 h prior to analysis for HIF-1α.
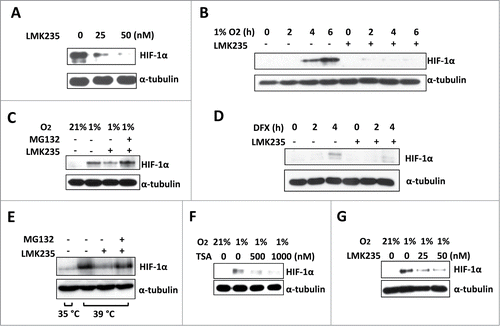
Figure 3. HDAC5 knockdown attenuates hypoxia-induced HIF-1α accumulation and impairs hypoxic activation of HIF-1 function. (A) Effect of HDAC5 knockdown on HIF-1α accumulation in response to hypoxia. Hep3B cells were transfected with HDAC5 or control siRNA. After 44 h, cells were exposed to 1% O2 for 0 to 4 h in the presence or absence of MG132 (5 μM). HIF-1α levels at each time point were determined. (B) Quantification of data in (A). The HIF-1α levels in were quantified by ImageJ software and normalized to α-tubulin. (C-D) HDAC5 knockdown represses the expression of HIF-1 target genes. 42 h after transfection with indicated siRNAs, MCF7 cells were exposed to 1% O2 for 6 h. The mRNA levels of CA-IX and GLUT1 were determined by qRT-PCR as indicators of HIF-1 function. (E) HDAC5 knockdown represses hypoxia-stimulated lactate fermentation. HeLa Cells transfected with control or HDAC5 siRNA were cultured for 48 h in 21% or 1% O2. Media were collected, lactate levels measured and normalized to cell numbers. (F-G) HDAC5 knockdown by shRNA represses nuclear accumulation of HIF-1α. HeLa cells transduced with control or HDAC5 shRNA were cultured with hydroxylase inhibitor DFX for 0-6 h prior to nuclear extract preparation. HDAC2 was detected as a control. (H-I) HDAC5 knockdown represses HIF-1-stimulated lactate formation. Control or HDAC5 shRNA transduced HeLa cells (20,000) were seeded into Seahorse XF24 microplates. Cells were treated with DFX for 4 h to activate HIF-1. The ECAR was recorded (* p < 0.05, **p < 0.01, shR: shRNA).
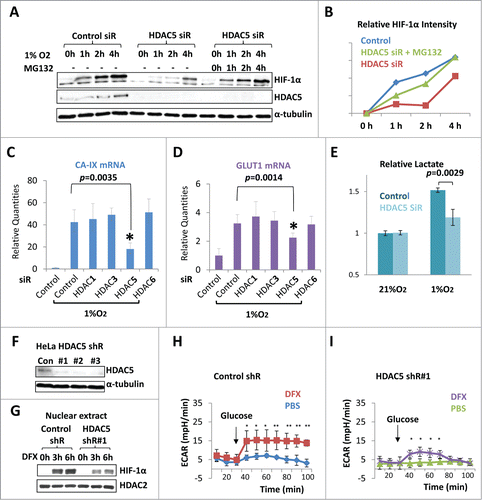
Figure 4. The cytosolic HDAC5 deacetylase activity is required for HIF-1α stabilization. (A) The schematic structures of HDAC5 and 4. (B) HDAC5-C698A/H704A mutant is deficiency of deacetylase. Hep3B cells were transfected with 2 μg of vectors, HDAC5-C698A/H704A or wt-HDAC5. (C) Deacetylase activity of HDAC5 is required for HIF-1α accumulation. Hep3B cells were transfected with HA-HIF-1α and 2 μg of vector, HDAC5-C698A/H704A or WT-Flag-HDAC5. HIF-1α and Flag-HDAC5 were examined. (D) Schematic structure of cytosolically and nuclearly localized HDAC5 mutants. Mutations were introduced in NLS (S278/279A) or NES (L1092A). (E) Subcellular localization of HDAC5 mutants. Hep3B cells were transfected with 2 μg of Flag-HDAC5-WT, HDAC5-L1092A or HDAC5-S278/279A. (F) The cytosolic HDAC5 is sufficient to stabilize HIF-1α. Cells were transfected with 2 μg of HA-HIF-1α and 2 μg of vector, Flag-HDAC5-WT, Flag-HDAC5-S278/279A (cytosol) or Flag-HDAC5-L1092A (nucleus). HIF-1α and Flag-HDAC5 were examined by Western blotting.
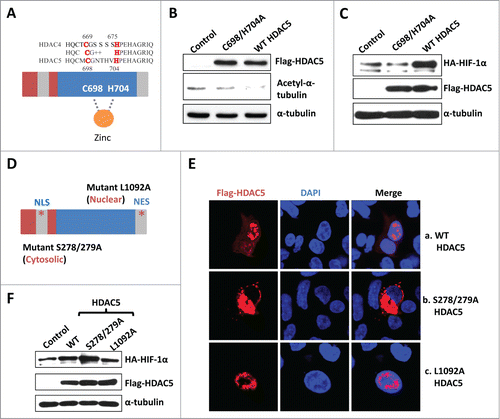
Figure 5. HDAC5 facilitates nuclear accumulation and potentiates the transactivation activity of exogenously expressed HIF-1α. (A) Posttranslational processes of HIF-1α. The cytosolic HIF-1α represents the portion undergoing posttranslational processes, while nuclear HIF-1α represents the mature form. (B-D) HDAC5 promotes nuclear localization of overexpressed HA-HIF-1α and potentiates its transactivation activity. Hep3B cells were transfected with 2 μg of HA-HIF-1α and 2 μg of flag-HDAC5. Cytosolic and nuclear extracts were separated and examined (B). Cells were stained with anti-Flag, anti-HIF-1α and DAPI. A representative microimage showing a cell expressing HIF-1α alone and a cell expressing both HIF-1α and HDAC5 in the same microscopic field was presented (C). The mRNA levels were determined by qRT-PCR (D). (F) HDAC5 inhibition blocks HIF-1α nuclear localization. Hep3B cells were cultured in 1% O2 or with DFX (100 μM) for 6 h in the presence or absence of 25 nM of LMK235.
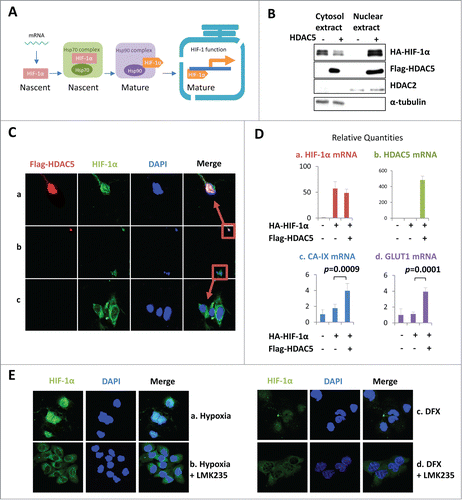
Figure 6 (See previous page). HDAC5 deacetylates Hsp70, attenuates Hsp70-HIF-1α interaction but enhances Hsp90-HIF-1α interaction. (A) Hep3B cells were treated as indicated and lysates were immunoprecipitated with anti-HIF-1α. The same blot was firstly used to detect Hsp90 and Hsp70, and then HIF-1α. MG132 (5 μM) was added to balance HIF-1α levels. (B) Hep3B cells were co-transfected with 2 μg of HA-HIF-1α and 2 μg of flag-HDAC5. Cell lysates were immunoprecipitated with anti-HIF-1α followed by detecting coimmunoprecipitated Hsp90 and Hsp70. (C) Hep3B cells were transfected with control or HDAC5 siRNA and exposed to 1% O2 in the presence of MG132 (5 μM) for 6 h. Cell lysates were immunoprecipitated with anti-acetyl-lysine antibody. Precipitated Hsp90 and Hsp70 were detected sequentially by Western blotting. (D-E) HDAC5 deacetylates Hsp70 in cultured cells. D. Hep3B cells were co-transfected with flag-HDAC5 and Flag-Hsp70, or Flag-HDAC3 and Flag-Hsp70. 5% of cell lysates were used to detect indicated proteins (left), and remaining cell lysates were immunoprecipitated with anti-Hsp70 or non-specific IgG. Acetyl-K and Flag-Hsp70 were detected sequentially. E. Hep3B cells were transfected with wt-HDAC5 or HDAC5C698/H704A mutant. Cell lysates were immunoprecipitated with anti-Hsp70 followed by examining Acetyl-K proteins and Hsp70. (F) HDAC5 directly deacetylates Hsp70 in vitro. Hep3B cells were transfected with Flag-Hsp70. Flag-Hsp70 was purified by immunoprecipitation with anti-flag antibody, and incubated with or without 60 ng of recombinant GST-HDAC5 (rHDAC5) at 37 °C for 30 min in deacetylase buffer. Acetylated Hsp70 and total Hsp70 were detected sequentially. (G) Overexpression of Hsp70 impairs HIF-1α accumulation, which is reversed by HDAC5. Cells were transfected with 2 μg of HA-HIF-1α. In addition, the following plasmids were cotransfected: (1) 2 μg control vector; (2) 1 μg Flag-Hsp70, 1 μg control vector; (3) 1 μg Flag-HDAC5, 1 μg control vector; and (4) 1 μg Flag-Hsp70 and 1 μg of Flag-HDAC5. (H) Schematic representing Hsp70 acetylation sites. (I) HDAC5 deacetylates Hsp70 at K559/561. From left to right, Hep3B cells were transfected with: 1. control siRNA+Hsp70 WT; 2. HDAC5 siRNA+Hsp70 WT; 3. control siRNA+Hsp70 K559/561R; 4. HDAC5 siRNA+Hsp70 K559/561R. Cells were exposed to 1% O2 for 6 h. Cell lysates were immunoprecipitated with anti-acetyl-K. (J) Deacetylation of Hsp70 at K559/561 enhances the stabilization of HIF-1α. Cells were transfected with empty vectors, Hsp70 or K559/561R as indicated and exposed to 1% O2 for 6 h. Hsp70 was immunoprecipitated with anti-Flag and associated HIF-1α was detected by Western blotting.
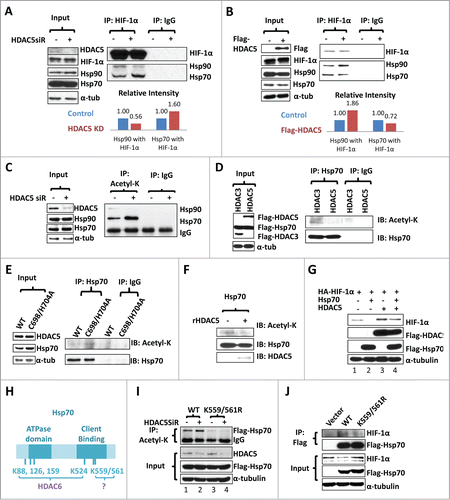
Figure 7. AMPK-triggered cytosolic shuttling of HDAC5 facilitates HIF-1α accumulation. (A) Effect of AMPK on HDAC5 subcellular localization. HeLa cells with stable Flag-HDAC5 were established and cultured in with 0.25 mM AICAR, or in 1% O2 for 6 h, or in glucose depleted medium (0 mM Glc) for 12 h. Cells were stained with anti-Flag and DAPI. (B) AMPK inhibition impairs hypoxic accumulation of HIF-1α. Hep3B cells were exposed to 1% O2 for 0–4 h in the presence of Compound C (Comp C, 20 μM) or DMSO. (C) Blocking nuclear export reduces hypoxic stabilization of HIF-1α. Hep3B cells were exposed to 1% O2 for 6 h in the presence or absence of LMB (20 ng/ml). (D) AMPK-facilitated HIF-1α stabilization depends on cytosolic localization of HDAC5. Hep3B cells were transfected with 2 μg of HA-HIF-1α and 2 μg of WT or the AMPK-resistant HDAC5 S259/498A mutant. Cells were cultured in glucose-free medium for 12 h. (E) AMPK enhances the ability of HDAC5 to stabilize HIF-1α. Hep3B cells were transfected with 2 μg of HA-HIF-1α and 2 μg of wt HDAC5 or cytosol-localized HDAC5 mutant (S278/279A). Cells were cultured in 21% or 1% O2 for 6 h, or in glucose free media for 12 h. (F-H) Hypoxia upregulates HDAC5 expression. Hep3B cells were cultured in 21% or 1% O2 for 6 h. HDAC5 and HIF-1α protein levels were determined by Western blotting (F). The mRNA levels of CA-IX (G: as positive control) and HDAC5 (H) were determined by qRT-RCR.
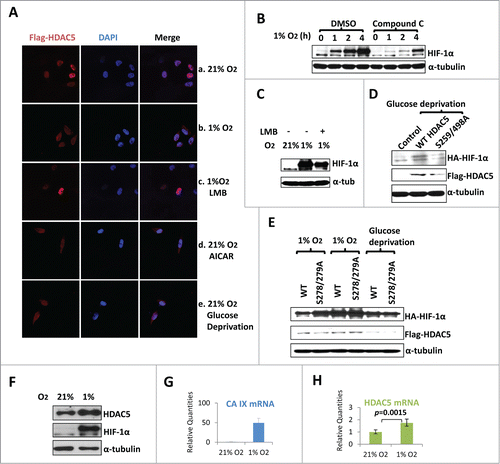
Figure 8 (See previous page). Inhibition of HDAC5 suppresses tumor cell proliferation under hypoxic conditions. (A) CRISPR-based knockdown of HDAC5 in Hep3B. (B) HDAC5 knockdown in Hep3B cells attenuates nuclear accumulation of HIF-1α. Control Hep3B or HDAC5 KD#5 cells were exposed to 1% O2 for 0, 3 and 6 h prior to nuclear extract preparation. HDAC2 were used as loading control. (C-F) Effects of HDAC5 knockdown on Hep3B cell survival and proliferation under hypoxic and/or low glucose conditions. HDAC5-KD or control Hep3B cells were seeded into 96-well microplates, each condition with 6 replicates. One microplate was measured 4 h after seeding. Other microplates were incubated in 21% or 1% O2 with indicated glucose concentrations and cell numbers were determined after 48 and 96 h culture. Both HDAC5-KD lines were tested and results are similar; and data from KD#5 cells are shown. (G, H) SiRNA-based HDAC5 knockdown represses HeLa cell proliferation under hypoxic conditions. HeLa cells were treated with HDAC5 siRNA or control siRNA. After 24 h and seeded into 96-well microplates, allowing 8 replicates for each experimental condition. One microplate was measured 4 h after seeding. The other microplates were cultured in 21% (G) or 1% (H) O2. Cell numbers were determined at 24, 48 or 72 h. (I, J) The inhibitory efficacy of HDACIs on tumor cell proliferation positively correlates to HDAC5 inhibition. Hep3B cells were seeded into 96 well microplates and cultured with various concentrations of LMK235 (I) or TSA (J) for indicated time. Cell proliferation inhibition was expressed as a percentage of the cell numbers obtained, with DMSO-treated negative control samples as 100%.
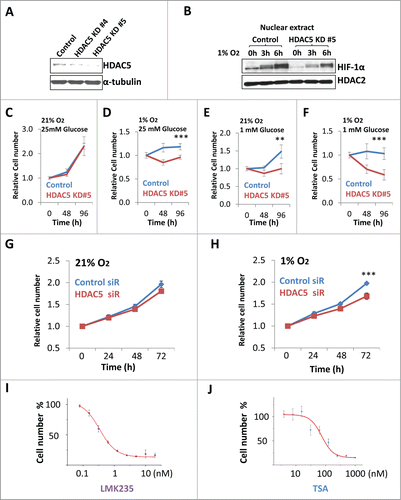
Figure 9. Updated model showing the role of AMPK-HDAC5 in HIF-1 activation as a response to hypoxia. Hypoxia-induced decrease of ATP level is couple to an increase of AMP levels, which activates AMPK. HDAC5 is phosphorylated by AMPK and exported to cytosol, where it deacetylate Hsp70, facilitates the transfer of Hsp70 associated HIF-1α to Hsp90 complex to complete the maturation process. Therefore, lack of cytosolic HDAC5 causes hyperacetylation of Hsp70, thereby deregulating Hsp70-HIF-1α interaction, leading to an increase of pre-mature HIF-1α in the cytosol and an inhibition of nuclear accumulation of HIF-1α.
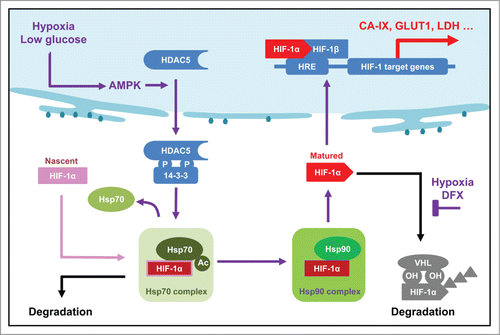