Figures & data
Figure 1. Isolation of Gyf/CG11148 as a genetic modifier of the Atg1-Atg13-gain-of-function eye phenotype. (A and B) Eyes from the flies expressing indicated transgenes were imaged using light dissection microscopy. (C) Schematic representation of the comparison among Drosophila and human Gyf orthologs. Amino acid sequence similarity is displayed as a percentage. (D) Phylogenic tree analysis of Gyf family proteins from Drosophila melanogaster (Dme), Caenorhabditis elegans (Cel), Anopheles gambiae (Aga), Apis mellifera (Ame), Danio rerio (Dre), Mus musculus (Mmu), and Homo sapiens (Hsa), constructed by the neighbor-joining algorithm. (E) Expression of Gyf at different developmental stages of Drosophila. Em, embryo; 1st, first instar larva; 2nd, second instar larva; 3rd, wandering-stage third instar larva; P, pupa; F, adult female; M, adult male. Quantitative reverse transcriptase (RT)-PCR was performed, and Gyf mRNA expression was normalized to ribosomal protein 49 (rp49) expression (upper panel, n = 3). Quantification data are represented as means ± standard error. Immunoblot analysis was performed to monitor Gyf (180 kDa) and Actin (40 kDa) expression. (F-I) Expression of Gyf in indicated 1-wk-old adult flies was examined through quantitative RT-PCR (F) and immunoblotting (G). Expression of 4EHP and pico in indicated 1-wk-old adult flies (H) or wandering-stage third instar larvae (I) was examined through quantitative RT-PCR. Tub>picodsRNA flies cannot develop into adulthood. Quantification data are represented as means ± standard error (n = 3). P values were calculated using the Student t test. *, P < 0.05; **, P < 0.01; NS, not significant. Immunoblot analysis was performed to monitor Gyf (180 kDa) and Actin (40 kDa) expression. (J) Eyes from the flies expressing the indicated transgenes were imaged using light dissection microscopy.
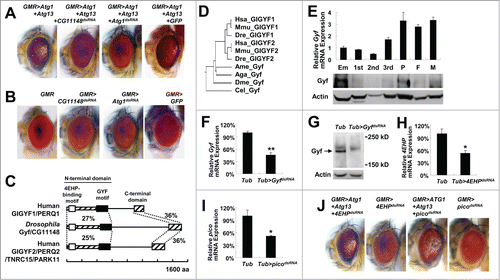
Figure 2. Gyf silencing suppresses Atg1-Atg13-induced eye degeneration and ectopic cell death. (A) Fly heads expressing indicated transgenic elements were subjected to immunoblotting to monitor the level of myc-Atg1 (Atg1, 120 kDa), Atg13-GFP (Atg13, 80 kDa), Actin (40 kDa), and GFP (25 kDa) expression. The level of Atg13 shift was quantified by densitometry of total and shifted Atg13 expression. Quantification data are represented as means ± standard error of the shifted/total Atg13 protein ratios (n=10). P values were calculated using the Student t test. *** P < 0.001; NS, not significant. (B) Eyes from the flies expressing indicated transgenes were analyzed by electron microscopy to visualize photoreceptor structures. Scale bars: 10 μm. (C and D) Developing eye discs from wandering-stage third instar larvae expressing indicated transgenes were analyzed by acridine orange (AO) staining (C) and LysoTracker Red staining (D) to visualize dying cells and autolysosomes, respectively. Brackets indicate the areas of differentiated ommatidia that express GMR-Gal4. Boxed regions in the top panel of (D) are magnified in the bottom panel to visualize the size of LysoTracker Red-positive vesicles.
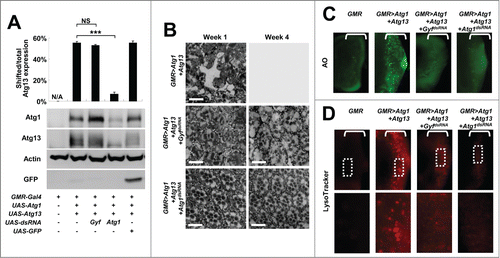
Figure 3. Gyf silencing interferes with Atg1-Atg13-induced autophagic flux. (A) Schematic representation of the GFP-mCherry-Atg8a autophagic flux reporter. (B) Due to the intrinsic nature of fluorescence proteins, GFP matures, fluoresces, and fades faster than mCherry. (C) Incorporation of GFP-mCherry-Atg8a into autolysosomes diminishes the fluorescence of GFP, but not that of mCherry. (D) Developing eye discs from wandering-stage third instar larvae expressing indicated transgenes and the GFP-mCherry-Atg8a reporter were observed using laser confocal microscopy. Brackets indicate the areas of differentiated ommatidia. (E–H) GFP and mCherry fluorescence intensities were measured from individual ommatidia expressing indicated transgenes and plotted into a graph against estimated time (h) after expression (n ≥ 15 in each time period) (E–G). GFP/mCherry fluorescence ratio at each time period was calculated (H). Data are represented as means ± standard error.
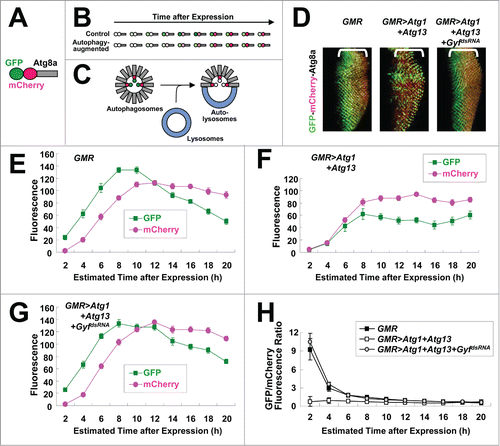
Figure 4. Gyf silencing does not interfere with the Atg6-Pi3K59F activity but inhibits Atg1-Atg13-induced Atg9 trafficking. (A and B) Developing eye discs from wandering-stage third instar larvae expressing indicated transgenes were observed under a fluorescence microscope to visualize Fyve-conjugated GFP (green) that monitors PtdIns3P. (C–E) Developing eye discs from wandering-stage third instar larvae expressing indicated transgenes were analyzed by anti-Atg9 (red) staining, which monitors trafficking of endosomes to autophagosomes. Boxed areas in the left-most panels are magnified in the middle panels. The right-most panels display fluorescence of Atg13-conjugated GFP (green) in the region corresponding to the left-most panel.
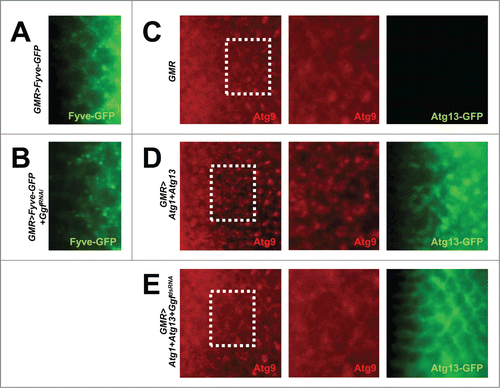
Figure 5. Gyf silencing does not interfere with apoptotic cell death. (A and B) Developing eye discs from wandering-stage third instar larvae expressing indicated transgenes were analyzed by acridine orange (AO, upper panels) and TUNEL (lower panels) staining to visualize apoptotic cells. Brackets indicate the areas of differentiated ommatidia. (C) Eyes from the flies expressing indicated transgenes were imaged using light dissection microscopy. GMR>rpr flies cannot develop into adulthood.
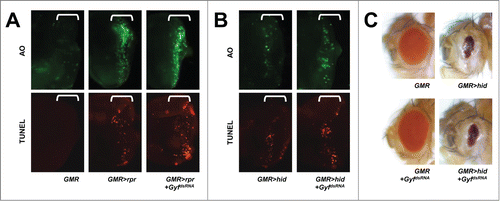
Figure 6. Gyf mutant flies exhibit shortened life span and mobility defects. (A) Schematic genomic organization of the Gyf (CG11148) locus and Gyf mutants. Triangles, transposon insertions; open boxes, untranslated exons; closed boxes, protein-coding exons. Scale bar, relative length of 1-kb genomic span. (B and C) Absence of Gyf expression in Gyf-null flies (GyfMI). RT-PCR of Gyf and rp49 (B) and immunoblotting of Gyf (180 kDa) and Tubulin (50 kDa) (C) were conducted from the flies of indicated genotypes. (D) Survivorship of WT (w1118 control), Gyf-null mutant (GyfMI) and Gyf-null mutant with Gyf genomic rescue (GyfMI; DpGyf) male flies (n ≥ 180). Survival of 4 independent cohorts is presented as mean ± standard error. (E–G) Photographs of the vials containing 2- or 4-wk-old adult male flies of indicated genotypes taken at 3 sec after negative geotaxis induction. (H) Quantification of the climbing speeds of indicated 2- or 4-wk-old adult male flies (n ≥ 80). Climbing speed is presented as mean ± standard error. P values were calculated using the Student t test. *** P < 0.001; NS, not significant.
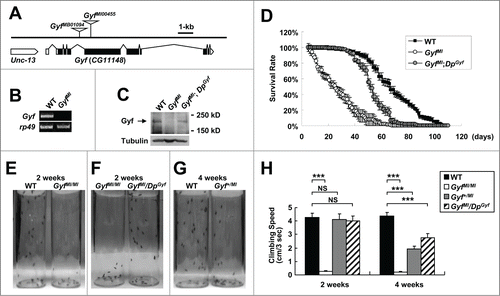
Figure 7. Gyf is essential for developmental, starvation-induced and physiological autophagy. (A–H) Fat bodies (FB) of wandering-stage third instar larvae (A–D) or feeding-stage third instar larvae that were placed on 20% sucrose solution for 3 h (E–H) of WT and GyfMI/MI flies were subjected to anti-Atg8a immunostaining (A, C, E and G) or LysoTracker Red (Lys) staining (B, D, F and H). Hoechst 33258 (DNA) was used to visualize nuclei. Scale bars: 50 μm. Number of Atg8a or LysoTracker Red puncta per cell in fat bodies are presented as means ±standard error (n ≥ 40) (C, D, G and H). (I–L) Heads (I and J) and thoraxes (K and L) of 1-wk-old WT and GyfMI/MI flies were analyzed through immunoblotting of Atg8a-I (16 kDa), Atg8a-II (14 kDa) and Actin (40 kDa). The ratios of Atg8a-II to Atg8a-I are presented as means ± standard error (n = 7) (J and L). (M and N) Thoraxes of 1-wk-old WT and GyfMI/MI flies were analyzed through immunoblotting of phospho-Thr398 S6k (70 kDa), phospho-Ser505 Akt1 (60 and 70 kDa), Atg1 (120 kDa) and Actin (40 kDa). The immunoblot results were quantified and presented as means ± standard error (n = 4). P values were calculated using the Student t-test. *, P < 0.05; **, P < 0.01; ***, P < 0.001; NS, not significant.
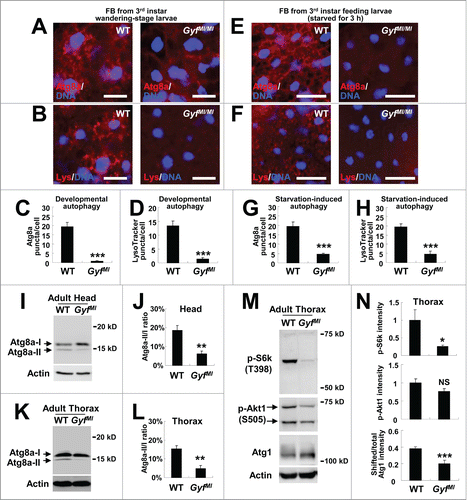
Figure 8. Accumulation of ubiquitinated proteins and damaged mitochondria in Gyf mutant flies. (A and B) 2-wk-old adult male flies of indicated genotypes were subjected to immunoblot analyses with ubiquitin and tubulin (50 kDa) antibodies (A). Relative protein level was quantified and presented as mean ±standard error (B). (C and E) Electron micrographs reveal the presence of dysfunctional mitochondria (green arrows) in the brain (C, optic lobe medulla) and the skeletal muscle (E) of 4-wk-old Gyf mutant flies. Boxed areas with normal or damaged mitochondria are magnified in lower (C) or right (E) panels. In the skeletal muscle of Gyf mutant flies, broadened Z-bands (black arrows) were frequently observed. Scale bars: 500 nm. (D, F and G) Quantification data are represented as means ± standard error (n ≥ 3). P values were calculated using the Student t test. **, P < 0.01; ***, P < 0.001; NS, not significant.
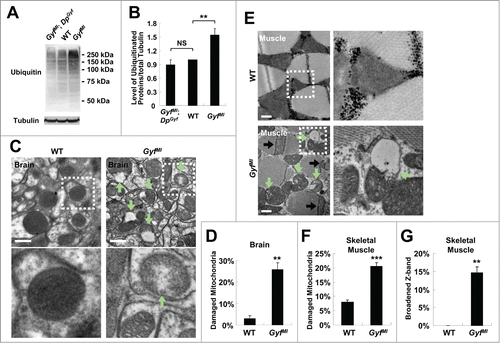
Figure 9. Degenerative phenotypes of brain and muscle tissues of Gyf mutant flies. (A–D) TUNEL (red) and DAPI (blue, DNA) staining reveals apoptotic cells in the brain and skeletal muscle of 4-wk-old Gyf mutant flies. Right panel images are corresponding to the boxed areas in the left panel images (A and B). Scale bars: 200 μm (white), 50 μm (gray).
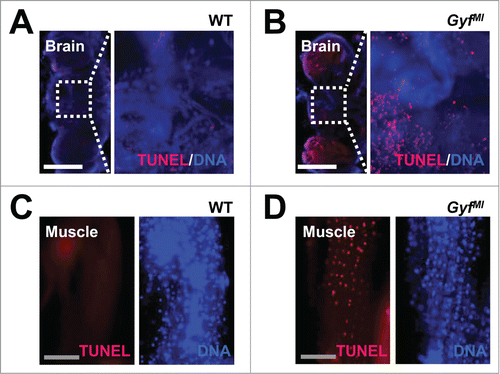