Figures & data
Figure 1. Evaluation of autophagosomes in WT and bs MEFs. WT (A) and bs (B) MEFs 24 h following transfection with GFP-LC3 (red). Nuclei were stained with DAPI (blue). Scale bar: 5 μm. (C) Quantification analysis revealed significantly greater (P = 0.0001; n = 30) percentage area positive for GFP-LC3 in bs MEFs when compared to WT MEFs. TEM images of WT (D) and bs (E) MEFs revealed an accumulation of double-membraned autophagosomal structures (red arrowheads) in bs MEFs. Some autophagosomes in bs MEFs (E) were observed as a part of larger multi-lamellar double-membrane structures (blue arrowheads). Scale bar: 100 nm. (F) Immunoblotting of WT and bs MEF cell lysates for LC3; immunoblotting for ACTB served as a loading control. (G) Quantification analysis confirmed a significant accumulation (P = 0.0008; n = 3) of endogenous LC3-II in bs MEF cell lysates when normalized to ACTB whereas levels of LC3-I:ACTB did not significantly differ (P = 0.2381; n = 3). The data in (C) and (G) are presented as the mean ± SEM and significance was established with the Student t test.
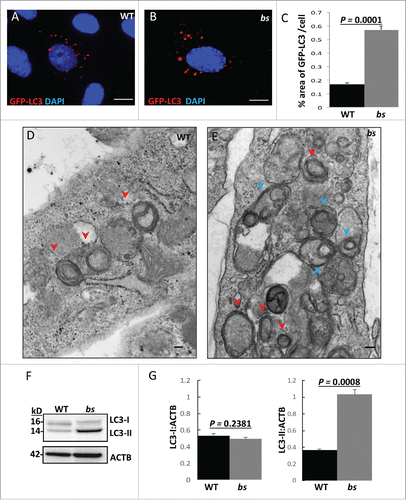
Figure 2. Autophagic flux in WT and bs MEFs. Immunostaining of WT (A) and bs (B) MEFs for SQSTM1. (C) Quantification analysis revealed a significantly greater (P = 0.0008; n = 30) percentage area of SQSTM1 per cell in bs MEFs when compared to WT MEFs. (D) Western blot for SQSTM1 of lysates from WT and bs MEFs; immunoblotting for ACTB served as a loading control. (E) Quantification analyses revealed a significantly greater (P = 0.0002; n = 3) levels of SQSTM1:ACTB in lysates from bs MEFs when compared to WT MEFs. (F) Immunoblotting of WT and bs MEF cell lysates for SQSTM1 following 2-h treatment with DMSO as vehicle control (lanes 1 and 5), serum-free and amino-acid free media (ST) (lanes 2 and 6), 100 nM bafilomycin A1 (BF) in complete medium (lanes 3 and 7), and combined treatment with bafilomycin A1 and serum-free and amino-acid free media (BF+ST) (lanes 4 and 8); immunoblotting for ACTB served as a loading control. (G) Quantification analysis revealed significantly greater levels (P = 0.0027; n = 3) of SQSTM1:ACTB in lysates from bs MEFs when compared to WT following treatment with DMSO (P = 0.0027; n = 3) or ST (P = 0.0001; n = 3). No significant differences were identified between WT and bs MEFs following treatments with BF (P = 0.0969; n = 3), and BF+ST (P = 0.097; n = 3). In WT MEFs ST significantly (P = 0.0049; n = 3) lowered SQSTM1 levels whereas BF and BF+ST significantly increased (P = 0.0013 and P = 0.0007 respectively; n = 3) levels of SQSTM1 when compared to DMSO treatment; by contrast SQSTM1 levels in bs MEFs did not significantly change following DMSO treatment and ST (P = 0.31; n = 3), BF (P = 0.851; n = 3) and ST+BF (P = 0.3234; n = 3).
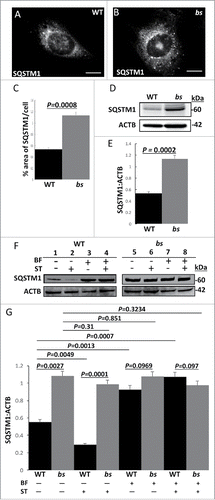
Figure 3. Autophagosome maturation in WT and bs MEFs. (A-A″) WT MEFs expressing tandem mCherry-EGFP-LC3 fusion protein revealed a mix of pH neutral autophagosomes (“yellow” = mCherry+EGFP tags) and acidic amphisomes and autolysosomes (“red-only” = mCherryΔEGFP tag). (B-B”) In bs MEFs mCherry-EGFP-LC3 fusion protein was arrested in enlarged pH neutral autophagosomes (“yellow”) indicating a failure of mCherry-EGFP-LC3 to progress to acidic amphisomes and autolysosomes. Nuclei were stained with DAPI (blue). Scale bars in all images: 5 μm. (C) Quantification analysis of WT and bs MEFs expressing mCherry-EGFP-LC3 tandem protein revealed significantly greater (P = 0.0001; n = 30) percentage area positive for both EGFP and mCherry (“yellow” = mCherry+EGFP) in bs MEFs; quantification analysis also revealed significantly lower (P = 0.0001; n = 30) percentage area of only mCherry that is not positive for EGFP (“red only” = mCherryDEGFP) in bs MEFs. LysoTracker Red-positive structures did not appear to differ between WT (D) and bs (E) MEFs. The area of LysoTracker Red-positive staining per cell (F) did not significantly differ (P = 0.6344; n = 30) between the 2 genotypes. (G) Western blot analysis of cell lysates for EGFR and ACTB from WT and bs MEFs treated with 40 ng/mL EGF and 20 μg/mL cycloheximide for 0, 30, 60 and 120 min. (H) Quantification analysis did not reveal a significant difference in the levels of EGFR relative to ACTB between WT and bs MEFs in untreated cells (P = 0.1854; n = 3), 30 min (P = 0.67; n = 3), 60 min (P = 02636; n = 3) or 120 min (P = 1; n = 3) following the treatment with EGF and cycloheximide. The data in (C), (F) and (H) are presented as the mean ± SEM and significance was established with the Student t test.
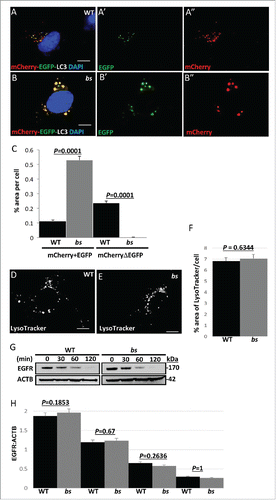
Figure 4. TBC1D20 is a regulator of RAB1B during autophagy. WT (A-A') and bs (B-B') MEFs following transfection with plasmids encoding GFP-LC3 (red) and FLAG-RAB1B (green). WT MEFs (C-C') following transfection with plasmids encoding GFP-LC3 (red) and FLAG-RAB1BQ67L (green). bs (D-D') and WT MEFs (E-E') following transfection with GFP-LC3 (red) and FLAG-RAB1BN121I (green). Nuclei were stained with DAPI (blue). Scale bar in all images: 5 μm. Quantification analysis (F) revealed significantly greater (P = 0.0059; n = 30) percentage area positive for GFP-LC3 in bs MEFs when compared to WT MEFs following transfection with FLAG-RAB1B. Additionally, WT MEFs overexpressing FLAG-RAB1BQ67L also exhibited significantly greater (P = 0.0107; n = 30) percentage area of GFP-LC3 when compared to WT MEFs overexpressing FLAG-RAB1B. The percentage area of GPF-LC3 per cells did not significantly differ (P = 0.172; n = 30) between bs MEFs overexpressing FLAG-RAB1B and WT MEFs overexpressing FLAG-RAB1BQ67L. Both WT and bs MEFs overexpressing FLAG-RAB1BN121I exhibited significantly lower (P = 0.004 and P = 0.0039, respectively) percentage area of GFP-LC3 per cell when compared to WT MEFs transfected with FLAG-RAB1B. The percentage area of GFP-LC3 per cell did not significantly differ (P = 0.84; n = 30) between WT and bs MEFs transfected with FLAG-RAB1BN121I.
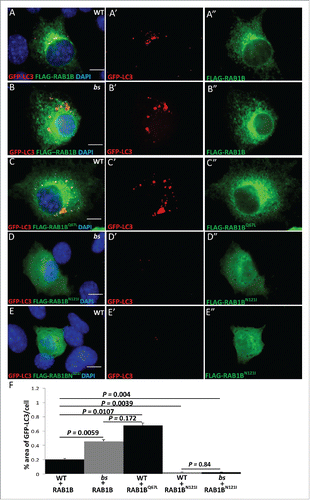
Figure 5. Autophagic cargo in WT and bs MEFs. Immunostaining of WT (A) and bs (B) MEFs for ubiquitin. Immunoblotting of WT and bs cell lysates (C) confirmed accumulation of ubiquitinated proteins in bs MEFs; immunoblotting for ACTB served as a loading control. Immunostaining of WT (D) and bs (E) MEFs for ABCD3 as a peroxisomal marker; quantification of ABCD3-positive puncta (F) confirmed a significant (P = 0.0001; n = 30) accumulation of ABCD3 in bs MEFs. Immunostaining of WT (G) and bs (H) MEFs for COX4I1 as a mitochondrial marker. Western blotting of WT and bs lysates for TOMM20 as a mitochondrial marker revealed in bs lysates significantly greater (P = 0.0063; n = 3) levels of TOMM20 relative to ACTB (I). Staining of WT (J) and bs (K) MEFs with the mitochondrial dye MitoTracker Red established that accumulating mitochondria in bs MEFs remained polarized. Nuclei in all images were stained with DAPI. Scale bar: 5 μm. The data in (F) and (I) are presented as the means ± SEM and significance was established with the Student t test.
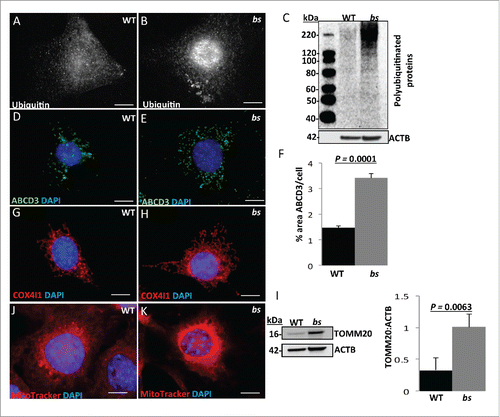
Figure 6. Autophagy in P12 WT and bs lenses. Clinical evaluation with a slit lamp biomicroscope of WT (A) (n = 6) eyes revealed a transparent lens core (black arrowhead) and a transparent lens cortex (blue arrow); in bs (B) (n = 6) eyes the lens core exhibited cataracts (black arrowhead) whereas the lens cortex remained transparent. H&E evaluation of WT (C) lenses revealed organized epithelium at the lens anterior (black arrow), elongated lens fiber cells (blue arrow) within the cortex and anucleated lens fiber cells within the core (black arrowhead). H&E evaluation of bs (D) lenses (n = 6) identified organized lens epithelial cells at the anterior (black arrow), elongated fiber cells within the lens cortex (blue arrow) and highly degenerated fibers within the lens core (arrowhead). Immunostaining of WT lenses (n = 6) for SQSTM1 (E) and ubiquitin (G) revealed the presence of both SQSTM1 and ubiquitin in lens epithelial cells (white arrows) and cortical fibers (blue arrows) without any staining present in the nuclear fibers (white arrowheads). By contrast immunostaining of bs lenses (n = 6) revealed accumulation of SQSTM1 (F) and ubiquitin (H) in bs cortical lens fibers (blue arrows) and nuclear lens fiber cells (white arrowheads). DAPI staining of WT (n = 6) (I) and bs (n = 6) (J) lenses revealed nuclei in cortical fibers (blue arrows). In WT lenses fibers within the lens core did not stain for DAPI indicating the removal of nuclei (I, white arrowheads) whereas bs fiber cells within the lens core stained for DAPI indicating a failure in the removal of nuclei (J, white arrowhead). Images (I') and (J') are a higher magnification of images (I) and (J) and arrows in (J) point to smaller nuclei retained in bs lens fiber cells within the OFZ. In (E) and (F) nuclei were stained with DAPI (blue). Scale bars: 50 μm. OFZ, organelle-free zone.
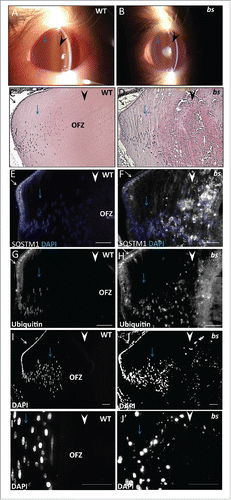
Figure 7. Evaluation of WT and bs seminiferous tubules. Immunostaining of WT seminiferous tubules (n = 10) for LC3 (A), LAMP1 (C), SQSTM1 (E) and ubiquitin (G) revealed crescent-shaped staining indicative of acrosomes. By contrast immunostaining of bs seminiferous tubules (n = 10) for LC3 (B), LAMP1 (D), SQSTM1 (F) and ubiquitin (H) revealed diffuse and disorganized staining. Nuclei were stained with DAPI. L, lumen. Scale bar: 50 μm.
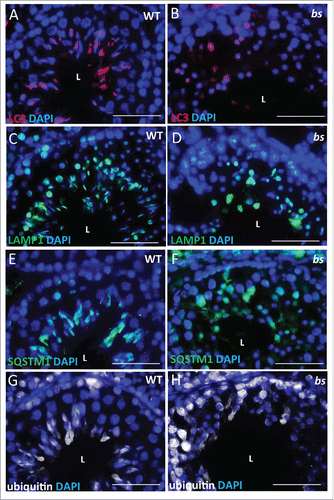
Figure 8. Neuronal phenotypes in WT and bs mice. At 5 mo of age bs mice (n = 3) (B) exhibit hindlimb clasping phenotypes in contrast to splaying of hindlimbs in age-matched WT littermates (n = 3) (A). At 5 mo bs mice exhibited abnormal gait (D) characterized by lower abdomen and feet positioned outward in contrast to age-matched WT animals (C) that display alternate limb movements with feet positioned forward. Western blot analysis of lysates from adult WT and bs brains revealed a significant (P = 0.0055; n = 3) accumulation of SQSTM1 (E) as well as a significant (P = 0.01; n = 3) accumulation of ubiquitin (F) in bs brains; immunoblotting for GAPDH in (E) and ACTB in (F) served as loading controls.
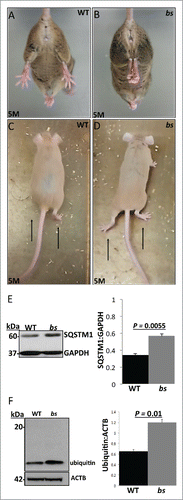
Figure 9. A proposed model for TBC1D20 function during autophagy. TBC1D20 mediates maturation of autophagosomes via its RAB1B GAP function, a process required for the fusion of autophagosomes with late endosomes to form amphisomes as well as a direct fusion of autophagosomes with lysosomes to form autolysosomes, thereby facilitating autophagic flux and delivery and subsequent degradation of autophagic cargo in autolysosomes.
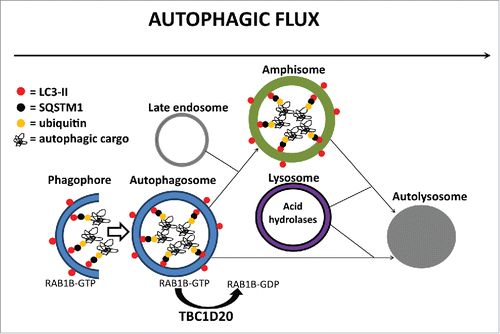