Figures & data
Figure 1. Schematic overview of four types of modifications of liposomal formulations. Conventional liposomes are composed of cationic, anionic, or neutral phospholipids and cholesterol, and enclose and aqueous core (a). These liposomes can be loaded with hydrophobic as well as hydrophilic agents. PEGylated liposomes to prolong the conventional liposomes (b). Targeted liposomes to which proteins, peptides, antibodies, carbohydrates or small molecules can be attached to enable specific targeting of the liposomes and their content (c). Theranostic liposomes consist of a liposome, an imaging agent, a therapeutic component and a targeting ligand (d) (Reproduced with permission from Sercombe et al. [Citation29]).
![Figure 1. Schematic overview of four types of modifications of liposomal formulations. Conventional liposomes are composed of cationic, anionic, or neutral phospholipids and cholesterol, and enclose and aqueous core (a). These liposomes can be loaded with hydrophobic as well as hydrophilic agents. PEGylated liposomes to prolong the conventional liposomes (b). Targeted liposomes to which proteins, peptides, antibodies, carbohydrates or small molecules can be attached to enable specific targeting of the liposomes and their content (c). Theranostic liposomes consist of a liposome, an imaging agent, a therapeutic component and a targeting ligand (d) (Reproduced with permission from Sercombe et al. [Citation29]).](/cms/asset/c97d0cd2-1711-4fd9-a6b9-c0c82b5c7cee/iedd_a_1205584_f0001_oc.jpg)
Figure 2. (a) SPECT image acquisition. The radionuclide emits γ-rays. The photons are collimated by the lead collimator and reach the sodium-iodine (NaI) chrystal, which produce scintillation. The scintillation is converted into an electronic signal, which is stored digitally to be processed to form the actual image. (b) Coincidence principle of PET. A radionuclide emits a positron, which annihilates with an electron. This results in two γ-photons of 511 keV that are emitted in opposite direction. These photons are detected in the ring of detectors and the activity distribution can be reconstructed into an image.
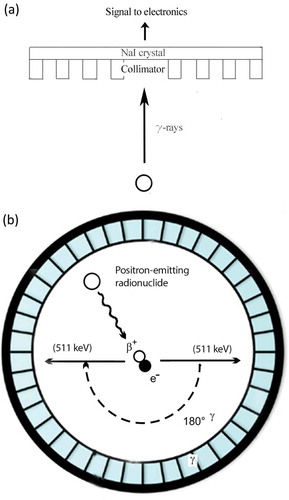
Figure 3. Schematic overview of the three radiolabelling approaches. Passive encapsulation of the radionuclides during preparation using a chelator (DOTA), liposomal membrane labelling during preparation of the liposomes, remote loading of radionuclides into preformed liposomes via ionophores or using lipophilic chelators (2-hydroxyquinoline), and surface labelling after incorporation of a chelator (DOTA) to the lipid bilayer or PEG are shown. In this figure, radionuclides are represented by the open circles.
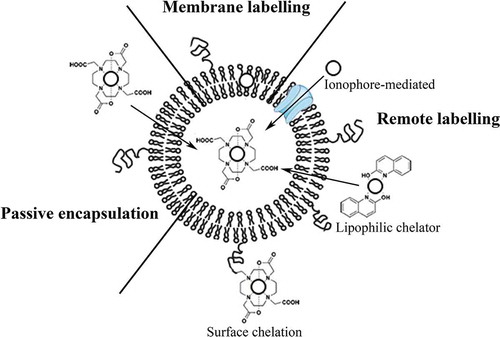
Table 1. Overview of radiolabeling methods.
Figure 4. MicroSPECT/CT images of a mouse with a S. Aureus abscess in the left thigh muscle. Images were acquired at 1 h, 4 h, 24 h, 48 h and 72 h after injection of 111In-labeled PEGylated liposomes. At all time points uptake in the abscess and the characteristic splenic and hepatic uptake is visualized (arrows) (Reproduced with permission from van der Geest et al. [Citation49]).
![Figure 4. MicroSPECT/CT images of a mouse with a S. Aureus abscess in the left thigh muscle. Images were acquired at 1 h, 4 h, 24 h, 48 h and 72 h after injection of 111In-labeled PEGylated liposomes. At all time points uptake in the abscess and the characteristic splenic and hepatic uptake is visualized (arrows) (Reproduced with permission from van der Geest et al. [Citation49]).](/cms/asset/2cf9987f-0b49-4833-af2a-9360ce2c3f65/iedd_a_1205584_f0004_oc.jpg)
Figure 5. MicroPET/CT-images of a mouse bearing human colon adenocacinoma (HT29, arrows) on their right and left flank. Image acquisition was performed 24 h post injection of 64Cu-labelled PEGylated liposomes (a) Coronal PET image showing both tumours (arrows). The characteristic uptake of liposomes in spleen and liver is also visualized (arrowheads). (b) Axial PET image (top) and PET/CT image (bottom), showing both tumours (arrows) (Reproduced with permission from Petersen et al. [Citation56]).
![Figure 5. MicroPET/CT-images of a mouse bearing human colon adenocacinoma (HT29, arrows) on their right and left flank. Image acquisition was performed 24 h post injection of 64Cu-labelled PEGylated liposomes (a) Coronal PET image showing both tumours (arrows). The characteristic uptake of liposomes in spleen and liver is also visualized (arrowheads). (b) Axial PET image (top) and PET/CT image (bottom), showing both tumours (arrows) (Reproduced with permission from Petersen et al. [Citation56]).](/cms/asset/3dff9a7e-6365-4c7f-94e3-fbc518af7ba6/iedd_a_1205584_f0005_oc.jpg)