Figures & data
Table 1. Scopus.com database query terms.
Figure 1. Number of original articles and reviews published between 2010 and 2023 in the organ-on-a-chip field. (a) Publication rate of articles and reviews with the terms organ, or body and multi tissue -on-a-chip over the last decade and a half (b) and detailed view on articles and reviews for the terms body- and multi tissue-organ-on-a-chip (red area represents publications containing the keywords for SARS-CoV-2). (c) Publications of common organ-on-a-chip models over the last 24 years. (d) Term distribution of the publication number of various organ-on-a-chip models before and after the year 2020. Yellow, colored background shows the division between pre- and post-pandemic research. Information was gathered through SCOPUS query displayed in .
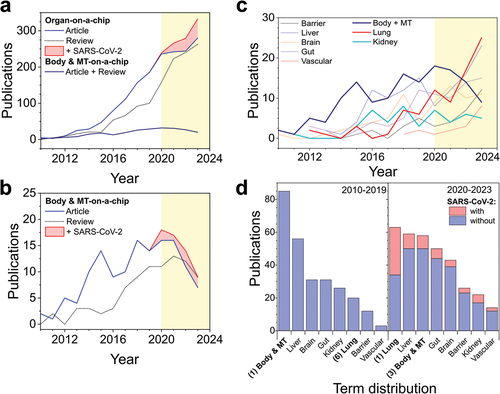
Table 2. Overview of the discussed papers.
Figure 2. Overview on three multi-tissue on-a-chip models. (ai) Design of a liver-heart chip with a polycarbonate membrane between cell types. (aii) Seeding assay to create liver and heart organoids in unique compartments inside the chip. (aiii) Effect of 1 µM Clomipramine on the beating velocity of the heart spheroids after liver metabolism. (aiv) Effect of 1 µM Clomipramine on the Ca2+ ion production of the heart spheroids in different culture conditions. (bi) Seeding procedure of pancreatic islets and liver spheroids into a pneumatic chip. (bii) Overlap of differentially expressed genes in liver spheroids and pancreatic islets after high glucose exposure in chip. (ci) 3 organ-on-a-chip system developed for malaria studies together with a flow profile simulation of the system. (cii) Over-time hepatocyte morphology and viability assay (via CYP3A4 activity) while inside.
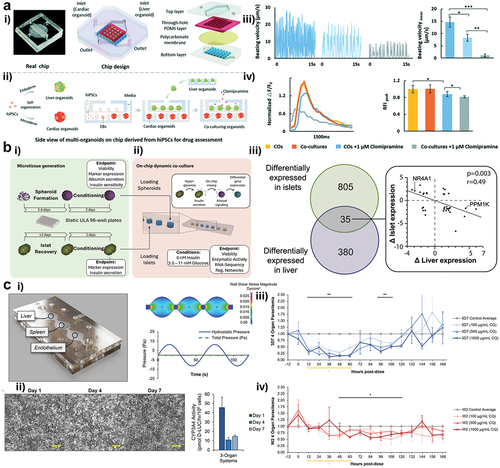
Figure 3. Overview of a humanized multi-organ on-a-chip platform for drug metabolism and toxicity testing. (ai) Schematic designs for 3 and 6 integrated organoid systems. (aii) Pipetting assay schematics for a thiolated-hydrogel-based cell 3D culture. (aiii) Photography of a multi-organ system with external fluid flow control. (b) Viability study of the effects of ifosfamide on a 6-organoid system (bi) Counterstain of life (calcein AM) and death cells (ethidium homodimer 1) of all 6 organs in the system. condition 1 and 2 are treatment with 1 mM ifosfamide but in condition 2 the liver was removed before treatment. (bii) Quantification of life/dead staining, viability is shown as percentage. [Adapted with permission from [Citation43]].
![Figure 3. Overview of a humanized multi-organ on-a-chip platform for drug metabolism and toxicity testing. (ai) Schematic designs for 3 and 6 integrated organoid systems. (aii) Pipetting assay schematics for a thiolated-hydrogel-based cell 3D culture. (aiii) Photography of a multi-organ system with external fluid flow control. (b) Viability study of the effects of ifosfamide on a 6-organoid system (bi) Counterstain of life (calcein AM) and death cells (ethidium homodimer 1) of all 6 organs in the system. condition 1 and 2 are treatment with 1 mM ifosfamide but in condition 2 the liver was removed before treatment. (bii) Quantification of life/dead staining, viability is shown as percentage. [Adapted with permission from [Citation43]].](/cms/asset/f76e7807-d6aa-4088-926a-cb8639d8bbaf/iemt_a_2362183_f0003_oc.jpg)
Figure 4. (a) Design of a multi-organ platform with shared fluid dynamics. (ai) final assembled platform. (aii) top and bottom views of the platform. (aiii) Schematic for modular connections of various multi-organ platforms. (aiv) alternative design for mono- and bi-organ cultures. (av) Computational simulation of shear stress on the system. (b) Cardiac tissues after maturation, (bi) α-actin alignment. (bii) Capture rate (biii) Excitation threshold. (biv) force response depending on calcium concentration. (c) Liver tissues after maturation (ci) Immunohistochemical staining images, (cii) Albumin secretion. (di) Immunohistochemical staining of engineered bone slices. (dii) Bone density over time. (ei) Immunohistochemical staining of engineered skin slices. (eii) Skin barrier function measured via TEER. [Adapted with permission from [Citation38]].
![Figure 4. (a) Design of a multi-organ platform with shared fluid dynamics. (ai) final assembled platform. (aii) top and bottom views of the platform. (aiii) Schematic for modular connections of various multi-organ platforms. (aiv) alternative design for mono- and bi-organ cultures. (av) Computational simulation of shear stress on the system. (b) Cardiac tissues after maturation, (bi) α-actin alignment. (bii) Capture rate (biii) Excitation threshold. (biv) force response depending on calcium concentration. (c) Liver tissues after maturation (ci) Immunohistochemical staining images, (cii) Albumin secretion. (di) Immunohistochemical staining of engineered bone slices. (dii) Bone density over time. (ei) Immunohistochemical staining of engineered skin slices. (eii) Skin barrier function measured via TEER. [Adapted with permission from [Citation38]].](/cms/asset/7d773708-1ef0-4e00-8eda-e340b21f52e8/iemt_a_2362183_f0004_oc.jpg)