Figures & data
Figure 1. Bispecific antibodies targeting CD47 and CD20 bind to each antigen alone: (A) Schematic of a CD20-CD47 DVD-Ig molecule with each variable domain of anti-CD20 (red) connected to each variable domain of anti-CD47 (blue) through a short or long amino acid linker. Constant regions are human Constant Light (kappa) and CH1, CH2, CH3 from human IgG1. (B) SDS-PAGE analysis of purified antibodies under non-reducing (left) and reducing (right) conditions. Sizes of heavy chain (HC) and light chain (LC) for each antibody type are indicated. (C) Antibodies were used to stain rat YB2/0 cells engineered to express human CD20, but not human CD47 (left) or YB2/0 cells engineered to express human CD47, but not human CD20 (right) prior to detection with PE-conjugated anti-human secondary antibody by flow cytometry. The experiment was performed 3 times with similar results. (D) Kinetic association and dissociation parameters, along with calculated affinity (KD) were measured by surface plasmon resonance using Biacore for anti-CD47 (B6H12-hIgG4), CD20-CD47 SL, or CD20-CD47 LL for human CD47. (E) CD20+CD47- YB2/0 cells were incubated with the indicated antibodies over a range of concentrations prior to staining with 10 μg/ml DyLight 488 (DL488) anti-CD20. Mean fluorescence intensity (MFI) of the DL488 signal was measured by flow cytometry. Data are representative of 3 independent experiments performed in triplicate. (F) CD20-CD47+ YB2/0 cells were incubated with the indicated antibodies over a range of concentrations prior to staining with 10 μg/ml Alexa Fluor 647 (AF647) anti-CD47. Mean fluorescence intensity (MFI) of the AF647 signal was measured by flow cytometry. Data are representative of 3 independent experiments performed in triplicate.
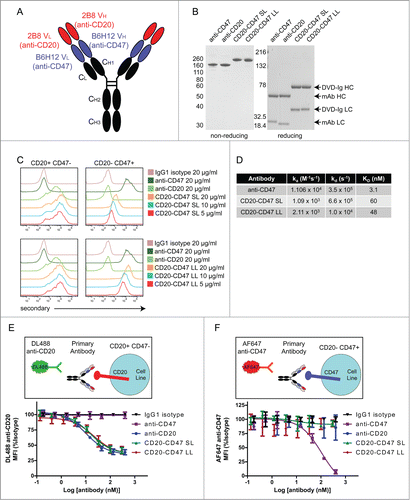
Figure 2. Simultaneous binding to CD47 and CD20 contributes to BsAb binding to cells: (A) Schematic of experimental design to detect simultaneous binding to CD20 and CD47. Biotinylated CD47 antigen was linked to a fluorescent (DyLight 650) neutravidin tetramer, which was co-incubated with unlabeled test antibody and CD20+CD47- YB2/0 cells. Test antibody binding was detected by staining with PE-conjugated anti-human secondary antibody. PE+DyLight 650+ live cells indicate simultaneous binding of antibody to both antigens. (B) Double positive events were detected by flow cytometry and represent simultaneous binding to CD47 and CD20. ****p < 0.0001, t test, (n = 6), error bars ± SEM. (C) CD20+CD47+ Raji cells were incubated with the indicated primary antibodies at 10 μg/ml prior to staining with 10 μg/ml AF647 anti-CD47. MFI of the AF647 signal was determined by flow cytometry and all test antibodies were compared to isotype control. ***p < 0.001; ns, not significant, t test, (n = 4), error bars ± SEM. (D) CD20+CD47+ Raji cells were incubated with the indicated primary antibodies at 10 μg/ml prior to staining with 10 μg/ml DyLight 488 anti-CD20. MFI of the DyLight 488 signal was determined by flow cytometry and all test antibodies were compared to isotype control. ***p < 0.001; ns, not significant, t test, (n = 4), error bars ± SEM.
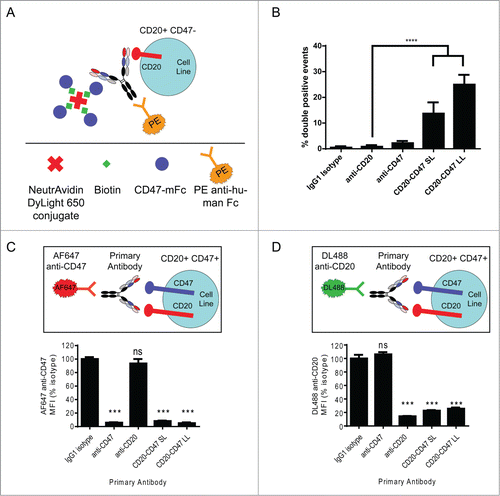
Figure 3. CD20-CD47 SL selectively binds to dual antigen-expressing cells in the presence of an antigen sink: (A) Schematic of the experimental design to assay for selectivity in binding to dual antigen-expressing cells in the presence of an excess of CD47-only expressing cells. CLL cells were labeled with CFSE and mixed with a 20-fold excess of CD20-CD47+ human red blood cells (RBCs). Cell mixtures were incubated with primary antibody prior to staining with PE anti-human Fc secondary and AF647 anti-CD47, and analysis by flow cytometry. (B) Two primary CLL samples were stained for CD47 and CD20 expression to identify the percentage of dual antigen-expressing cells by flow cytometry. (C) CLL cells were distinguished from RBCs on the basis of CFSE uptake. Primary antibody was used at 10 μg/ml and binding to cells was detected with PE secondary antibody staining. (D) Percentages of CLL cells (CFSE+) and RBC (CFSE-) within the antibody bound (PE+) population. A single replicate from each donor was averaged together (n = 2). (E) Binding of AF647 anti-CD47 to CLL cells from panel C is reported as MFI normalized to isotype control. A single replicate from each donor was averaged together (n = 2).
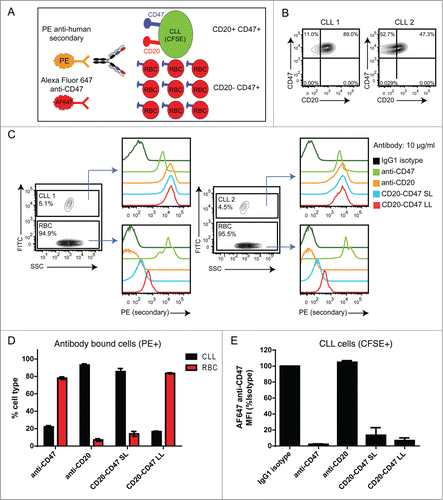
Figure 4. BsAbs induce phagocytosis of target cells in an FcR-dependent manner and recapitulate the synergy of antibody combinations: (A) Phagocytosis of CD20+CD47+CFSE+ cell lines by donor-derived human macrophages was assessed by flow cytometry. All antibodies were used at a final concentration of 10 μg/ml. Anti-CD47 is B6H12-mIgG1. The assay was performed with macrophages derived from 3 independent donors. The percentage of CFSE+ macrophages was normalized to the maximal response for each donor. ****p < 0.0001; ***p < 0.001; **p < 0.01; ns, not significant versus anti-CD47 by one-way ANOVA, (n = 9), error bars ± SEM. (B) J774 mouse macrophage cells were pre-incubated with anti-CD16/anti-CD32 to block Fc receptors. Macrophages were co-incubated with CD20+CD47+GFP+ Raji cells and the indicated antibodies at 1 μg/ml. Anti-CD47 is B6H12-mIgG1. The percentage of GFP+ macrophages was determined by flow cytometry and was normalized to the maximal response in each of 2 independent experiments. ns, not significant; ****p < 0.0001, t test, (n = 6), error bars ± SEM.
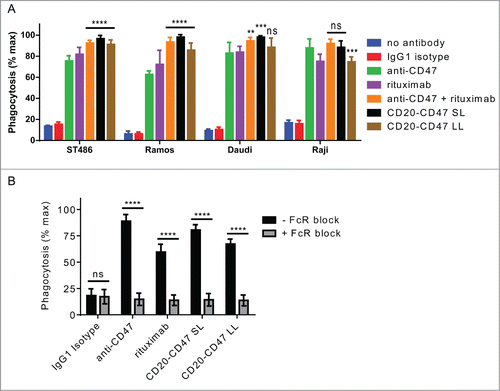
Figure 5. CD20-CD47 SL reduces lymphoma burden and extends survival in vivo: (A) NSG mice transplanted subcutaneously with Raji-luciferase cells were treated with daily injections of 200 μg mouse IgG control, anti-CD47 antibody, rituximab, or 200 μg anti-CD47 antibody + 200 μg rituximab. CD20-CD47 SL was administered at 1 mg to account for the increased molecular weight (n = 10 per treatment group). Luciferase imaging of representative mice from pre-treatment and after 14 doses are shown. (B) Bioluminescence values were normalized to pre-treatment values for each mouse. p values were derived by one-way ANOVA with Holm-Sidak's correction. *p < 0.05, **p < 0.01, ****p < 0.0001, line denotes mean. (C) Survival of engrafted mice. Arrows indicate the start (day 7) and stop (day 21) of treatment. Statistical analysis was performed by Mantel-Cox test. (D) Serum concentration pharmacokinetic profiles of each antibody throughout the course of treatment. Each line represents an individual mouse. Arrows indicate start (day 7) and stop (day 21) of treatment. Immobilized antibody directed against the human or mouse Fc region was used to capture the treatment antibodies from mouse serum prior to detection with HRP-conjugated antibody against the human or mouse kappa light chain of the treatment antibodies. Standard curves using known concentrations of antibodies were used to derive the serum levels. Antibody concentration is represented as μM of anti-CD20 F(ab’)2 (left) or as μM of anti-CD47 F(ab’)2 (right).
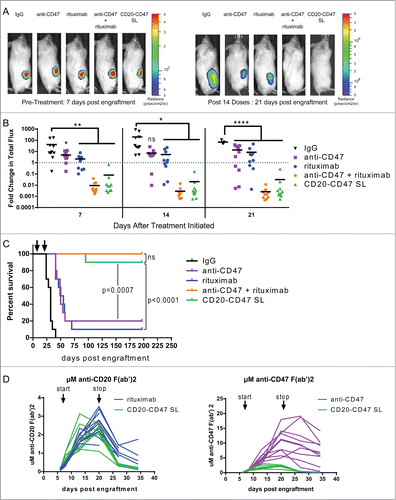