Figures & data
Figure 1. MFI analysis of insoluble aggregates. (A) Representative MFI images of particles (>5 µm) formed in the mixture of Avastin, 5% dextrose, and human plasma. (B) Particle counts in the sizes of 1–70 µm were determined using MFI for the resulting mixtures before and after centrifugation at 21,000 g for 3 minutes. Shown are representatives of 3 independent experiments.
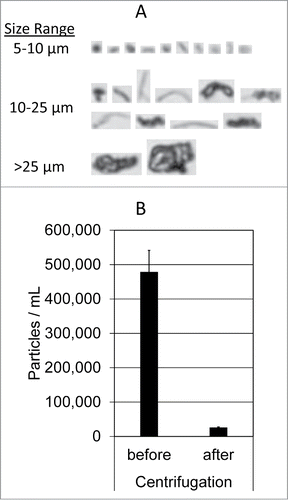
Figure 2. Characterization of insoluble protein aggregates formed when therapeutic mAbs are mixed with dextrose and human plasma. (A) SDS-PAGE analysis of individual protein samples (lanes 1–4), insoluble aggregate pellets collected using centrifugation (lanes 5–10), and basal protein adsorption (Ads) to the microtube surface (lane 11). Samples were prepared as described in Materials and Methods. Shown are representatives of 2 independent experiments. (B) The most abundant proteins that are identified by in-gel digestion and LC-MS/MS from samples in the corresponding bands of the SDS-PAGE gel. Note: pI values taken from the UniProtKB/Swiss-Prot database.
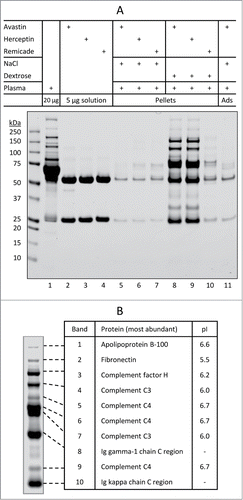
Table 1. Protein candidates identified in the insoluble aggregates formed in the mixture of dextrose-Avastin-plasma
Figure 3. Dextrose-mediated aggregation of Avastin in immunodepleted serum. (A) Dextrose-mediated aggregation of Avastin in modified human serum. Lanes 1–3, protein adsorption/aggregation in human plasma. Lanes 4–10, protein aggregation in complete human serum (Ctl) or immunodepleted human serum void of one of the following complement proteins: C1q, C2, C3, C4, factor B (FctB), or factor D (FctD). (B) Dextrose-mediated aggregation of Avastin in human plasma (Pla) or in fresh mouse serum from wild-type (WT) or C3 knocked out (C3KO) mouse.
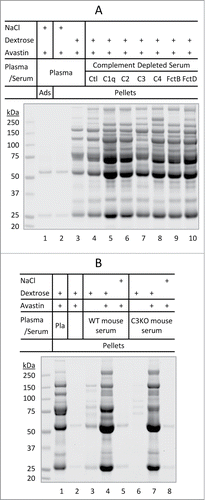
Figure 4. Formulation-dependent aggregation of therapeutic mAbs when encountering dextrose and human plasma. (A) Reversal of aggregation patterns of mAbs after switching formulation buffers. Buffer exchange was achieved using centrifugal filter units as described in Materials and Methods. R/Af and A/Rf: Remicade was placed in Avastin's formulation buffer or vice versa. (B) Effects of altering formulation pH. Lanes 3 and 5: aliquot of phosphate buffer (0.2 M, pH 8.0 or 5.9) 7.5 µL was added to 0.3 mL of dextrose prior to mixing with 10 µL of Avastin or Remicade and then 10 µL of plasma, respectively, resulting in the pH values as indicated. (C) Effects of alterations to formulation excipients. A solution of 0.3 mL dextrose was mixed with 10 µL of either Avastin (lane 1 and 3), Avastin formulation (lane 4, no API), or artificial formulation buffers lacking the specific excipients (lane 5–7). After mixing with 10 µL of human plasma (lane 2–7), resulting in the final pH as indicated, the mixtures were incubated for 10 min at 25°C, pellets were collected and resolved on SDS-PAGE.
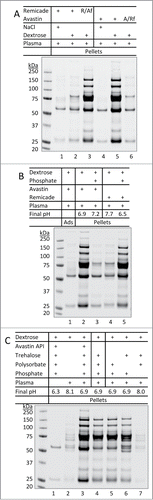
Table 2. Comparison of the approved product formulations
Figure 5. Aggregation of plasma proteins as a prerequisite of Avastin aggregation in the presence of dextrose. (A) pH-dependent aggregation of plasma proteins. Aliquots of dextrose (0.3 mL) were mixed with either 10 µL of Avastin formulation (no mAb API) or 7.5 µL of phosphate buffer (0.2 M) with different starting pH (5.9 to 8.0). After mixing with 10 µL of plasma, the mixture at the indicated final pH was incubated for 10 min at 25°C, the pellets were collected and analyzed. (B) Removal of pre-formed aggregates of plasma proteins prevented dextrose mediated aggregation of Avastin. Aggregates formed in the mixture of Avastin formulation (no mAb API) and dextrose-plasma solution were removed by either centrifugation (Cen) or filtration (Flt) through 0.22 µm filter. Avastin was then added and the pellets were analyzed.
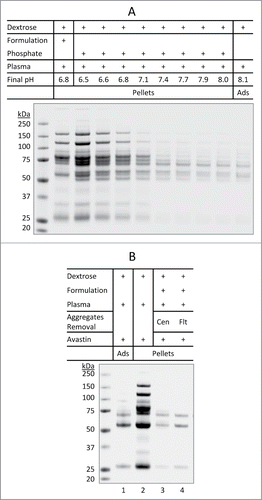
Figure 6. Inability of Pulmozyme to co-precipitate with aggregates of plasma proteins. Pulmozyme was exchanged into dextrose-phosphate solution prior to mixing with plasma (lane 3). Briefly, Pulmozyme (250 µL) was added to 3 mL of 5% dextrose containing 75 µL of 0.2 M phosphate buffer pH 5.9. The solution was concentrated to 0.3 mL at 5°C using an Amicon ultra-4 membrane unit (3 kDa molecular weight cutoff). The membrane integrity was confirmed by SDS-PAGE showing a complete recovery of total proteins and that no protein was detected in the filtrate. A solution of 10 µL plasma was then added to the Pulmozyme-dextrose-phosphate mixture, followed by 30 min incubation at 25°C. The pellets were collected and analyzed by SDS-PAGE. Lane 1: Pulmozyme solution (2 µg) alone. Lane 2: plasma protein aggregates formed in dextrose-phosphate-plasma mixture. Arrow indicates potential location of Pulmozyme protein band (˜37 kDa), if any, presented in the pellets.
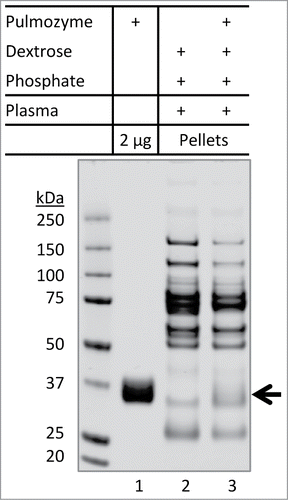
Figure 7. Schematic view of possible biochemical pathways facilitating dextrose-mediated aggregation of therapeutic monoclonal antibodies in human plasma. Dextrose appears to induce isoelectric precipitation of abundant plasma proteins, namely complement protein C3/C4, complement factor H, fibronectin, and apolipoprotein B-100, whose pI values are proximate to the pH of product formulation (in the range of 6.0 to 6.2). Subsequently, the concurrent mAb becomes co-precipitated, possibly through interactions with complement proteins,Citation18 resulting in insoluble protein aggregates containing both mAb and plasma proteins.
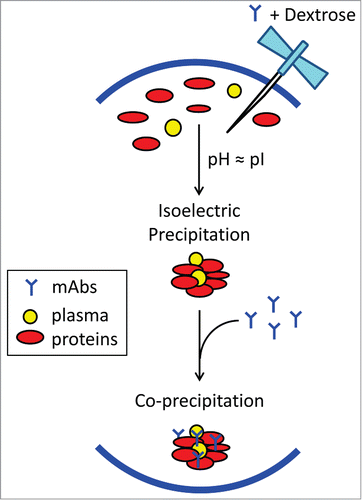