Figures & data
Figure 1. Kinetics of TNF expression in human monocytes and macrophages post LPS treatment. Human CD14+ monocytes (A) or day 7 human CD14+ monocyte-derived macrophages (B) were either untreated or treated with LPS in the presence or absence of TACE inhibitor, TAPI-2, for the indicated periods of time. Cell surface proteins were labeled with cell-impermeable Sulfo-NHS-SS-biotin. Biotinylated surface proteins were precipitated with streptavidin-conjugated agarose beads. Cell surface biotinylated and total proteins were subjected to immunoblotting using anti-TNF IgG. Na, K-ATPase and GAPDH protein expressions were used as total protein loading and cell surface biotinylation controls. Cell-free culture supernatants from monocytes (C) and macrophages (D) as treated in (A) and (B), respectively, were assayed for the presence of soluble TNF (sTNF) by ELISA.
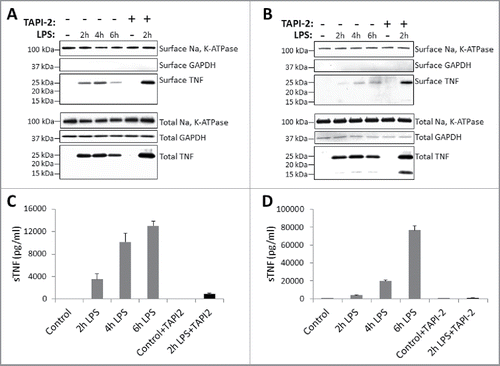
Figure 2. Kinetics of TNF expression in monocyte-derived dendritic cells post LPS treatment. Day 5 human CD14+ monocyte-derived DCs were either untreated or treated with LPS in the absence (A) or presence (B) of 20 μM TACE inhibitor, TAPI-2, for the indicated periods of time. Cell surface proteins were labeled with cell-impermeable Sulfo-NHS-SS-biotin. Biotinylated surface proteins were precipitated with streptavidin-conjugated agarose beads. Cell surface biotinylated and total proteins were subjected to immunoblotting using anti-TNF IgG. Cadherins and GAPDH protein expressions were used as total protein and cell surface biotinylation loading controls. Cell-free culture supernatants of DCs as treated in (A) and (B) above were assayed for the presence of soluble TNF (sTNF) (C) by ELISA.
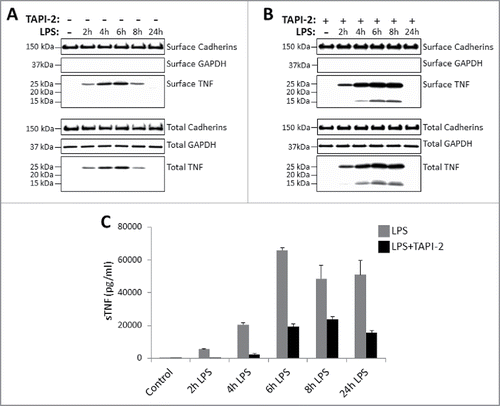
Figure 3. TmTNF-dependent endocytosis of anti-TNFs in dendritic cells. (A) Day 5 human CD14+ monocyte-derived DCs were treated with LPS for 2 h, incubated with pHrodo-conjugated anti-TNF and cellular uptake of labeled anti-TNF was monitored. Plasma membrane was stained using anti-MHC class I IgG (green), the nucleus (blue), and the internalized pHrodo-anti-TNF (red) were visualized using Amnis imaging flow cytometry. (B) Day 5 human DCs were treated with LPS for 2 h and cells were incubated either in the presence of pHrodo-conjugated matched isotype control IgG (red dotted histogram) or pHrodo-conjugated anti-TNF (blue filled histogram). Cellular uptake of the labeled antibodies was monitored for up to 4 h using flow cytometry. (C) To measure the kinetics of endocytosis of anti-TNF into acidic compartments in DCs, cells were either untreated (No LPS) or treated with LPS for 2 h. Cells were incubated with either human IgG1-pHrodo or anti-TNF-pHrodo antibodies. Endocytosis of the internalized anti-TNF was measured as an increase in fluorescence intensity by flow cytometry for up to 4 h.
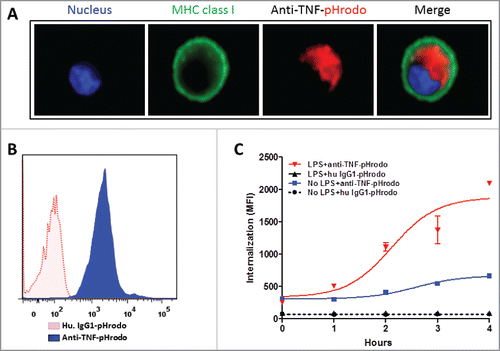
Figure 4. Clathrin-dependent endocytosis of anti-TNFs in dendritic cells. (A) Identification of anti-TNF/TmTNF-associated proteins: Day 5 human CD14+ monocyte-derived DCs were treated with LPS for 2 h and incubated either with isotype matched human IgG1 or with anti-TNF for 5 min at 37°C. Peptide sequences corresponding to TNF or clathrin heavy chain from anti-TNF immunoprecipitated proteins were identified by LC-MS/MS. (B) Identification of anti-TNF/TmTNF-associated clathrin heavy chain: Immunoprecipitated proteins and total cell extracts from DCs as treated in (A) above were subjected to immunoblotting using anti-clathrin heavy chain or anti-TNF antibodies. IP: Immunoprecipitation. (C) Effect of clathrin inhibitor on endocytosis of anti-TNF: Day 5 human CD14+ monocyte-derived DCs were treated with LPS for 2 h either in the absence (Medium) or presence of a 5 μM clathrin inhibitor, Dyngo 4a. Endocytosis of the internalized anti-TNF was measured as an increase in fluorescence intensity by flow cytometry for up to 4 h.
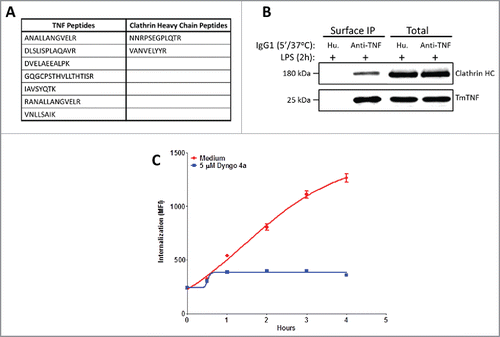
Figure 5. TmTNF-dependent vesicular trafficking of humanized anti-TNFs and detection of cell surface anti-TNF peptides in dendritic cells. (A) Compartmentalization of anti-TNF: Day 5 human CD14+ monocyte-derived DCs were treated with LPS for 2 h and the cells ‘pulsed’ on ice using Alexa 488-conjugated anti-TNF (0 min, ice). The fluorescent label on the anti-TNF was ‘chased’ for up to 1 h at 37°C. Endosomes and lysosomes were stained with Alexa 647-conjuated anti-EEA1 and anti-LAMP1, respectively. Nucleus was stained with DAPI. Images were acquired using confocal microscopy. (B) Identification of cell surface-associated anti-TNF peptides: Day 5 human CD14+ monocyte-derived DCs were treated with LPS for 2 h and incubated with either an anti-TNF mAb or a DVD-Ig containing one anti-TNF domain for 6 h. Cell surface displayed peptides were eluted under mild acidic conditions and the identity of anti-TNF peptides was obtained using LC-MS/MS. (C) Identification of HLA-DR-associated anti-TNF peptides: Day 5 human CD14+ monocyte-derived DCs were treated with LPS for 2 h and incubated with anti-TNF for up to 8 h. HLA-DR was immunoprecipitated and the identity of HLA-DR-associated anti-TNF peptides was obtained using LC-MS/MS (underlined: linker peptide sequence of anti-TNF DVD-Ig).
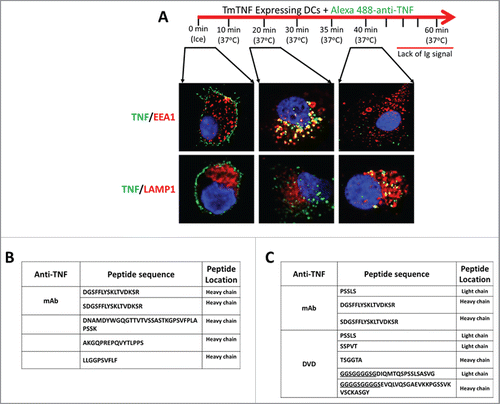
Table 1. Use of various anti-TNF antibodies in the current study.
Figure 6. TmTNF-dependent uptake of humanized anti-TNF mAb and generation of a memory T cell recall response by dendritic cells. (A) Scheme of generating anti-TNF-TT fusion IgGs: Sequence of tetanus toxin (TT) peptides (S1 or S2) were fused to the C-terminus of the heavy chains of an anti-TNF to obtain anti-TNF-S1 or anti-TNF-S2 antibodies. (B) Testing the potency of anti-TNF-TT fusion IgGs: The potency of the parental anti-TNF and anti-TT-fusion IgGs (anti-TNF-S1 and anti-TNF-S2) to neutralize soluble TNF were tested using the L929 assay. (C) Measuring T cell recall response in DCs: Day 5 human CD14+ monocyte-derived DCs were treated with LPS for 2 h and incubated either with tetanus toxin (TT antigen), anti-TNF-S1 or anti-TNF-S2. DCs were co-cultured with CFSE-labeled autologous T cells, and T cell proliferation assessed using flow cytometry.
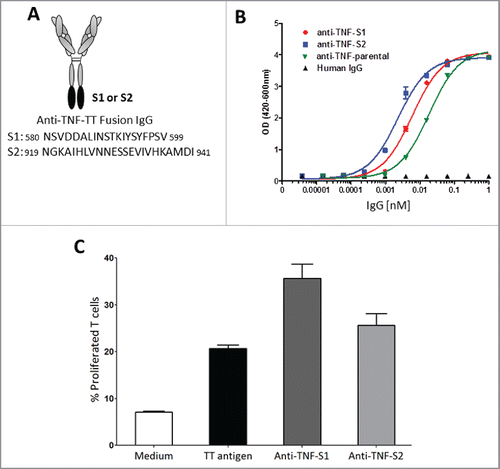
Figure 7. Schematic overview of the fate of TmTNF-bound anti-TNF. Binding of anti-TNF to cell surface expressed TmTNF (1) results in transducing intracellular ‘Reverse’ signal (2) and formation of a clathrin-coated pit (3) resulting in endocytosis of the complex into the endosomes (4) followed by delivery of the complex to the phagolysosome compartment (5) where proteolytically processed anti-TNF peptides are loaded onto HLA-DR and subsequently displayed as HLA-DR-associated peptides on the cell surface (6).
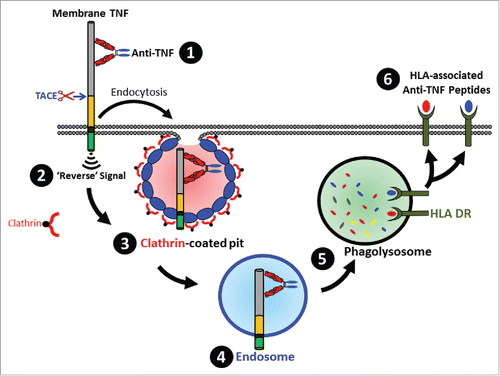