Figures & data
Figure 1. Steric and electrostatic in silico design strategies to drive IgA Fc heterodimer formation and OAA format used to test designs in vitro. (a) Cartoon depictions of steric and electrostatic negative and positive design concepts for in silico modeling of mutations to drive heterodimerization of an IgA Fc (left). Examples of structural models of steric and electrostatic designs in an IgA CH3 predicted to favor heterodimer formation of the Fc (right). Cartoon depiction of concepts are overlayed on the structural models for clarity. (b) Example of in silico knowledge-based and physics-based metrics used to rank favorable steric and electrostatic designs (single design shown). (c) Antibody formats used to test heterodimeric designs in vitro. Mutations favoring formation of a heterodimeric IgA Fc assemble into a OAA species that can be resolved by size from homodimeric Fc-only and full-sized antibody species. The OAA used is composed of anti-Her2 IgG1 Fab, IgG1/IgA2 hybrid hinge and IgA2m1 Fc.
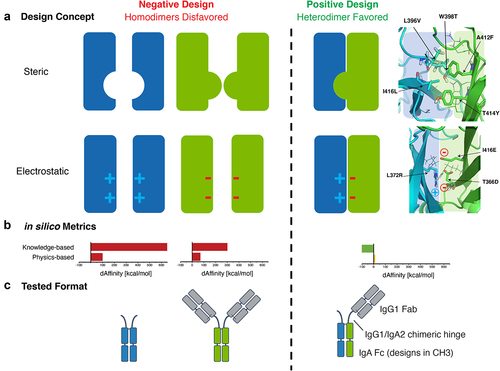
Table 1. Summary of purification results and CH3 thermal stability of heterodimeric IgA Fc steric designs.
Figure 2. Lead steric designs 6, 10, and 11 drive formation of a heterodimeric IgA Fc as determined by formation of OAA species in vitro and possess wild-type IgA-like thermal stability. (a) Non-reducing CE-SDS profiles of IgA Fc OAA variants after affinity purification. Data are shown for variants comprising a wild-type IgA CH3 and those containing heterodimer-driving mutations from steric designs 1–4 and 6–11. (b) Analysis of IgA OAA variants by analytical SEC after affinity purification. Data is shown for a wild-type IgA Fc (left) as well as steric design 6 (right). (c) Overlay of DSC thermograms measured for SEC-purified wild-type IgA Fc (black) and heterodimeric IgA Fc containing mutations from steric designs 2 (red), 3 (orange), 6 (yellow), 10 (light green), and 11 (green) OAA variants. The first and second transitions are attributed to CH3 and CH2 + Fab unfolding, respectively.
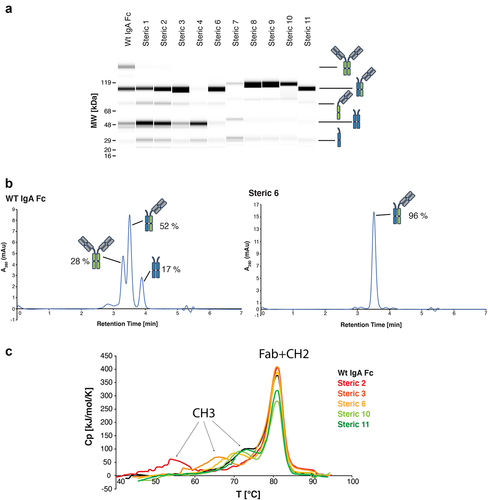
Figure 3. The crystal structure of heterodimeric IgA Fc steric design 6 was solved in complex with S. aureus SSL7 (PDB ID: 7TTZ). The heterodimeric IgA Fc has high structural homology with the predicted in silico model and differs from wild-type IgA Fc only at the CH3:CH3 interface. (a) Cartoon representation of the heterodimeric IgA Fc (steric 6) in pale cyan and pale green in complex with two S. aureus SSL7 monomers in pale orange and pale yellow (PDB ID: 7TTZ). (b) Superimposition of the heterodimeric IgA Fc (steric 6) crystal structure (pale blue and pale green) and wild-type IgA Fc (PDB ID: 2QEJ; gray) illustrates the structural homology between heterodimeric and homodimeric IgA CH2 and CH3 domain structure. The peptide backbone is drawn as ribbon and mutated residues and equivalent wild-type residues in the CH3:CH3 interface are drawn as sticks. (c) Cartoon representation of heterodimeric IgA (steric 6) CH3:CH3 interface with mutated residues drawn as sticks (right) compared to wild-type homodimeric IgA Fc (PDB ID: 2QEJ) interface (left) and the predicted in silico model (middle).
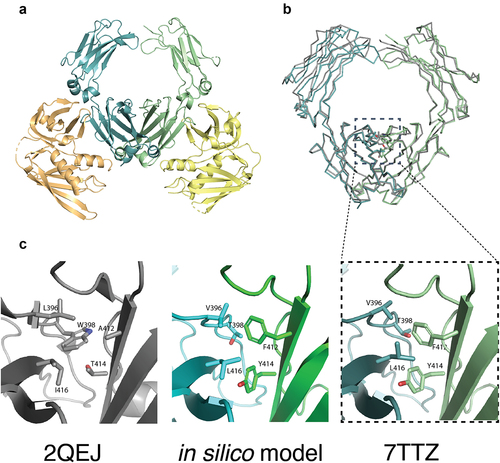
Figure 4. IgA heterodimeric Fc variants retain binding to the FcαRI. (a) Surface plasmon resonance sensorgrams for wild-type IgA OAA (WT) (top, apparent KD = 11 nM), IgA OAA FcαRI-KO 1x (middle, KD = 273 nM), and heterodimeric IgA Fc (steric 6) OAA (bottom, apparent KD = 23 nM) measured at FcαRI ligand density of 14 RU, fit using a 1:1 Langmuir model. (b) Dependence of apparent KD of binding of IgA OAA variants to FcαRI on ligand density of FcαRI immobilized on the SPR sensor chip. Data for a wild-type IgA OAA, steric heterodimeric designs 6, 10, 11 as well as a single sided FcαRI-binding-deficient IgA Fc based on heterodimeric design 6 (IgA OAA FcαRI-KO 1x) is shown.
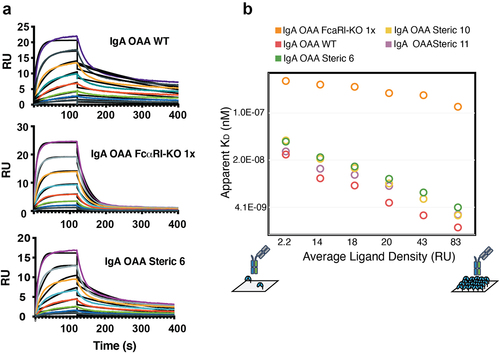
Figure 5. Applications of a heterodimeric IgA Fc in the development of IgA multispecifics and interrogation of IgA biology. The heterodimeric IgA Fc designs described herein (center) can be used for a variety of applications. These include engineering of an asymmetric IgA Fc, for example to introduce a FcRn-binding motif to increase the half-life of IgA while preserving binding to FcαRI (top left). A heterodimeric IgA can also be used to create multispecific therapeutics with a variety of formats, ranging from OAAs, bispecifics and biparatopics to multispecific multimers (top right) which can be used to target the mucosa when bound to the secretory compartment (bottom left). Heterodimeric IgAs can furthermore be used as a tool to interrogate fundamental questions surrounding IgA biology such as the effect of FcαRI binding valency on the activation of effector cells such as neutrophils (bottom right).
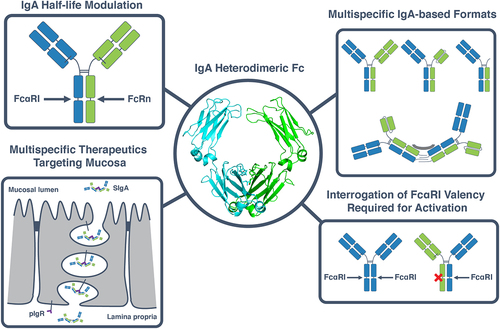