Figures & data
Figure 1. Operon integrity, fragmentation and diversification profiling and classification in Bacteroidota based on the number/presence of classical rfb genes ABCD and non-rfb genes. a) lipopolysaccharide (LPS) and O-antigen schematics. b) classical contiguity of rfb genes ABCD in the reference genome of E. coli K12 (rfbXABCD; XCADB; [rfb:5]). Horizontal lines represent the bacterial genome length, distribution of rfb genes/operons (shaded circles; darker = more genes) and rfb gene orientation (+, sense; –, antisense). nL: n of rfb clusters or gene singlet loci. Note orientations/duplications. c) classification of Bacteroidota based on rfb operon integrity. Six categories of operon arrangement & ‘rfb-gene-cluster’ fragmentation are depicted to summarize the observations of this study. For the purpose of operon classification, based on continuity vs. fragmentation, and diversification, the analysis summary is focused on the presence/absence of ‘expected contiguous rfb genes ABCD’ irrespective of other genetic elements required in any operon (e.g. transcribers, promoters, enhancers). Prediction analysis (see methods) indicates that such fragmented yet contiguous rfb-gene-clusters (minioperons) in Bacteroidota, including additional (non rfb) genes, have resulted in a diversified set of operons’ that are computationally-predictable functional transcriptional units involving the additional non-rfb gene. The location of non-rfb gene in the cluster of ‘diversified operons’ could be represented with an exclamation mark (!) for the locations of non-rfb gene in the cluster; wherein Alistipes finegoldii DSM17242 has non-rfb(!) genes within its operons rfb(BDCA!) indicates (B!1D!2C!3A)(----), and where (!Citation1=glycosyltransferase, !Citation2=acyltransferase and !Citation3= acetyltransferase). Supplementary Figure S1c illustrates in context rfb operon fragmentation and diversification for Alistipes, Bacteroides, Parabacteroides, Prevotella, Paraprevotella, Barnesiella, Tannerella, Odoribacter and Porphyromonas.
![Figure 1. Operon integrity, fragmentation and diversification profiling and classification in Bacteroidota based on the number/presence of classical rfb genes ABCD and non-rfb genes. a) lipopolysaccharide (LPS) and O-antigen schematics. b) classical contiguity of rfb genes ABCD in the reference genome of E. coli K12 (rfbXABCD; XCADB; [rfb:5]). Horizontal lines represent the bacterial genome length, distribution of rfb genes/operons (shaded circles; darker = more genes) and rfb gene orientation (+, sense; –, antisense). nL: n of rfb clusters or gene singlet loci. Note orientations/duplications. c) classification of Bacteroidota based on rfb operon integrity. Six categories of operon arrangement & ‘rfb-gene-cluster’ fragmentation are depicted to summarize the observations of this study. For the purpose of operon classification, based on continuity vs. fragmentation, and diversification, the analysis summary is focused on the presence/absence of ‘expected contiguous rfb genes ABCD’ irrespective of other genetic elements required in any operon (e.g. transcribers, promoters, enhancers). Prediction analysis (see methods) indicates that such fragmented yet contiguous rfb-gene-clusters (minioperons) in Bacteroidota, including additional (non rfb) genes, have resulted in a diversified set of operons’ that are computationally-predictable functional transcriptional units involving the additional non-rfb gene. The location of non-rfb gene in the cluster of ‘diversified operons’ could be represented with an exclamation mark (!) for the locations of non-rfb gene in the cluster; wherein Alistipes finegoldii DSM17242 has non-rfb(!) genes within its operons rfb(BDCA!) indicates (B!1D!2C!3A)(----), and where (!Citation1=glycosyltransferase, !Citation2=acyltransferase and !Citation3= acetyltransferase). Supplementary Figure S1c illustrates in context rfb operon fragmentation and diversification for Alistipes, Bacteroides, Parabacteroides, Prevotella, Paraprevotella, Barnesiella, Tannerella, Odoribacter and Porphyromonas.](/cms/asset/c00d1d87-da77-4ed1-b737-446c289fd80a/kgmi_a_2350150_f0001_oc.jpg)
Figure 2. The occurrence, patterns and inversions of rfb-gene-clusters in P. distasonis and B. thetaiotaomicron suggests mechanism of operon fragmentation in Bacteroidota. a) fragmentation of rfb operon in P. distasonis is in modern times supra-numerary compared to ATCC8503 strain from USA/1933. b) fragmentation has resulted in conserved singlet, doublet/triplet patterns in P. distasonis. c) heatmap clustering of P. distasonis strains based on rfb-gene-cluster shows distinct clades. Additional information is available in supplementary figure S2b. d) unique rfbFGC->rfbA rfb-gene-cluster distancing pattern (downward red/black arrows and solid circles) in B. thetaiotaomicron is also present in novel CavFT strains of P. distasonis from gut wall lesions in Crohn’s disease. Notice the orientation sense and patterns. e) SDS-PAGE of LPS extract analysis of E. coli and P. distasonis CavFT-46 shows that P. distasonis is unable to produce O-antigen polysaccharides in diverse growth conditions (Fisher’s exact p=0.079), the observed higher molecular weight band (approximately 130Kb) in P. distasonis suggests saccharide is of capsular origin, rather than lipopolysaccharide (see manuscript).
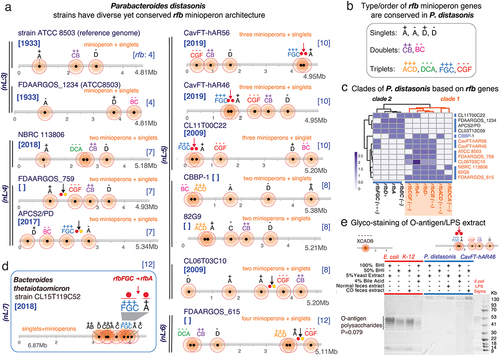
Figure 3. The rfb operon in Alistipes is contiguous and duplicated suggesting evolutionary benefit. a) Alistipes has contiguous rfb operons with frequent duplication and less common incorporation of rfb-gene-cluster duplets. b) patterns of conserved rfb-gene-clusters in Alistipes differ from Parabacteroides. c) schematics of gene-gene rfb distances measured within and between rfb-gene-clusters. d) Parabacteroides and Bacteroides demonstrate the greatest variance in number of rfb operon fragments and gene-gene distances. Alistipes and enterobacteriaceae are similarly contiguous. Intergene distances for Parabacteroides, Bacteroides and Prevotella were greater than enterobacteriaceae (0.54 ± 0.84Mb, 0.49 ± 0.86Mb, 0.34 ± 0.32Mb, respectively, vs. 0.13 ± 0.46Mb, p < .001). Alistipes gene distribution is similar to enterobacteriaceae (0.17 ± 0.49Mb, p = .79). e) rfb-gene-cluster sequence homologies for Alistipes vs. Parabacteroides based on rfb-gene-cluster orientation between and within genera/species. Two tailed-T tests p < .01 **, p < .001 ***. f) alignment and g) phylogeny based on rfb operon sequences. Note that Alistipes clusters are driven by the sense/antisense orientation of the operons.
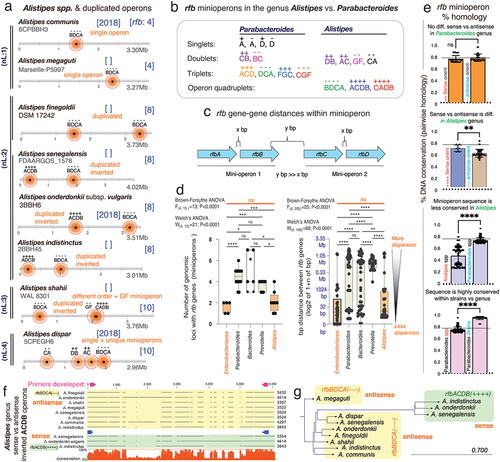
Figure 4. ‘Rfb-operon profiling’ indicates non-random selection and rfb-gene-cluster similarity between Parabacteroides and Bacteroides, namely B. thetaiotaomicron. a) gaps and insertions in rfb gene sequences designate different rfb-types using protocols described for rfbA-typing.Citation3 supplementary figure S5 illustrates the rfb-typing of rfbC/D/F/G. b) exa mple of global rfb operon profiling system (GOPS) for P. distasonis. b) density plots between random and real rfb operon profiles in P. distasonis. Observed types are statistically different from a random (uniform) distribution (**, *** for p<.05 and p<.01, respectively). We generated random and real operon profiles based on the rfb(A,B,C,D,E,F, and G) gene types. For example, P. distasonis ATCC 8503 has rfbA, rfbB, rfbC, and rfbD genes of type I, with the real operon profile represented as 111,100 (indicating types I for rfbA, rfbB, rfbC, and rfbD, and absence of rfbF and rfbG). Each gene type has varying subtypes (e.g., rfbA has 4 types, rfbB has 2 types, rfbC has 6 types, etc. ()). We then created random profiles by permuting combinations of these types, resulting in profiles such as 111,100, 211100, 311100, 411100, and so on. Using these numeric profile we create a density plot using STATA software. d) phylogenetics across Bacteroidota and enterobacteriaceae based on rfb mini/operon sequences. Remarkably, several Bacteroides species, but namely B. thetaiotaomicron CLT5T119C52, cluster together with several Parabacteroides, especially P. distasonis, irrespective of rfb-gene-cluster considered (red squares; further details in Supplementary Figures S6).
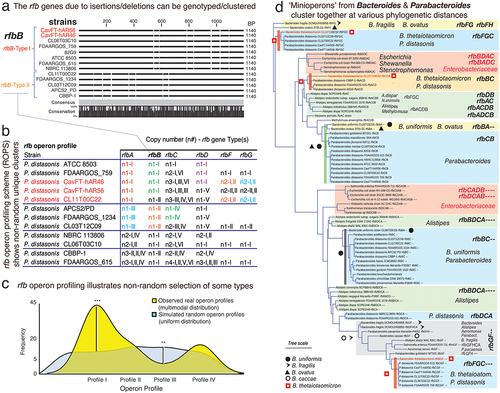
Figure 5. Fragmentation of nonessential operons is driven by insertions of ‘foreign DNA’, mainly Bacteroides. a) Schematics of O-antigen (rfb), fimbriae (fim) and ribosomal (rrn) gene operons tested for DNA insertions. b) example of P. distasonis inter-rfb-gene-cluster DNA fragment found in other genomes. c) % of DNA from one species common to the genome of 4–5 other species within the genus. d) Schematics showing source of ‘foreign DNA’ insertions into the rfbFGC->rfbA space separating the rfb genes in P. distasonis. e) ‘pure’ P. distasonis DNA fragments intermixed within inter-rfb-gene-cluster ‘foreign DNA’ insertions. f) genus sources and % of ‘foreign DNA’ fragmenting the rfb and fim rfb-gene-clusters in P. distasonis, as in . Bacteroides (B. thetaiotaomicron, B. fragilis, B. ovatus, B. vulgatus) are the main source of ‘foreign DNA bombardment’ (p<.0001 vs. Alistipes and Escherichia). Additional information is available in supplementary table S3. g) ribosomal operons (rrn, deemed essential) are not fragmented in P. distasonis, despite presence of ‘foreign DNA’ in vicinity. *, **, ***, for p<.01, p<.001, p<.0001, respectively. Supplementary table S4 shows rrn operons are not fragmented in other genera, i.e., Prevotella, Bacteroides, Alistipes, and Porphyromonas.
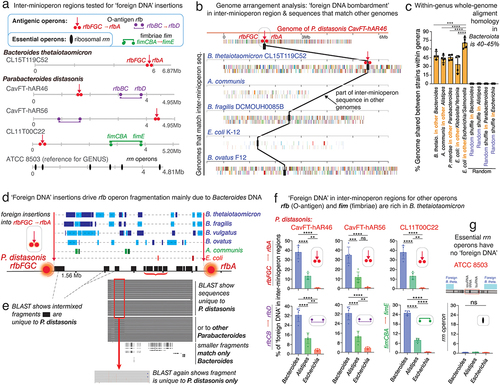
Figure 6. Impact of ‘foreign DNA’ insertions in Bacteroidota on metagenomics, antigenic operons and bacteria-bacteria inflammatory interactions in a ‘Bacteroidota and Enterobacteriaceae model of gut microlesions’. a) plot of DNA fragments that align between parabacteroides CL11T00C22 and Bacteroides, Alistipes, Escherichia genomes (n=16) ordered by length of each aligning fragment. Notice genus-genus differences. B. thetaiotaomicron CL15T119C52 has unique pattern of abundant fragments (maximum fragment sizes and average % of genome shared, inset bar plots). b) BlastX (protein) and metagenomic (nucleotide) taxonomic analyses of 250bp-fragmented P. distasonis genome. Notice ‘extra species’ assigned by metagenomics (inflation), reflecting ‘foreign DNA’ insertions/exchange across Bacteroidota, and not real presence of species (inset bar plot). The n of ‘extra species’ varied with fragment length (Pearson corr. 0.84, p<.05). The performance of BLAST and BLASTX depends on bacterial genome (supplementary figure S9). c) metagenomic community simulation with Bacteroides, Parabacteroides, Alistipes, and Escherichia (1:1:1:1 genomes). Krona plot (relative abundances within hierarchies of metagenomic classificationsCitation47 illustrates E. coli sequences are poorly assigned to E. coli leading to relative ratio overestimation of Bacteroidota abundance (1:17:12:18; see krona plots for individual genomes (‘alone’) in supplementary figure S10). Bacteroides is commonly listed as ‘extra species’ (bar plot; complete list in supplementary figure S8). d) KEGG pathway and total gene counts in Enterobacteriaceae and Bacteroidota, highlighting the significant differences for Bacteroidota (details in supplementary table S7). e) Stereomicroscopic 3D-apperance of an intestinal cavernous fistulous tract (CavFT) micropathology in a Crohn’s disease patient. Reproduced for illustration from Rodriguez-Palacios, A. et al.Citation23 Diagram illustrates co-existence of Enterobacteriaceae (red) and Bacteroidota (blue) in microlesions, and a peritonitis experiment in which mice received P. distasonis/Bacteroidota or E. coli/Enterobacteriaceae. f) average pro-inflammatory cytokine secretion by bacteria derived from in the Raw murine macrophage stimulation assays, indicating that Bacteroidota whole-cell heat killed extract trigger the release of pro-inflammatory TNF-a cytokines to a lower extent compared to Enterobacteriaceae. g) hypothetical model of gut microlesions with colonization of commensal/pathobionts modulating inflammation. Peritonitis model showed mice with enterobacteriaceae had fatal peritonitis, but not if receiving Bacteroidota B. thetaiotaomicron, B. fragilis, or P. distasonis. *p<.01; ****p<.00001.
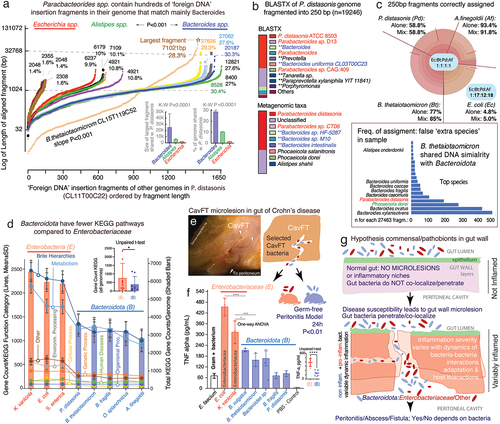