Figures & data
Table 1. Comparison of model scale and inflow velocity used for noise simulation in the literature.
Figure 1. Full model and sub-model of high-speed train: (a) full model, (b) sub-model and (c) bogie model.
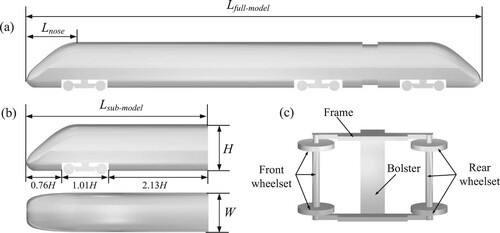
Table 2. Case descriptions.
Table 3. Comparison of far-field noise results under different grid densities.
Table 4. Solver settings for numerical simulation.
Figure 8. Comparison of noise spectra for far-field acoustic receivers: (a) top receiver and (b) side receiver.
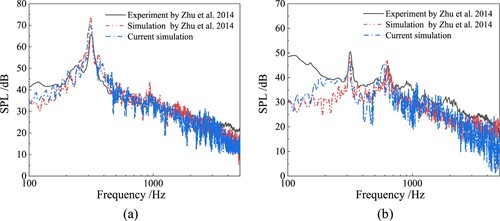
Figure 11. Spectrum characteristics of train surface pressure monitoring points: (a) Measurement points P1–P4 for case2, and (b) Measurement point P2 for different cases.
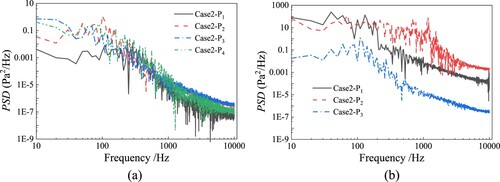
Figure 12. The instantaneous distribution of vortex structure in bogie region with different values, coloured by velocity: (a) case0,(b) case1 and (c) case2.
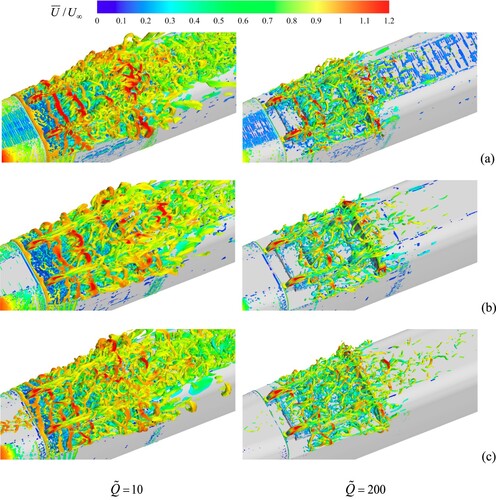
Figure 13. The instantaneous vorticity field distribution on the longitudinal central section (y = 0) and the vertical section (z = 0.18H): (a) case0, (b) case1 and (c) case2.
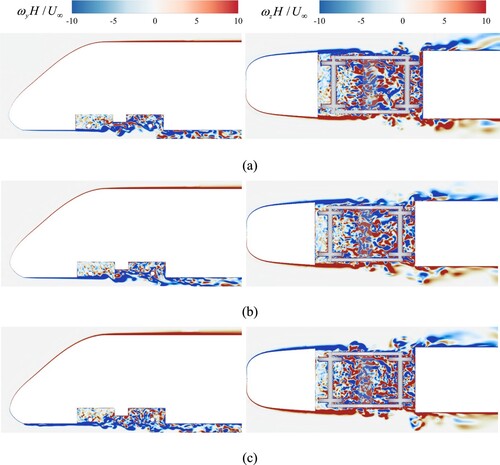
Figure 14. Comparison of flow field at the bottom of train: (a) dimensionless time-averaged velocity and (b) time-averaged vorticity magnitude.
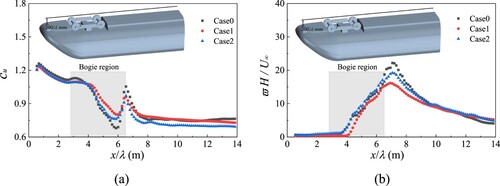
Figure 15. The instantaneous vortex core distribution in the bogie region: (a) case0, (b) case1 and (c) case2.
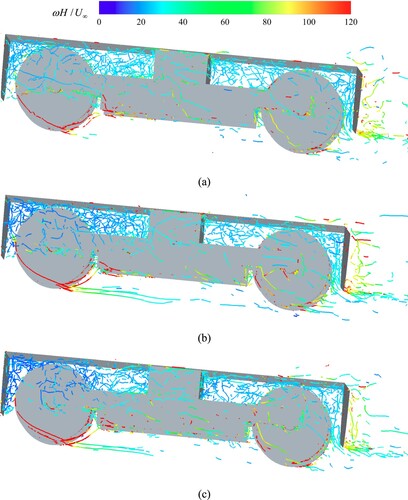
Figure 16. Surface pressure fluctuation distribution in the bogie region: (a) case0, (b) case1 and (c) case2.
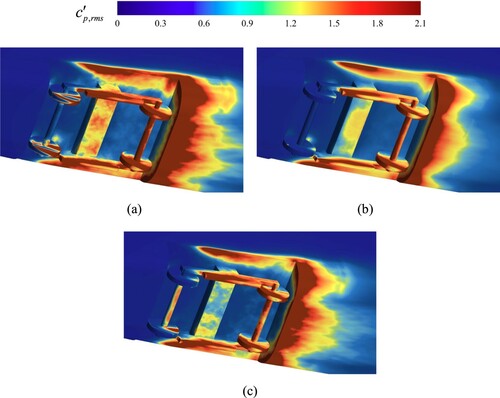
Figure 17. Mean velocity distribution near the wall of the bogie region: (a) case0, (b) case1 and (c) case2.
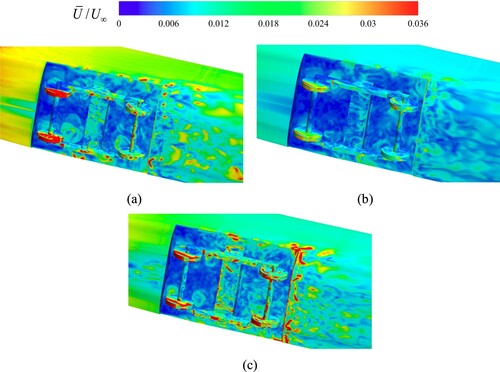
Figure 18. The instantaneous turbulent kinetic energy distribution around the train: (a) case0, (b) case1 and (c) case2.
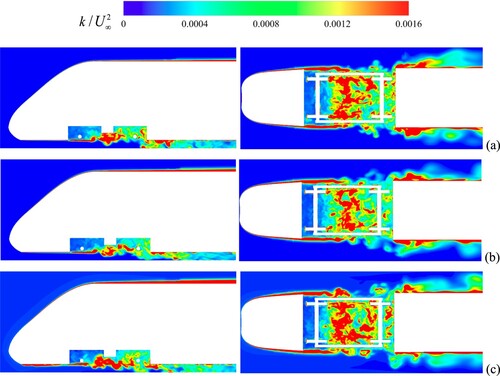
Figure 20. OASPL comparison of different components as noise sources: (a) case0, (b) case1 and (c) case2.
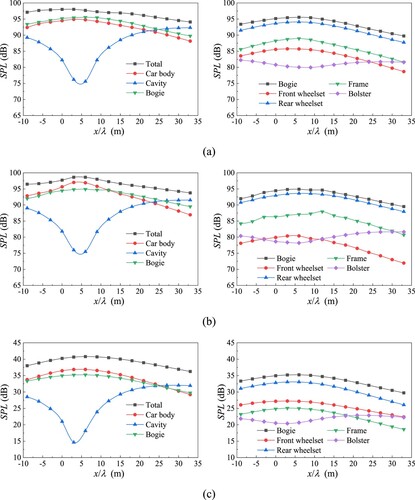
Figure 21. Comparison of OASPL results in different cases: (a) total, (b) car body, (c) cavity and (d) bogie.
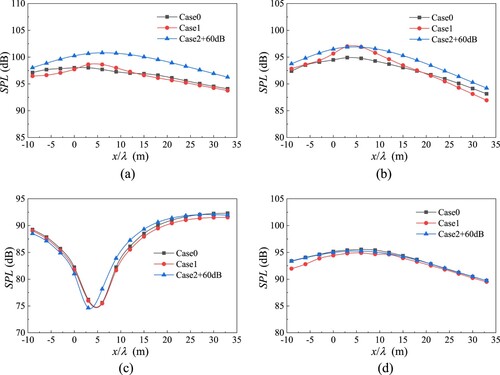
Figure 22. OASPL comparison for each component of the bogie: (a) front wheelset, (b) rear wheelset, (c) frame and (d) bolster.
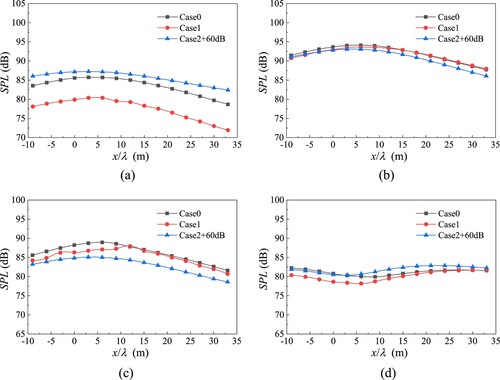
Figure 23. Comparison of noise spectra at receiver r6, with a frequency resolution of 10 Hz: (a) car body, (b) cavity, (c) bogie and (d) rear wheelset.
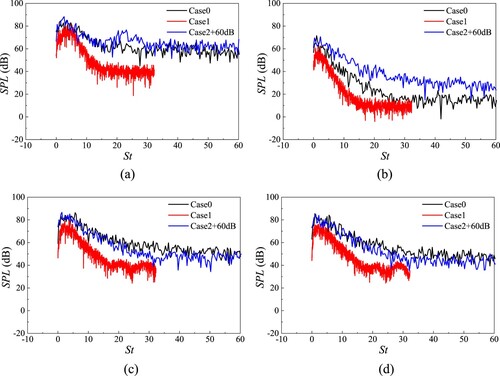
Data availability
The data that support the findings of this study are available from the corresponding author upon reasonable request.