Figures & data
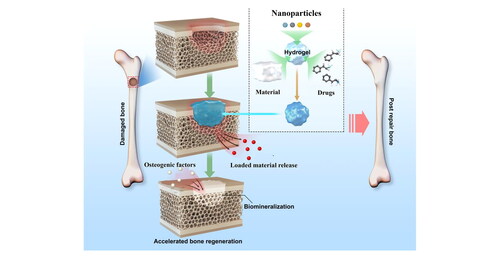
Figure 1. Chemical structures of nanoclay platelets currently used for various biomedical engineering applications. Nanoclays are basically ultrathin (≈1 nm thick) platelets with a dual charge that makes it easy to dissolve and incorporate into almost any existing hydrogel matrix. Reproduced with permission [Citation56]. Copyright, John Wiley and Sons.
![Figure 1. Chemical structures of nanoclay platelets currently used for various biomedical engineering applications. Nanoclays are basically ultrathin (≈1 nm thick) platelets with a dual charge that makes it easy to dissolve and incorporate into almost any existing hydrogel matrix. Reproduced with permission [Citation56]. Copyright, John Wiley and Sons.](/cms/asset/23962121-5660-4277-bd31-412ffd257650/ynan_a_2076025_f0001_c.jpg)
Figure 2. Schematic representation of the design strategy for the development of multifunctional nanocomposite hydrogels. DNA—nSi injectable hydrogels are formed viaa two-step gelation method. The first step consists of an intermediate weak gel (pregel) formation by heating and subsequent cooling of double-stranded DNA. The denaturation of doublestranded DNA followed by rehybridization in a random fashion facilitates the development of interconnections between adjacent DNA strands (type A network points) via complementary base pairing. Introduction of nSi in the second step of the gelation process increases the number of network points (type B) viaelectrostatic interaction with the DNA backbone, resulting in a shear-thinning injectable hydrogel. Reprinted with permission from Basu et al. (2018) [Citation70].
![Figure 2. Schematic representation of the design strategy for the development of multifunctional nanocomposite hydrogels. DNA—nSi injectable hydrogels are formed viaa two-step gelation method. The first step consists of an intermediate weak gel (pregel) formation by heating and subsequent cooling of double-stranded DNA. The denaturation of doublestranded DNA followed by rehybridization in a random fashion facilitates the development of interconnections between adjacent DNA strands (type A network points) via complementary base pairing. Introduction of nSi in the second step of the gelation process increases the number of network points (type B) viaelectrostatic interaction with the DNA backbone, resulting in a shear-thinning injectable hydrogel. Reprinted with permission from Basu et al. (2018) [Citation70].](/cms/asset/2ea02925-0f7e-40a7-a9af-04ef91265502/ynan_a_2076025_f0002_c.jpg)
Figure 3. Engineering of an osteon-like, cell-laden hydrogel as discussed in Section 2.1.2 a) Schematic representation of the formation of hydroxyapatite nanoparticles in GelMA. b) Schematic representation of the UV-microfabrication procedure employed in the generation of the osteon-like microstructures. c) Bright-field and fluorescence imaging of the resulting biomimetic osteon construct. c) Reprinted with permission from Shi et al. (2016) [Citation90] Copyright 2015, American Chemical Society.
![Figure 3. Engineering of an osteon-like, cell-laden hydrogel as discussed in Section 2.1.2 a) Schematic representation of the formation of hydroxyapatite nanoparticles in GelMA. b) Schematic representation of the UV-microfabrication procedure employed in the generation of the osteon-like microstructures. c) Bright-field and fluorescence imaging of the resulting biomimetic osteon construct. c) Reprinted with permission from Shi et al. (2016) [Citation90] Copyright 2015, American Chemical Society.](/cms/asset/e117a6a1-218d-4c9b-ad6c-069400fdd7a0/ynan_a_2076025_f0003_c.jpg)
Figure 4. Synthesis of MNP-reinforced nanocomposite hydrogels. (a) Nanocomposite hydrogels were synthesized by combining PEG-dopamine-MNPs with GelMA and exposing it to UV radiation in the presence of a photoinitiator to obtain covalently crosslinked network. (b) Crosslinking of the prepolymer solution was monitor using UV rheology. After the UV radiation was turned on, a significant increase in the modulus of the prepolymer solution was observed, indicating the formation of a crosslinked network. (c) The presence of MNPs within nanocomposite network was determined from XPS. (d) TEM images of nanocomposite show uniform distribution of MNPs within GelMA matrix. Inset show the electron diffraction pattern. (e) SEM micrographs of the nanocomposites indicate the formation of highly porous and interconnected networks. Reprinted with permission from Jaiswal et al. (2016) [Citation115].
![Figure 4. Synthesis of MNP-reinforced nanocomposite hydrogels. (a) Nanocomposite hydrogels were synthesized by combining PEG-dopamine-MNPs with GelMA and exposing it to UV radiation in the presence of a photoinitiator to obtain covalently crosslinked network. (b) Crosslinking of the prepolymer solution was monitor using UV rheology. After the UV radiation was turned on, a significant increase in the modulus of the prepolymer solution was observed, indicating the formation of a crosslinked network. (c) The presence of MNPs within nanocomposite network was determined from XPS. (d) TEM images of nanocomposite show uniform distribution of MNPs within GelMA matrix. Inset show the electron diffraction pattern. (e) SEM micrographs of the nanocomposites indicate the formation of highly porous and interconnected networks. Reprinted with permission from Jaiswal et al. (2016) [Citation115].](/cms/asset/128eada6-0905-40ff-9991-33c5fee54537/ynan_a_2076025_f0004_c.jpg)
Figure 5. Illustration of modulating cell − matrix interactions and mechanosensing of hMSCs by using a MNP-borne soft hydrogel substrate under a directional magneticfield. (A, B) Upward/downward magnetic attraction induces the EXPOSED/HIDDEN presentation of MNPs grafted with cell adhesive ligand on the hydrogel surface to trigger the activation/inactivation of cell mechanotransduction signaling, respectively. (C) Detailed chemical structures in a gray dashed square from (A). Reprinted with permission from Wong et al. (2020) [Citation116].
![Figure 5. Illustration of modulating cell − matrix interactions and mechanosensing of hMSCs by using a MNP-borne soft hydrogel substrate under a directional magneticfield. (A, B) Upward/downward magnetic attraction induces the EXPOSED/HIDDEN presentation of MNPs grafted with cell adhesive ligand on the hydrogel surface to trigger the activation/inactivation of cell mechanotransduction signaling, respectively. (C) Detailed chemical structures in a gray dashed square from (A). Reprinted with permission from Wong et al. (2020) [Citation116].](/cms/asset/508d0995-d08c-4e60-b823-d41e2e875146/ynan_a_2076025_f0005_c.jpg)
Figure 6. (a) Schematic description of gelatin methacrylate (GelMA) coated onto a carbon nanotube (CNT). (b) High resolution TEM image of CNT-GelMA. (c) Stress-strain curves of CNTGelMA hydrogels with various concentrations of CNTs. (d) Tensile moduli of CNT-GelMA hydrogels. (e) 3T3 fibroblasts cultured on GelMA hydrogel (A) and CNT-GelMA hydrogel (B).(Scale bar: 100 μm) Reprinted with permission from Shin et al. (2012) [Citation131]. Copyright 2011 American Chemical Society.
![Figure 6. (a) Schematic description of gelatin methacrylate (GelMA) coated onto a carbon nanotube (CNT). (b) High resolution TEM image of CNT-GelMA. (c) Stress-strain curves of CNTGelMA hydrogels with various concentrations of CNTs. (d) Tensile moduli of CNT-GelMA hydrogels. (e) 3T3 fibroblasts cultured on GelMA hydrogel (A) and CNT-GelMA hydrogel (B).(Scale bar: 100 μm) Reprinted with permission from Shin et al. (2012) [Citation131]. Copyright 2011 American Chemical Society.](/cms/asset/2d9c2ddd-fd82-4c1e-81e9-cd159b560d1c/ynan_a_2076025_f0006_c.jpg)
Figure 7. (A). SMH/GO hydrogels as an artificial bone substitute for bone regeneration in a rat calvarial defect model via regulating BMSCs migration and osteogenesis differentiation.
Characterizations of SMH/GO hydrogels. (B) Preparation of SMH/GO hydrogels via UV light at 365 nm. (C) FTIR spectra of SMH/GO hydrogels. (D) Mechanical properties of SMH/GO hydrogels. (E) Degradation profiles of SMH/GO hydrogels in PBS (pH7.4, 37 oC). (F) Scanning electron micrographs of SMH (left), SMH/GO-1 (middle) and SMH/GO-2 (right). Scale Bars, 500μm (upper panel) and 10μm (lower panel). Red arrowheads indicate graphene oxide. Reprinted with permission from Qi et al. (2020) [Citation144].
![Figure 7. (A). SMH/GO hydrogels as an artificial bone substitute for bone regeneration in a rat calvarial defect model via regulating BMSCs migration and osteogenesis differentiation.Characterizations of SMH/GO hydrogels. (B) Preparation of SMH/GO hydrogels via UV light at 365 nm. (C) FTIR spectra of SMH/GO hydrogels. (D) Mechanical properties of SMH/GO hydrogels. (E) Degradation profiles of SMH/GO hydrogels in PBS (pH7.4, 37 oC). (F) Scanning electron micrographs of SMH (left), SMH/GO-1 (middle) and SMH/GO-2 (right). Scale Bars, 500μm (upper panel) and 10μm (lower panel). Red arrowheads indicate graphene oxide. Reprinted with permission from Qi et al. (2020) [Citation144].](/cms/asset/20bb8ea5-7930-4626-96d5-c2fbe85430a3/ynan_a_2076025_f0007_c.jpg)
Table 1. The properties of nanocomposite hydrogels.