Figures & data
Figure 1. HU protein structure and phylogenetic relationship among Francisella species. Predicted homodimer structure of the FSC200 HU protein (FTS_0886) and highlighted amino acids that are supposed to be important for DNA binding capacity of the protein. Protein structure was predicted using HDOCK server and visualized by BIOVIA Discovery Studio Visualizer (a). FSC200 HU protein sequence with highlighted (red dots) amino acids important for DNA binding properties of the HU protein (b). Phylogram of the Francisella HU proteins generated using Clustal Omega (Simple Phylogeny web service) (c). Conservation of the HU protein in Francisella species (d).
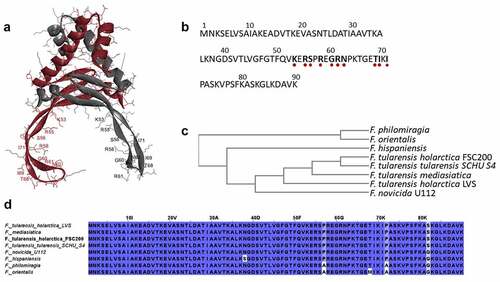
Table 1. Bacterial strains used in this study.
Table 2. Selected genes containing HU protein binding site under standard od stress growth conditions.
Figure 2. Identification of HU protein binding motifs. Two different HU protein binding motifs depending upon growth conditions ((a) standard growth conditions and (b) oxidative stress conditions) were found using the Multiple EM for Motif Elicitation (MEME-ChIP) tool (v5.0.2). Binding motifs were found in all target sequences identified using ChIP-seq.
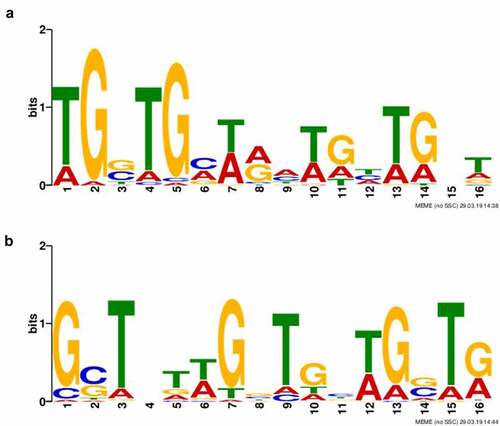
Figure 3. Visualization of HU–DNA complexes using AFM. F. tularensis FSC200/HU_HA was grown under standard (a) or oxidative stress (b) conditions. AFM imaging was performed in PeakForce Tapping mode. Samples purified from F. tularensis FSC200/ΔHU were used as negative controls. Larger quantities of DNA and HU–DNA complexes (HU protein corresponds to white dots) were identified in the late exponential phase. Imaging of samples of negative control confirmed the absence of HU protein, DNA, and their complexes, as well. The height profile scale is shown on the right.
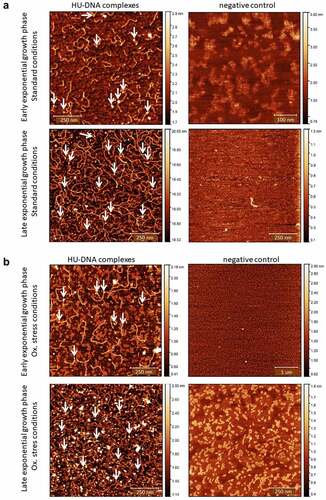
Figure 4. Identification of HU protein binding motifs within the sequences of the 477 bp upstream of the pigR gene and pigR gene. (a) in silico analysis of DNA sequence upstream of the pigR gene (in black) and within pigR gene (in blue) showed 8 possible binding sites (turquoise) of the HU protein for standard (6 of them upstream of the pigR gene and two within the gene) and one binding site (red) for oxidative stress conditions. (b). EMSA experiment confirmed binding of the HU protein to 477 bp DNA sequence upstream of the pigR gene. 100 ng of 477 bp DNA sequence upstream of the pigR gene was added to a various amounts of HU protein (0-800 ng) in a binding buffer and incubated for 20 min on ice. Then, the samples were resolved on DNA Retardation Gels (6%) (Invitrogen, EC6365BOX). There is a strong correlation between the amount of the HU protein and the decrease of free DNA and the increased number of HU–DNA complexes. 1- standard (AccuBand 100 bp DNA Marker II, SMOB-DM2000), 2- control sample containing the DNA fragment in a binding buffer without any protein, 3–10 – samples containing the DNA fragment in a binding buffer with increasing amounts of HU protein. Free DNA and HU–DNA binding complexes are depicted by red and white arrows respectively.
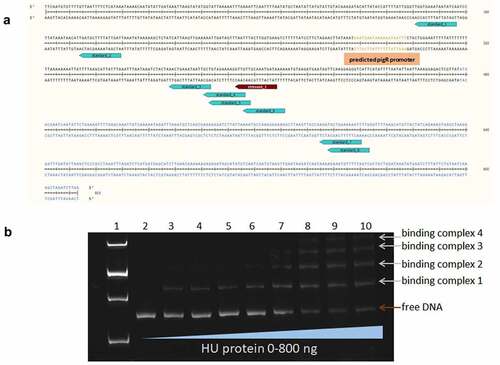
Figure 5. Arginine 58 and arginine 61 are essential for the DNA binding capacity of HU protein. The role of R58, R61, and S74 on DNA binding capacity in comparison with the WT form of HU_HA protein was tested using EMSA. 500 ng of WT HU_HA protein or HU protein mutant variants were mixed with 60 ng of (a) 477 bp DNA sequence upstream of the pigR gene or (b) 568 bp DNA sequence corresponding to the part of clpB gene (identified in ChIP-seq analysis) in a binding buffer for 20 min on ice and resolved on 1% TBE/agarose gel. ST – molecular weight standard (GeneRuler 1 kb DNA Ladder, Thermo, SM0311), DNA – control sample containing the DNA fragment in a binding buffer without any protein, WT, R58Q, R61Q, S74A, and BSA – samples containing the DNA fragment in a binding buffer with the appropriate protein.
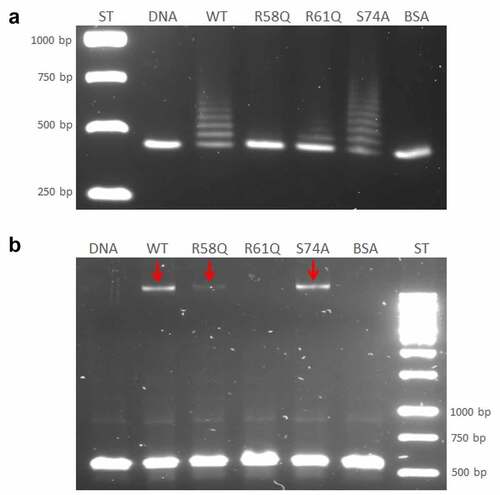
Figure 6. Semi-quantitative RT-PCR demonstrates decreased transcription level of pigR gene in FSC200/ΔHU and FSC200/HU_HA/R58Q. Expression of pigR in all tested strains was verified on transcription level using reverse transcription followed by PCR. Samples were analysed by gel electrophoresis. pigR showed significantly decreased expression in deletion mutant strain and FSC200/HU_HA/R58Q in contrast to WT. This result suggests HU protein participation in pigR regulation.
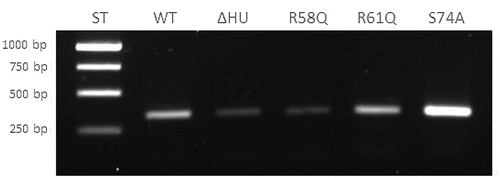
Figure 7. Mutation in arginine 58 affects the ability of HU protein to introduce negative DNA supercoiling. Effects of R58 (b), R61(c), and S74 (d) mutations on the ability of HU protein to introduce negative DNA supercoiling in the presence of human topoisomerase I in comparison to the WT form of HU protein (a) was investigated. 1, 9 - molecular weight standard (GeneRuler 1 kb DNA Ladder, Thermo, SM0311), 2 - supercoiled pBluescript KS- DNA incubated in an assay buffer without any protein, 3 - supercoiled pBluescript KS- DNA incubated in an assay buffer with 1500 ng of appropriate HU protein and no human topoisomerase I, 4–8 - supercoiled pBluescript KS- DNA incubated in an assay buffer with 4 U of human topoisomerase I and the increasing amount of corresponding HU proteins (0, 187.5, 375, 750, and 1500 ng).
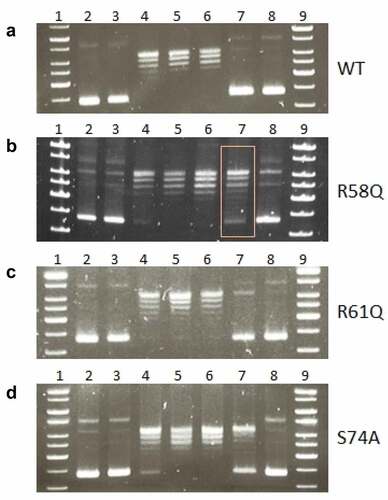
Figure 8. AFM images of ΔHU chDNA in the absence/presence of WT and mutant HU protein variants. Comparison of ΔHU chDNA compactness changes depending upon HU protein variant addition. Two representative images (different scale) of FSC200/ΔHU strain chDNA combined with HU protein variants are displayed in each row. The height profile scale is shown on the right.
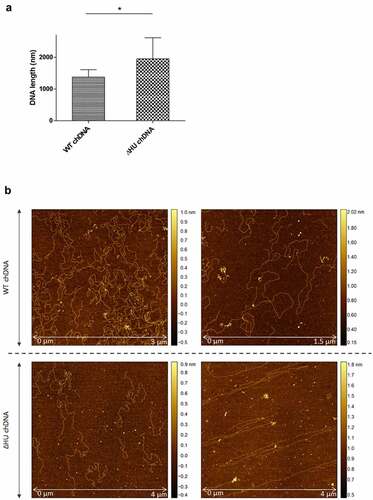
Figure 9. Intracellular replication of F. tularensis FSC200 and mutant strains with point mutations in hupB gene inside BMMs. Macrophages were infected with FSC200 or tested mutant strains at MOI of 50 and the numbers of replicating bacteria expressed as CFU counts were determined at 1, 6, 24, and 48 h post infection. Error bars represent the mean ± sd derived from three independent experiments. Statistical significance was analysed using Two-way ANOVA followed by Bonferroni’s multiple comparison test. P-value <0.05 *, P < 0.01 **, P < 0.001 ***, ns not significant.
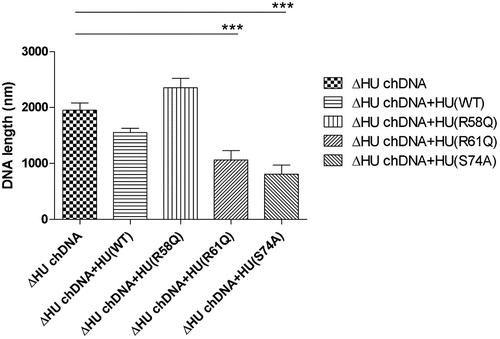
Figure 10. Survival of BALB/c mice upon infection of F. tularensis. The dose of 102 CFU of WT strain FSC200 or mutant strains FSC200/HU_HA/R58Q, FSC200/HU_HA/R61Q, FSC200/HU_HA/S74A was administered (s.C.). Mice were observed daily for the following 30 days. All mice infected with WT strain FSC200 died within 7 days post infection whereas all mice infected with mutant strains survived. On day 30, these mice were challenged by infection with FSC200 strain (the dose of 3 × 102 CFU/mouse) to study the ability of corresponding mutant strains to elicit protective immunity against WT strain. 90 % or 80 % of mice immunized with FSC200/HU_HA/R61Q or FSC200/HU_HA/S74A respectively and 100 % of mice immunized with FSC200/HU_HA/R58Q strain survived the WT challenge.
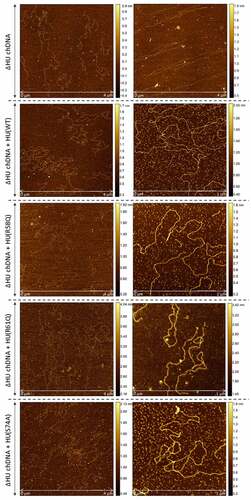
Figure 11. The effect of HU protein on the length and shape of Francisella chDNA. (A) Comparison of lengths of chDNA isolated from WT and ΔHU strains measured after AFM visualization revealed significant differences. The absence of HU protein caused an extension of ΔHU strain chDNA of about 30 %. Student´s t-test P-value <0.05* (B) AFM images of chDNA from WT and ΔHU strains. The chDNA from the WT strain showed an ordinary compact structure whereas chDNA from the ΔHU strain was more relaxed and long straight strands were observed. For both strains two images of chDNA obtained from different AFM scans are displayed in row. The height profile scale is shown on the right.
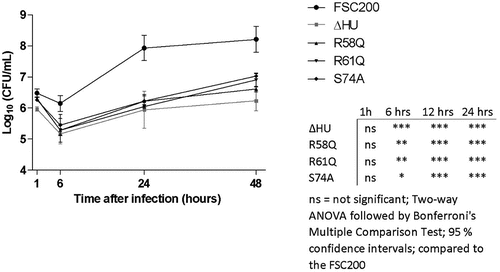
Figure 12. Survival of BALB/c mice upon infection of F. tularensis. The dose of 102 CFU of WT strain FSC200 or mutant strains FSC200/HU_HA/R58Q, FSC200/HU_HA/R61Q, FSC200/HU_HA/S74A was administered (s.c.). Mice were observed daily for the following 30 days. All mice infected with WT strain FSC200 died within 7 days post infection whereas all mice infected with mutant strains survived. On day 30, these mice were challenged by infection with FSC200 strain (the dose of 3 x 102 CFU/mouse) to study the ability of corresponding mutant strains to elicit protective immunity against WT strain. 90 % or 80 % of mice immunized with FSC200/HU_HA/R61Q or FSC200/HU_HA/S74A respectively and 100 % of mice immunized with FSC200/HU_HA/R58Q strain survived the WT challenge.
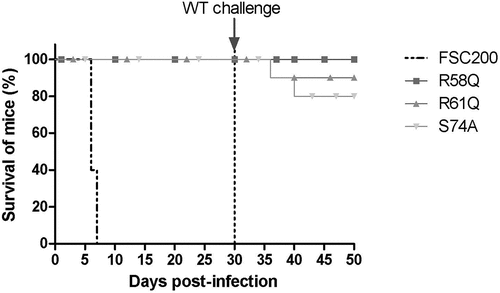
Supplemental Material
Download Zip (152.7 KB)Data availability statement
The data that support the findings of this study are openly available in this manuscript and supplementary files.