Figures & data
Table 1. Streptococcal strains included in this study.
Table 2. Oligonucleotides used in this study.
Figure 1. Effects of pepO and cppA on complement activation. Bacterial binding to C3b, C4BP, fator H, C1q and SAP were compared between pepO or cppA mutants (grey columns on the left side) with the parent strain SK36 (black columns) and the respective complemented strains (hatched columns). Intensities of protein binding were determined by flow cytometry in strains treated with 20% human serum or PBS (negative controls) and expressed as fluorescence index (FI). Columns represent means of three independent experiments. Bars indicate standard deviations. Asterisks indicate significant differences in relation to SK36 (Kruskal-Wallis with post hoc Dunn’s test; *p < 0.05).
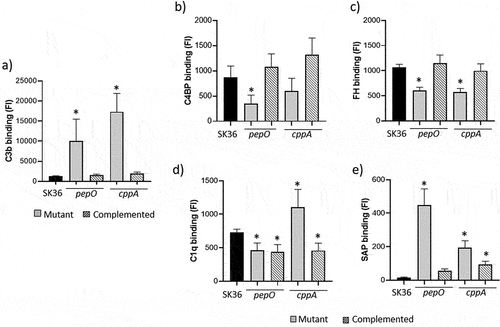
Figure 2. Effects of pepO and cppA on S. sanguinis susceptibility to phagocytosis by PMN and persistence in human blood. Mutants of pepO (SKpepO) and cppA (SKcppA) were compared to SK36 and the respective complemented strains (+). a) Phagocytosis by PMN isolated from blood were assessed by flow cytometry after incubation with FITC-labelled strains in the absence (PBS) or presence of 20% human serum (HS). Asterisks indicate significant differences in relation to SK36 at the same condition (Kruskal-Wallis with post hoc Dunn’s test; *p < 0.05). b) Comparisons of bacterial counts (log UFC/ml) in blood suspensions. Initial counts (time 0) were determined just after bacterial suspension in human blood. Asterisks indicate significant differences in bacterial counts in relation to SK36 at each time point. Kruskal–Wallis with post hoc Dunn’s test (p < 0.05).
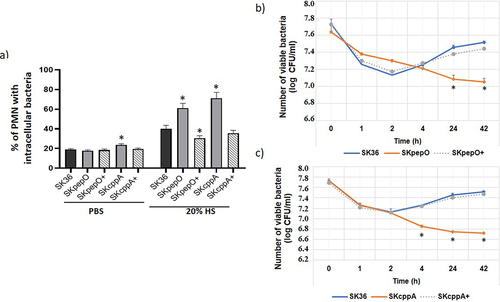
Figure 3. Contribution of pepO and cppA to the S. sanguinis capacity to invade human coronary artery endothelial cells. Bacterial invasion into primary HCAEC was determined in antibiotic-protection assays with pepO and cppA mutants, SK36 and complemented strains (+). Strains were treated with human serum (HS) (a,b), heat-inactivated serum (HIS) (c,d) or PBS (e,f) before co-cultivation with HCAEC. Invasion rates were expressed as the percentage of intracellular bacteria in relation to the initial inoculum. Columns represent means of three independent experiments. Bars indicate standard deviation values. Asterisks indicate significant differences in relation to SK36 (Kruskal-Wallis with post hoc Dunn’s test; *p < 0.05).
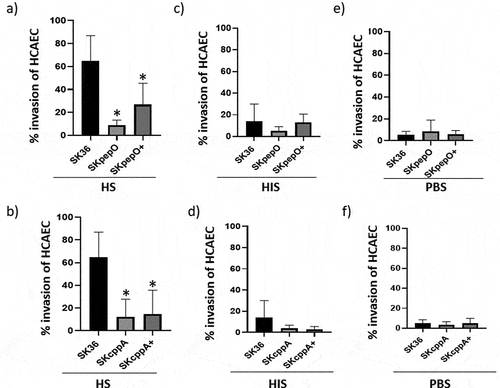
Figure 4. Contribution of pepO and cppA to S. sanguinis virulence. Galleria mellonella larvae were infected with the S. sanguinis strains SK36, mutants of pepO (SKpepO) and cppA (SKcppA), and the respective complemented strains (+). Larvae inoculated with PBS were used as negative controls. a) Kaplan–Meier survival curves. Percents of larvae survival were compared between the mutant strains with SK36 (indicated by asterisks) or with the respective complemented mutant (indicated by crosses) at p < 0.001 (log rank test). b) Curves of health index scores of larvae infected with S. sanguinis strains or with PBS (negative control). Significant increases in health index scores were observed in larvae infected with SKpepO or SKcppA in relation to SK36 (indicated by asterisks) or to the respective complemented mutant (indicated by crosses) (Unpaired t test; p < 0.001).
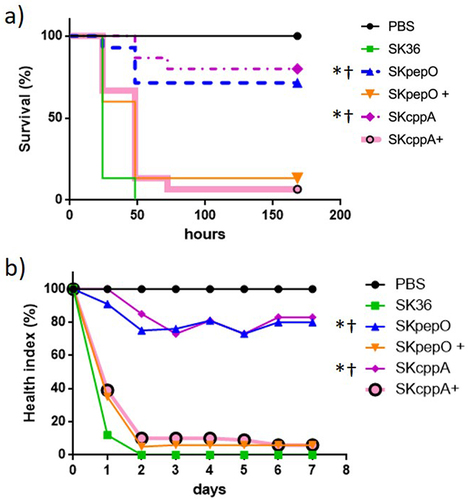
Figure 5. Transformation efficiency of S. sanguinis strains. Strains at A660 nm 0.7 to 0.8 were incubated with plasmid pDL278 during 90 min. and the transformants recovered in agar BHI with spectinomycin. The number of transformants obtained per µg of plasmid DNA was expressed as transformation efficiency. a) Comparisons of the transformation efficiency of mutant strains (SKpepO and SKcppA) with SK36. b) Comparisons of the transformation efficiency of eight clinical strains with SK36. Columns represent means of four independent experiments; bars represent standard deviations. Asterisks indicate significant differences in relation to the reference strain SK36 (Mann Whitney test; p < 0.05).

Figure 6. Transcription analysis of pepO and cppA in S. sanguinis strains. Levels of gene transcripts were determined by RT-qPCR in samples with equal number of bacterial cells at A550 nm 0.3. a) Levels of pepO transcripts normalized by the respective levels of 16S rRNA gene. b) Levels of cppA transcripts normalized by the respective levels of 16S rRNA gene. Columns represent means of three independent experiments; bars indicate standard deviation. Asterisks indicate significant differences in relation to the reference strain SK36 (Kruskal-Wallis with post hoc Dunn’s test; p < 0.05).
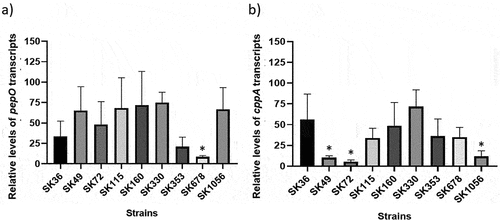
Figure 7. Polymorphism and phylogenetic analysis of PepO and CppA. CppA and PepO protein sequences obtained from the genomes of SK36 (reference) and of eight S. sanguinis strains (partial genomes) available that the GenBank were compared by multiple sequence alignment using ClustalW (http://www.Ebi.ac.uk/Tools/msa/clustalw2/). Phylogenetic analyses of PepO and CppA homologues were performed using the PhyML platform (https://ngphylogeny.Fr/). a) Multiple alignment of a polymorphic region (position 61…180) of PepO including conservative and non-conservative amino acid substitutions (marked in blue). b) Phylogram of streptococcal PepO. A PepO homologue of Enterococcus faecium was used as outgroup. c) the polymorphic region (position 121…240) of CppA including non-conservative amino acid substitutions (marked in green). c) Phylogram of streptococcal CppA. A CppA homologue of Lactococcus lactis was used as outgroup. S. sanguinis strains isolated from blood samples are marked in red. Protein accession numbers of PepO homologues: S. gordonii (MBN2959327.1); S. parasanguinis (MBS5358381.1); S. pneumoniae (WP_061759435.1); S. mitis (WP_125455933.1); S. oralis (WP_125440898.1); S. salivarius (WP_195968955.1); S. mutans (WP_002262350); S. pyogenes (WP_009880612.1); Enterococcus faecium (EGP5219851.1). Protein accession numbers of CppA homologues: S. gordonii (KXT70936); S. parasanguinis (WP_272157479.1); S. mitis (WP_049502209.1); S. pneumoniae (WP_054394392.1); S. oralis (KXT93124.1); S. mutans UA159 (WP_002262622); S. salivarius (WP_195413538.1); S. pyogenes (WP_032462135.1); Lactococcus lactis (WP_259742941.1).

Data availability statement
The data that support the findings of this study are openly available in https://doi.org/10.25824/redu/U4IJL0.