Figures & data
Figure 1. Rab proteins as molecular switches. (a) After being synthesized, Rab proteins bind to the Rab escort protein (REP) and become prenylated by RabGGTase at C-terminal cysteine residues. The prenylated protein can be solubilized in the cytosol by the GDP dissociation inhibitor (GDI), which shields the hydrophobic geranylgeranyl groups from the hydrophilic environment (1). Guanine nucleotide exchange factors (GEFs) catalyze nucleotide exchange and help to recruit Rabs to certain membranes within the cell (2). In their GTP-bound active state, Rabs interact with effectors and regulate different steps in vesicular trafficking (budding, vesicular transport along the cytoskeleton, tethering and fusion with a target membrane; 3). Finally, inactivation takes place via interaction with GTPase activating proteins (GAPs) to yield the GDP-bound Rab (4). (b) Comparison of Rab proteins bound to GppNHp (left; Rab5 (1HUQ), Rab8 (4LHW), Rab9 (2OCB), Rab11 (2F9M), Sec 4 (1G17) and Ypt7 (1KY2)) and bound to GDP (right; Rab5 (3CLV), Rab8 (4LHV), Rab9 (1WMS), Rab11 (2F9L), Sec 4 (1G16) and Ypt7 (1KY3)). The nucleotide (sticks) and Mg2+ (green sphere), switch I (red), switch II (blue) and the P-loop (magenta) are highlighted (N: N-terminus, C: C-terminus). Note the switch regions that adopt an ordered and defined conformation in the active state, whereas more flexible and less well defined conformations can be seen in the inactive Rabs. (c) A sequence alignment of different representatives of Rab proteins from different Rab families (according to Klöpper et al.Citation134) is shown highlighting important features within the sequences. The P-loop (magenta), switch I (red) and switch II (blue), the nucleotide-binding G-motifs (green, G1-G5) as well as the C-terminal cysteine residues used for prenylation (brown) are highlighted. Additionally, the position of Rab-family (RabF, gray) and Rab-subfamily (RabSF, orange) motifs that play an important role in recognition of general Rab interacting proteins or Rab family specific interactions, respectively, are indicated. The secondary structure (α-helices 1–5 and β-sheets 1–6) is shown below the aligned sequences.
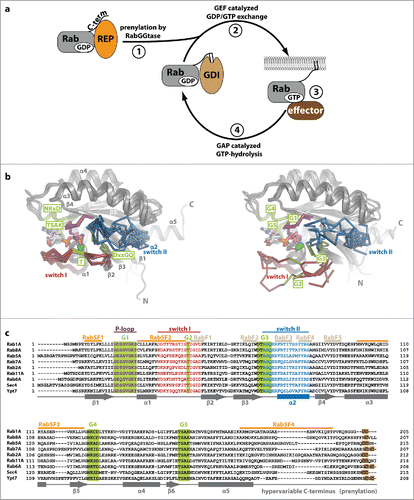
Table 1. Rab proteins and their known GEFs and GAPs.
Figure 2. Conformational changes during GEF-catalyzed nucleotide exchange. (a) Structures of Rab1b:GppNHp (pdb id 3NKV) and the Rab:GEF complexes Rab21:Rabex-5 (pdb id 2OT3), Ypt1:TRAPP (pdb id 3CUE), Rab35:DennD1 (pdb id 3TW8) and Rab1b:DrrA (pdb id 3JZA) Sec 4:Sec 2 (pdb id 2EQB and 2OCY). The relative distance of residues of the different Rab proteins (Cα-positions) within the P-loop, switch I and switch II compared with Rab1b:GppNHp is shown below each structure, highlighting the structural changes during GEF-catalyzed nucleotide exchange. Note the structures of Sec 4:Sec 2 in the presence of a PO42−-ion (pdb id 2EQB) and Sec 2:Sec 4 in the absence of a PO42−-ion (pdb id 2OCY), showing the collapsed state of the P-loop due to missing interactions with a negatively charged ion. (b) A comparison of the P-loop conformation of myosin bound to a nucleotide analogon (ADP-Metavanadate; pdb id 3MJX) and of the P-loop conformation of myosin in the absence of any nucleotide (pdb id 2AKA) indicates that the collapsed state of the P-loop is solely caused by missing interactions with a negatively charged phosphate group, not by binding of an exchange factor.
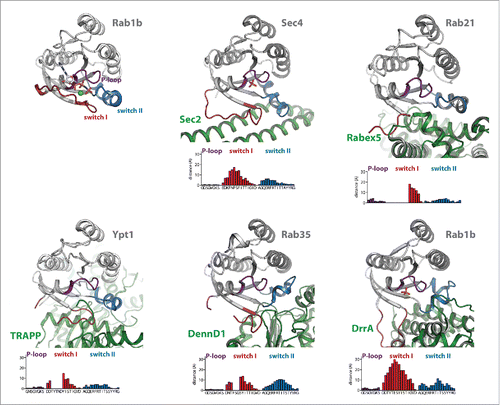
Figure 3. Dual- and single-finger mechanism used by RabGAPs. The structures of Gyp1:Rab33 (a) and LepB:Rab1 (b) are shown (left; Rab: gray cartoon with the switch I and II in red and blue, respectively and the P-loop in magenta (the switch regions and the P-loop are shown as ribbon), the GAP is shown as rainbow colored cartoon) and a zoom into the active site (right; residues provided by the GAP are colored in green, residues of the Rab protein in gray). Both structures were obtained in the presence of GDP and BeF3 or AlF3 as transition state mimetics of the hydrolysis reaction. (a) Gyp1 uses a dual-finger mechanism to catalyze GTP-hydrolysis and provides both Arg343 and Gln378 (green) in trans. The G3-motif Gln92 (gray) of Rab33 is repositioned by interactions with backbone atoms of Gyp1 and does not participate in catalysis. (b) In contrast to TBC-domain GAPs, the Legionella pneumophila GAP LepB uses a single-finger mechanism and provides only one residue (Arg444, green). Gln67 of Rab1b remains in its usual conformation to position the attacking water for the in-line attack of the γ-phosphate.
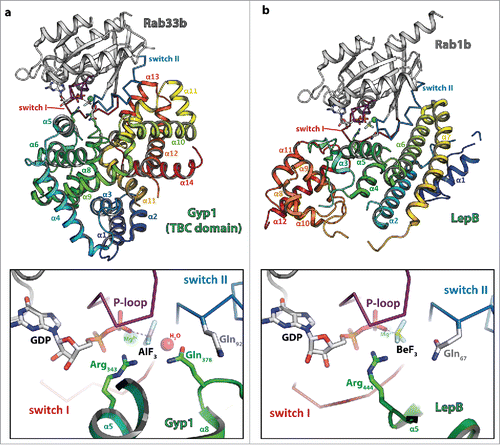
Figure 4. The interaction of Rab proteins with REP, RabGGTase and GDI. (a) Model of the ternary complex between Rab7:GDP (gray, switch I – red, switch II – blue, P-loop – magenta, Mg2+ - green sphere, GDP - sticks), REP (cyan) and RabGGTase (α-subunit – green, β-subunit – blue, Zn2+ - orange sphere). The ternary complex was modeled from the structures of Rab7:REP (pdb id 1VG0) and REP:RabGGTase (pdb id 1LTX). (b) Model of doubly prenylated Ypt1:GDP (colors as above) in complex with GDI (yellow, pdb id 2BCG). Note the structural similarity between Rab7:REP and Ypt1:GDI. (c) Close-up view of helices D, E and H of the lipid-binding domain II of REP in complex with RabGGTase (left) or in complex with one geranylgeranyl-group (middle) and of the corresponding domain II of GDI in complex with 2 geranylgeranyl-groups (right). A conformational change within this domain upon binding of the C-terminally linked geranylgeranyl-groups of the Rab is presumably the cause of dissociation of RabGGTase from REP subsequent to prenylation.
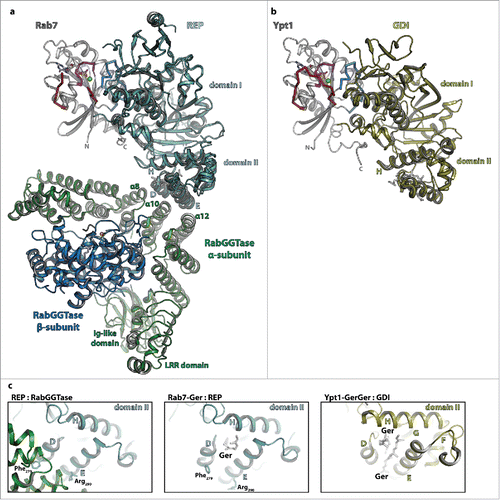