Figures & data
Figure 1. Sequence alignment of human Rab G-domains. Conserved nucleotide binding motifs are highlighted: PM1-PM3 - phosphate, magnesium binding motifs; G1-G3 - guanine moiety binding motifs. Rab family specific motifs (RabF1–5), Rab subfamily specific motifs (RabSF1–4) as well as C-terminal interacting motif (CIM) are highlighted. C-terminal cysteines (red) within geranylgeranylation motifs (GGM) are found in one of the following combinations: XXXCC, XXCCX, XCCXX, CCXXX, XXCXC and XCXXX. Hydrophobic triad residues (see ) are marked in yellow. Secondary structure elements corresponding to the Rab3 structure (3RAB) are shown on the top, as well as Switch-1, Interswitch, Switch-2, complementarity-determining regions (CDR1–5) and the hyper-variable C-terminal domain (HVD). Rab sequences are presented in an order of proximity in the phylogenetic tree of human Rabs.Citation4 The G-domains of closely related Rabs (Rab26 and Rab37) share 76% sequence identity while the more diverse family members (Rab1A and Rab20) exhibit as low as 16% G-domain identity. Among the human Rabs the protein lengths vary between 194 (Rab22a) to 740 residues (RasEF45 or Rab45). Residue conservation color code: red -negatively charged, blue - positively charged, polar - magenta, hydrophobic - green, prolines and glycines -brown. The protein sequences Uniprot database accession numbers: RAB23 Q9ULC3; RAB29 O14966; RAB38 P57729; RAB32 Q13637; RAB9A P51151; RAB7A P51149; RAB28 P51157; RAB20 Q9NX57; RAB34 Q9BZG1; RAB36 O95755; RAB22A Q9UL26; RAB5A P20339; RAB17 Q9H0T7; RAB21 Q9UL25; RAB24 Q969Q5; RAB41 Q5JT25; RAB6A P20340; RAB30 Q15771; RAB33A Q14088; RAB43 Q86YS6; RAB19 A4D1S5; RAB25 P57735; RAB11A P62491; RAB42 NP_001180461.1; RAB39A Q14964; RAB2A P61019; RAB14 P61106; RAB4A P20338; RASEF45 Q8IZ41; RAB44 Q7Z6P3; RAB12 Q6IQ22; RAB18 Q9NP72; RAB3A P20336; RAB27A P51159; RAB26 Q9ULW5; RAB37 Q96AX2; RAB10 P61026; RAB13 P51153; RAB8A P61006; RAB35 Q15286; RAB1A P62820; RAB15 P59190; RAB40A Q8WXH6.
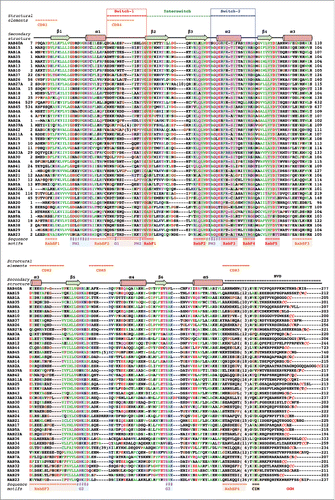
Figure 2. Structures of Rab GTPase and the diversity of their conformational switch. (A) Crystal structures of yeast Rab Sec4p in its GTP- and GDP-bound forms (1G17 and 1G16), the first Rab structures determined in both nucleotide bound states,Citation149 are shown. Conserved PM and G motifs are highlighted in cyan. Switch1, Switch2 and Interswitch (sequence between the 2 Switches) regions are shown in red, blue, and green, respectively. The nucleotides and magnesium ions are shown as dark-gray sticks and spheres, respectively. The color scheme is used throughout all figures. (B) Diverse nucleotide-dependent conformational changes in Rabs. Ypt32Citation13 and Rab28Citation14 structures in GTP- and GDP-bound states are shown. (C) Comparison of the conformations of GTP-bound Rabs and GDP-bound Rabs. Left: superimposition of GTP-bound structures of Rab1b (3NKV), Rab2 (4RKE), Rab3a (3RAB), Rab4a (2BME), Rab5c (1HUQ), Rab6a (1YZQ), Rab7a (1T91), Rab8a (4LHW), Rab9a (1YZL), Rab11a (1OIW), Rab18(1×3S), Rab21 (1YZT), Rab22a (1YVD), Rab26 (2G6B), Rab28 (3E5H), Rab30 (2EW1), Rab33b (1Z06), Sec4p (1G17), Ypt1 (1YZN), Ypt32 (3RWM), Ypt51 (1EK0), and Ypt7 (1KY2). Right: superimposition of GDP-bound structures of Rab1a (2FOL), Rab2a (1Z0A), Rab3d (2GF9), Rab4a (2O52), Rab5a (1TU4), Rab6b (2E9S), Rab7a (1VG1), Rab8a (4LHV), Rab9a (1S8F), Rab11a (1OIV), Rab12 (2IL1), Rab14 (1Z0F), Rab21 (1Z0I), Rab23 (1Z22), Rab25 (2OIL), Rab28 (2HXS), Rab45 (2P5S), Sec 4 (1G16), Ypt32 (3RWM), and Ypt7 (1KY3).
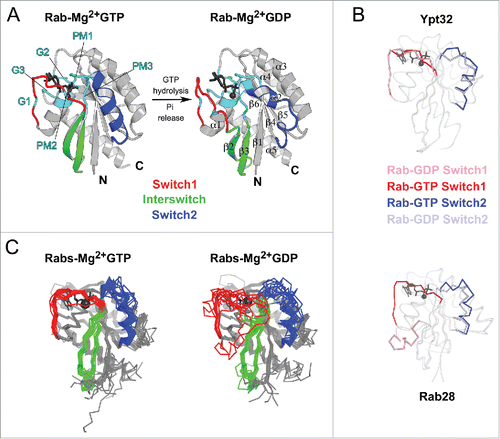
Figure 3. Rab functional cycle. The newly synthesized GDP-bound Rab is recognized by REP and presented to RabGGT, which geranylgeranylates the Rab on one or two C-terminal Cys residues. Targeting of the Rab/REP (or Rab/GDI) complex to specific membranes is mediated by the interaction with a membrane-associated GEF, which stimulates GDP to GTP exchange of Rab; or in few cases by interaction with a GDF first, followed by GEF stimulated activation. The GTP-bound (active) Rab conformation is recognized by multiple effector proteins, which then carry out their specific functions. GAP stimulates Rab GTPase activity and converts Rab back to the inactive, GDP-bound form, resulting in the dissociation of effectors. GDI regulates the cycling of Rab between the membranes by extracting inactive Rab from a membrane into the cytosol and subsequently delivering it back to a donor membrane compartment.
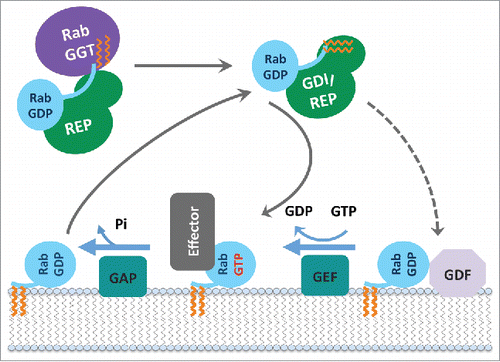
Figure 4. Rab partner recognition sites. Rab (Rab3a, 3RAB) Mg2+-GTP (dark gray) bound structure with the structural elements contributing to partner binding highlighted. (A) Side view showing the Rab canonical effector binding site composed of Switch1, Interswitch, and Switch2; surrounded by complementarity determining regions (CDR1–5, shown in orange). Conserved Rab hydrophobic triad is shown in yellow sticks. The surface representation is shown in the middle, and conservation of surface residues (based on the sequence alignment in ) generated using ConSurf web server Citation150 is presented on the right. (B) Top view showing a new, secondary Rab effector binding site composed of the CDR4 and CDR5 regions flanking the nucleotide binding pocket.
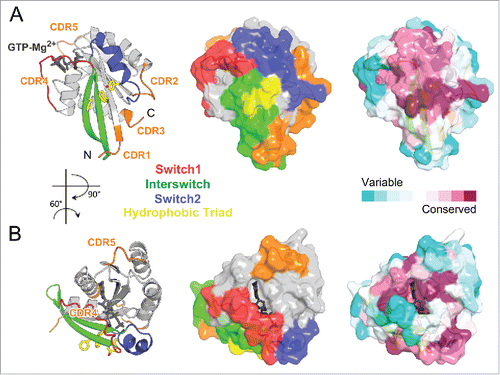
Figure 5. Universal Rab partners support Rab prenylation and recycling. (A) A model of Rab prenylation ternary complex based on Rab7a/REP1 (1VG0) and REP1/RabGGT (1LTX) complex structures. Rab7a, REP1 (composed of 2 domains D-I and D-II), and RabGGT are shown in light blue, green, and violet; respectively. Prenyl moiety bound to RabGGT active site is shown in yellow sticks. (B) (Left) Rab7a residues involved in REP1 binding are shown as spheres and colored by conservation. (Right) Rab27b-GDP forms a dimer by swapping Switch1-Interswitch-Switch2 regions between the monomers in the crystal.Citation41 Small angle X-ray scattering (SAXS) data demonstrate, however, that Rab27b-GDP is monomeric in solution and adopts an atypical extended structure.Citation41 (C) Structure of prenylated Ypt1p (light blue), in complex with yeast RabGDI (green). The prenyl moiety bound to RabGDI D-II is shown in yellow sticks (1UKV).
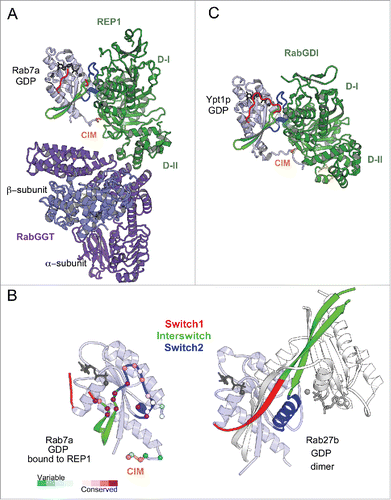
Figure 6. Rab nucleotide exchange factors of different structural folds. Left: Conformational changes in Rabs upon interactions with cognate GEFs. Rab residues contacting GEF (within 4 Å distance) are shown as spheres. PM-G motif residues are cyan, CDR residues are orange. Rab8a-GTP structure (4LHW) is shown as a reference for the Switch1, Switch2 conformations in a GTP-bound Rab (top). Right: Structures of Rab/RabGEF complexes: Rab21/Rabex5-HB-VPS9-domain (2OT3), Rab35/DENND1B-DENN-domain (3TW8), Rab1a/DrrA-GEF-domain (2WWX), Rab8a/Rabin8-GEF-domain (4LHX), Ypt7p/Mon1-Ccz1-complex-core (5LDD), Ypt1p/TRAPPI-complex-core (3CUE). Rabs are shown in light blue and GEFs are shown in green hues.
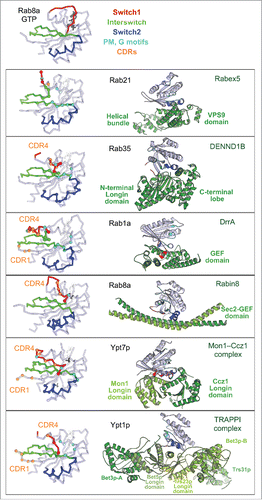
Figure 7. Rab effector binding surface. Structures of Rab-GTP molecules bound to effectors are shown (cartoon) and superimposed (line) to represent their effector binding sites. Residues changing their solvent assessable area upon binding to the effectors are shown in black lines, hydrophobic triad residues are shown in yellow sticks. (A) Rab5/22-effector complexes. Structures of homologous Rab5 and Rab22 bound to their effectors' RBDs, including EEA1-C2H2-RBD (3MJH), Rabaptin5-Cterminal-RBD (Rapt5; 1TU3), Rabenosyn5-(728–784)-RBD (RBSN5; 1Z0J), and VipD (4KYI), are shown. The superimposed structures illustrate that the effector binding site is similar among different Rab-effector interactions. Rab5/22 specific residues contributing to binding specificity are highlighted with cyan circles. (B) Rab3/27-effector complexes: Rab3a/Rabphilin3a-RBD (RPH3a; 1ZBD), Rab27a/Exophilin4-RBD (EXPH4; 3BC1), and Rab27b/Melanophilin-RBD (MLPH; 2ZET). The effector binding site is extended to the CDR1–3 regions. Cyan circles highlight residues that are different between Rab3 and Rab27, contributing to Rab27 selectivity. (C) Rab11a bound to the RBDs of effectors Rab11FIP2 (4C4P), Rab11FIP3 (2HV8), MyosinVa (MyoVa; 5JCZ), and MyosinVb (MyoVb; 4LX0) are shown. The binding site undergoes remodeling in Switch1, Switch2 and the hydrophobic triad. (D) Rab6a binds 2 effectors RBDs, GCC185 (3BBP) and Rab6IP1 (3CWZ), in different conformations. (E) Rab7a binding to RILP-RBD (1YHN) induces remodeling of the C-terminal CDR3 region that forms an additional β-strand (β7). (F) Rab1b, Rab8a and Rab10 interact with Mical-family (Mical-cl and Mical1) (5SZH, 5SZI, 5LPN, 5SZJ) RBDs using similar binding sites except for the CDR1 region, which modulates the partners binding affinities. In the case of Rab8a, complex structures of it bound to different effectors' RBDs, including OCRL1 (3QBT), Mical-cl (5SZI) and LidA (3TNF), demonstrate subtle structural rearrangements in Switch1 and Switch2.
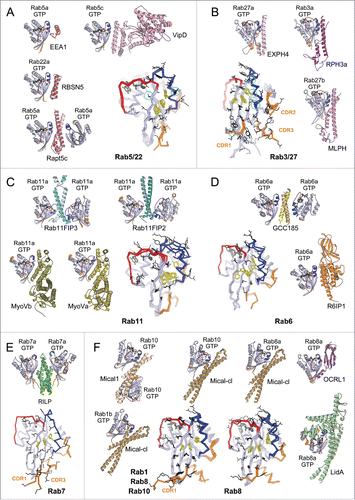
Figure 8. Structures of Rab-GAP complexes and the mechanisms of GAP stimulated GTP hydrolysis. Representative Rab/RabGAP complexes: Rab33b/Gyp1-TBC-domain (2G77), Rab1a/VirA-TBC-like-domain (4FMB), and Rab1a/LepB-GAP-domain (4IRU). (A) TBC domain Gyp1 and bacterial RabGAP VirA have different folds but stimulate Rab GTP hydrolysis using the same, dual trans-finger mechanism exploiting the trans-Gln-finger and trans-Arg-finger from the conserved TBC YxQ and IxxDxxR motifs, respectively. The nucleophilic water is shown as a pink sphere. The cis-Gln in the Rab Switch2 PM3 motif contributes to GAP binding. (B) LepB, a bacterial RabGAP, binds to Rab1a and generates a trans-cis polar network where the GAP supplies the trans-Arg-finger and trans-Glu to the GTPase hydrolytic site and the Rab provides the cis-Gln from the PM3 motif, Ser residues from the P-loop and Switch1, and the Arg from Switch2. The Switch1 Tyr contributes to destabilization of the Rab ground state as well as binding to LepB.
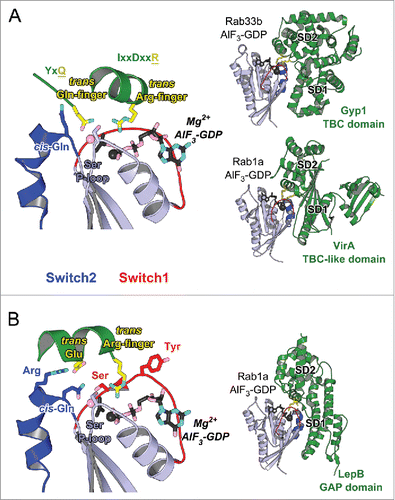
Figure 9. Effector conformational activation. (A) A schematic model of Mical1 activation upon Rab35 binding. Mical1s C-terminal domain, including the RBD, engages in auto-inhibitory interactions with its FAD-CH-LIM domains. Rab35 binding to the C-terminal domain promotes Mical1 enzymatic activity presumably by destabilizing the auto-inhibited state. (B) Dimeric EEA1 associates with early endosomes by binding to PI(3)P with its C-terminal FYVE domain. Its extended coiled-coil allows the N-terminal C2H2Zn2+ RBD to bind to Rab5-GTP, thereby capturing another early endosome. This interaction induces entropic collapse of its coiled-coil region, bringing 2 membranes together before endosomal fusion. (C) Rab11 cooperates with Spir in MyosinV activation and membrane recruitment. Spir and MyoV proteins adopt a back-folded, auto-inhibited conformation in the cytoplasm. Spir's globular tail binding motif (GTBM) binds to the inhibited MyoV and contributes to the release of MyoV auto-inhibition and facilitates MyoV-GTD interaction with Rab11 on vesicles. Together, they stabilize MyoV in an activated, extended conformation on the membrane. Spir's FYVE domain binds to the membrane and the WH2-KIND domains are available for interaction with Formin1 and F-actin nucleation.
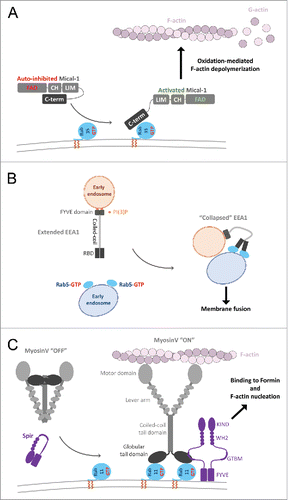
Figure 10. Rab dual-effector binding mode. (A) Non-canonical second effector binding site on Rab11a. Structures of PI4KIIIβ/Rab11a (4D0L, left) and Rabin8-RBD/Rab11a (4UJ5, right) complexes and the superimposition of their respective Rab11a (center) are shown. Residues changing solvent accessible area upon binding to the effectors are shown in black lines (middle). (B) PI4KIIIβ/Rab11a/Rab11FIP3-RBD complex structure (4D0M). Two Rab11a molecules bind to the Rab11FIP3-RBD dimer using the canonical effector-binding surface; and each binds to a PI4KIIIβ using the second binding sites. (C) A model of the Rab11-Rabin8-Rab8 cascade. Rabin8-RBD/Rab11a/Rab11FIP3-RBD complex structure (4UJ3, right) showing Rabin8-RBD dimer binds 2 Rab11a molecules using the second effector binding sites, while Rab11FIP3 interacts with Rab11a via the canonical site. Few direct contacts are observed between Rab11FIP3 and one of the Rabin8 RBDs. Rab11/Rab11FIP3 recruits Rabin8 by binding to its C-terminal dimeric RBD. Subsequently, the Rabin8 GEF domain can activate Rab8 (4LHX, left) and facilitate its membrane recruitment.
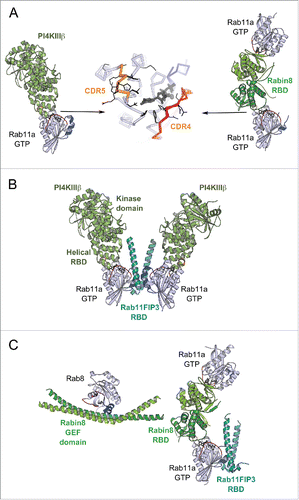
Figure 11. A model of the positive feedback loop mechanism. Crystal structure of minimal Rabaptin5/Rabex5/Rab5 complex (4Q9U, upper part) shows that the extended Rabaptin5 central coiled-coil domain binds to Rabex5s auto-inhibitory helix, whereas Rabex5 helix-bundle-VPS9 GEF unit interacts with the nucleotide free Rab5a. The structure of Rabaptin5-RBD/Rab5-GTP (1TU3, lower part) represents the complex's membrane-binding unit.
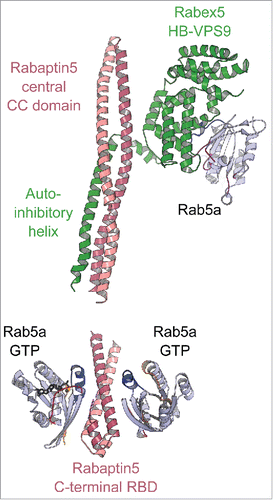
Figure 12. Bacterial Rab regulators. (A) Structure of active Rab1b modified by DrrA (3NKV). GTP analog and Mg2+ are shown in dark gray. The Tyr of Rab1b hydrophobic triad that is AMPylated (yellow) by DrrA is in the central part of the canonical partner binding site. The Rab1 Ser phosphocholination site in the Switch2 is also shown (cyan). (B) Legionella effector LidA bound to Rab8a (3TNF). Rab8a effector binding site (right) includes the canonical partner interaction surface (top) and expands to the adjacent surface (bottom). Rab8a residues changing solvent accessible area upon interaction with LidA are shown in black lines. (C) Legionella effector VipD bound to Rab5c (4KYI). Binding of Rab5c to VipD's helical RBD allosterically induces conformational changes in the phospholipase domain, resulting in opening of the catalytic site and activation of the enzymatic activity.
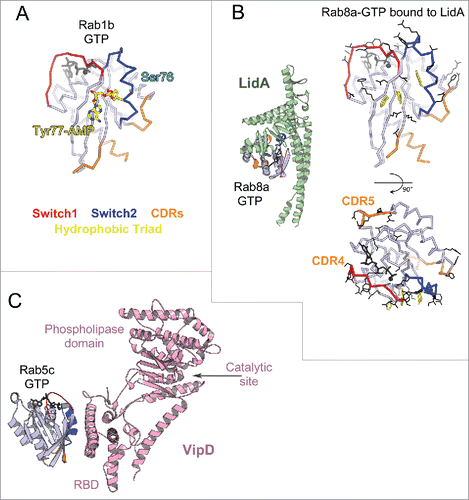