Figures & data
Figure 1. Rho-GTPases are master regulators of dendritic spines. (A) Confocal image of a dendritic segment showing spines from a mature rat hippocampal neuron expressing green fluorescent protein. Spine morphology is diverse, ranging from filopodia-like protrusions (spine precursors) to more mature thin, stubby, or mushroom-shaped structures (shown). The shape of a spine is highly correlated with the strength of its associated synapse, with the strongest synapses located on mushroom-shaped spines. Image by C. A. Cronkite. (B). Schematic of a dendritic spine and associated glutamatergic excitatory synapse. (C) Overview of Rho-GTPase signalling. Rho-GTPase activity is tightly regulated in space and time by GEFs, GAPs, and GDIs. GEFs activate Rho-GTPases by facilitating GDP/GTP exchange, whereas GAPs inactivate Rho-GTPases by enhancing GTP hydrolysis. GDIs also inhibit Rho-GTPases by sequestering them in an inactive state in the cytosol.
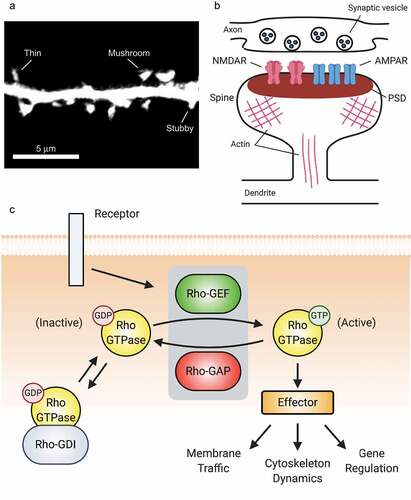
Figure 2. Synaptic receptors signal to Rho-GTPases via multiple pathways. (A) By regulating the function of different Rho-family GEFs, the EphB2 receptor tyrosine kinase controls Rac1, Cdc42, and RhoA signalling important for the actin cytoskeletal remodelling that drives spine and synapse development. (B) At synapses, the actions of individual Rho-GTPases are coordinated by multiple receptors.
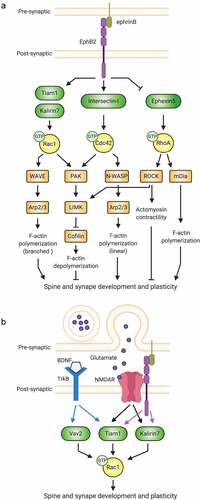
Figure 3. GEFs, GAPs, and multifunctional regulatory proteins tightly control Rho-GTPase activity. (A) The Tiam1/Bcr GEF/GAP complex regulates Rac1 activity during synaptogenesis. By forming a complex whose association can be modulated, the Rac1-GEF Tiam1 and the Rac1-GAP Bcr provide tight spatiotemporal regulation of Rac1 activation. (B) Tandem GEF/GAPs and GEF/GEF proteins contribute additional precise regulation to coordinated Rho-GTPase signalling. C2: protein kinase C conserved region 2, SEC14: domain in phosphatidylinositol transfer protein Sec14, SPEC: spectrin-like repeats, SH3: Src homology 3 domain, CC: coiled coil, Ig/FN3: Ig/fibronectin III.
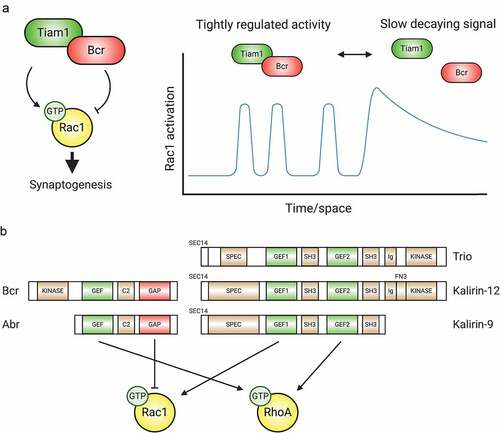
Figure 4. Downstream effectors modulate Rho-GTPase activity. (A) PAK regulates the activity of Rac1. Activated PAK associates with the Rac-GEF βPIX, increasing its GEF activity. Additionally, active PAK promotes Rac1 activation by phosphorylating and inactivating Rho-GDI, resulting in the release of sequestered Rac1. (B) Downstream effectors mediate crosstalk between Rac1, Cdc42, and RhoA. Following activation, Cdc42 recruits members of the Par polarity complex (Par3, Par6, and PKCζ), which in turn associate with the Rac1-GEF Tiam1, promoting local Rac1 activation. Similarly, PAK, ROCK, and mDia mediate crosstalk between Rac1 and RhoA.
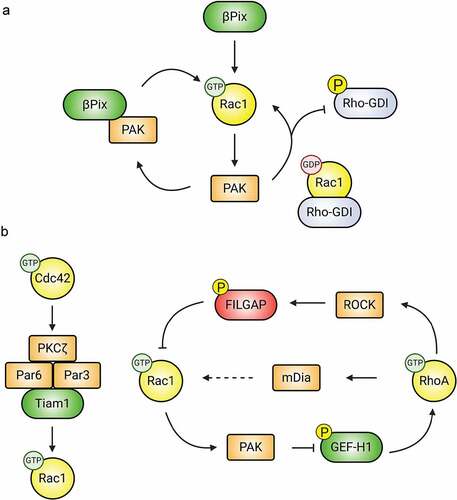
Figure 5. Rac1-GEFs differentially coordinate synaptic AMPAR insertion (LTP) and endocytosis (LTD) through Rac1-mediated actin cytoskeletal modifications. The surface expression of AMPARs during development and in response to LTP relies on Rac1 activity. Rac1-GEFs like DOCK4, Trio, and Kalirin-7 can activate Rac1 following LTP-inducing NMDAR activity. Subsequent Rac1-mediated actin cytoskeletal remodelling promotes the synaptic insertion of AMPARs. Conversely, Rac1 activity can also drive the downregulation of AMPAR surface expression in conditions promoting LTD. NMDAR-mediated signalling of Tiam1 and P-Rex1 activate Rac1 to promote synaptic AMPAR endocytosis.
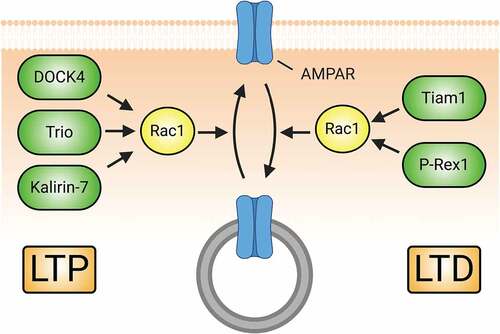
Figure 6. Dysregulation of Rho-GTPase signalling is involved in the pathologies of numerous neuropsychiatric disorders. (A) IRSp53 serves as a scaffold to mediate the interactions of Rac1 and Cdc42 with regulatory proteins. Under normal conditions, IRSp53 promotes actin stabilization and synaptic maturation by promoting Rac1 and Cdc42 activity. Loss of IRSp53 activity through mutation or hypomethylation of BAIAP2, the gene encoding IRSp53, is associated with the development of schizophrenia. The reduction in IRSp53 levels limits the activity of GEFs to activate Rac1 and Cdc42, thereby diminishing pro-spinogenic Rho-GTPase-mediated actin cytoskeletal changes and reducing dendritic spine density. Additionally, diminished levels of Kalirin-7 at the protein and mRNA levels have been observed in schizophrenia patients. This reduction can directly lead to decreased size and density of dendiritic spines. (B) Numerous Rho-GEFs and -GAPs are implicated in the pathophysiology of intellectual disability (ID). For example, multiple mutations in the Rac-GEFs TRIO and α-PIX have been found in individuals with ID. Loss-of-function of these proteins diminish Rac1 activation and the downstream signals associated with its activity. Likewise, DOCK4 is one of many Rho-GEFs that may be linked to the pathogenesis of autism spectrum disorder. The dysregulation of DOCK4 protein levels or activity reduces Rac1-mediated actin stabilization in addition to NMDAR subunit translation. This results in the reduction of both excitatory synapses through spine loss and LTP by the reduction in available NMDARs.
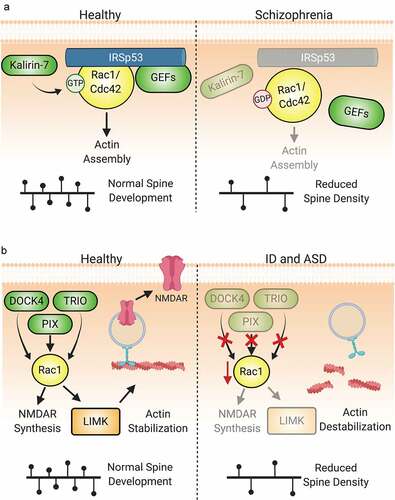
Figure 7. Aberrant Rho-GTPase signalling is also implicated in neurodegenerative diseases. (A) Both Rac1 and RhoA dysregulation are implicated in the pathogenesis of Alzheimer’s disease. A reduction in the ubiquitination and degradation of RhoA leading to an increase in ROCK1/2 activity promotes the aggregation of Aβ. This in turn further downregulates Ube3A-mediated ubiquitination of RhoA, propagating the aberrant signalling and increased amyloid plaque development. Rac1 signalling is also dysregulated due to reduced levels of the Rac1 effectors PAK1 and PAK3. The decrease in LIMK activation disinhibits cofilin, promoting actin cleavage and spine loss. (B) Rac1 activity is also implicated in several aetiologies of amyotrophic lateral sclerosis (ALS). Loss-of-function or downregulation of Rac1-GEFs such as Alsin and ARHGEF16 are associated with both juvenile- and adult-onset ALS. Mutations in SOD1, strongly associated with ALS development, also affect Rac1. Mutant SOD1, as well as an increased oxidative environment found in ALS, inhibit Rac1 by promoting GTP hydrolysis.
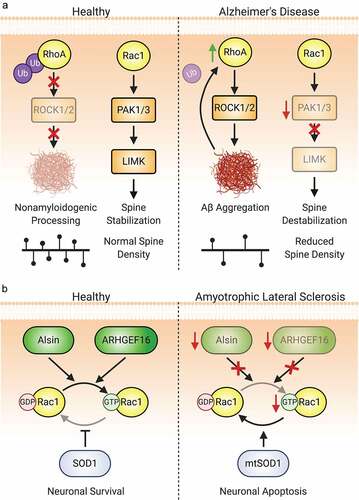