Figures & data
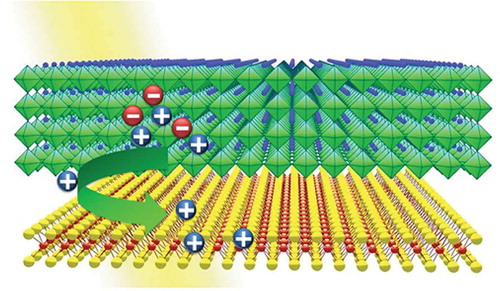
Figure 1. (a) Device schematic diagram. (b) The energy level diagram of the CsPbBr3-PC71BM bilayer photodetector under the illumination of hν > 2.3 eV. Photodetector performances of EQE (c) and photodetectivity (d) with the relationship of light irradiance, respectively [Citation33]. Copyright from 2018 Chem. Phys. Lett.
![Figure 1. (a) Device schematic diagram. (b) The energy level diagram of the CsPbBr3-PC71BM bilayer photodetector under the illumination of hν > 2.3 eV. Photodetector performances of EQE (c) and photodetectivity (d) with the relationship of light irradiance, respectively [Citation33]. Copyright from 2018 Chem. Phys. Lett.](/cms/asset/68993903-5b33-4f88-a3a5-1b27d2e97441/tapx_a_1592709_f0001_oc.jpg)
Figure 2. (a) Schematic illustration of the VGHP photodetectors. (b) Responsivity and detectivity of the reference and VGHP photodetectors [Citation35]. Copyright from 2018 ACS Nano. (c) The SEM image of the nanowires and time response behavior of reversible on/off switching for HPNW-based photodetectors. (d) The detectivity performance of HPNW-based photodetectors fabricated with PbI2 molar ratios of 0.4 M and 0.8 M devices [Citation46]. Copyright from 2018 Nano Energy. (e) Schematic illustration of CH3NH3PbI3 NW array photodetector. (f) Plots of responsivity and photoconductive gain of the photodetector as a function of light wavelength [Citation49]. Copyright from 2017 Nano Lett.
![Figure 2. (a) Schematic illustration of the VGHP photodetectors. (b) Responsivity and detectivity of the reference and VGHP photodetectors [Citation35]. Copyright from 2018 ACS Nano. (c) The SEM image of the nanowires and time response behavior of reversible on/off switching for HPNW-based photodetectors. (d) The detectivity performance of HPNW-based photodetectors fabricated with PbI2 molar ratios of 0.4 M and 0.8 M devices [Citation46]. Copyright from 2018 Nano Energy. (e) Schematic illustration of CH3NH3PbI3 NW array photodetector. (f) Plots of responsivity and photoconductive gain of the photodetector as a function of light wavelength [Citation49]. Copyright from 2017 Nano Lett.](/cms/asset/8fd5c446-4cba-4cb4-a903-30e0eebb26b7/tapx_a_1592709_f0002_oc.jpg)
Figure 3. (a) Schematic of a 2D CH3NH3PbI3 platelet phototransistor. The upright inset showed the optical microscopy image of the 2D CH3NH3PbI3 platelet phototransistor. (b) Dependence of photocurrent and photoresponsivity on incident light power, the blue and black dots correspond to original data [Citation55]. Copyright from 2018 Nanomaterials. (c) Schematic image of the CH3NH3PbI3 vertical structure photodetector looking through the back side of the glass substrate. (d) The band alignment for the ITO/perovskite/Au vertical heterostructure [Citation56]. Copyright from 2017 J. Phys. D. (e) Schematic illustration of the mechanism of perovskite nanonet photodetector. (f) I–t curves of the CH3NH3PbI3 photodetectors based on nanonet and compact film illuminated under monochromatic light at 700 nm [Citation57]. Copyright from 2017 Adv. Funct. Mater.
![Figure 3. (a) Schematic of a 2D CH3NH3PbI3 platelet phototransistor. The upright inset showed the optical microscopy image of the 2D CH3NH3PbI3 platelet phototransistor. (b) Dependence of photocurrent and photoresponsivity on incident light power, the blue and black dots correspond to original data [Citation55]. Copyright from 2018 Nanomaterials. (c) Schematic image of the CH3NH3PbI3 vertical structure photodetector looking through the back side of the glass substrate. (d) The band alignment for the ITO/perovskite/Au vertical heterostructure [Citation56]. Copyright from 2017 J. Phys. D. (e) Schematic illustration of the mechanism of perovskite nanonet photodetector. (f) I–t curves of the CH3NH3PbI3 photodetectors based on nanonet and compact film illuminated under monochromatic light at 700 nm [Citation57]. Copyright from 2017 Adv. Funct. Mater.](/cms/asset/b9ab7eb1-0785-4ad4-8508-ec393fdedc1b/tapx_a_1592709_f0003_oc.jpg)
Figure 4. (a) Photocurrent spectra of different SWCNT/PbS-QDs hybrid devices made with QDs of different sizes, showing the responses in the NIR which can be tuned by varying the PbS-QDs size. (b) Comparison between the photocurrent and the corresponding responsivity of the SWCNT/MAPbI3-xClx hybrid and of the SWCNT/PbS-QDs/MAPbI3-xClx hybrid photodetectors as a function of the illumination power of a 980 nm laser. (c) Responsivity and photoconductive gain of the SWCNT/PbS-QDs/MAPbI3-xClx hybrid fabricated with PbS-QDs of 4.95 nm diameter measured with lamp power 10 times at 1 V [Citation59]. Copyright from 2018 Nanoscale. (d) Schematic diagram of energy levels of the CsPbBr3:ZnO photodetectors. (e) I–V curves of CsPbBr3:ZnO photodetectors. Insets showed the zoomed-in areas. (f) Photoresponse of the CsPbBr3:ZnO devices with external bias [Citation60]. Copyright from 2017 Sol. Energ. Mat. Sol. C.
![Figure 4. (a) Photocurrent spectra of different SWCNT/PbS-QDs hybrid devices made with QDs of different sizes, showing the responses in the NIR which can be tuned by varying the PbS-QDs size. (b) Comparison between the photocurrent and the corresponding responsivity of the SWCNT/MAPbI3-xClx hybrid and of the SWCNT/PbS-QDs/MAPbI3-xClx hybrid photodetectors as a function of the illumination power of a 980 nm laser. (c) Responsivity and photoconductive gain of the SWCNT/PbS-QDs/MAPbI3-xClx hybrid fabricated with PbS-QDs of 4.95 nm diameter measured with lamp power 10 times at 1 V [Citation59]. Copyright from 2018 Nanoscale. (d) Schematic diagram of energy levels of the CsPbBr3:ZnO photodetectors. (e) I–V curves of CsPbBr3:ZnO photodetectors. Insets showed the zoomed-in areas. (f) Photoresponse of the CsPbBr3:ZnO devices with external bias [Citation60]. Copyright from 2017 Sol. Energ. Mat. Sol. C.](/cms/asset/aadfa689-51b8-41d8-9d64-ce4dae338589/tapx_a_1592709_f0004_oc.jpg)
Figure 5. (a) Schematic of the working mechanism of perovskite and carbon nanotubes composites in photodetector [Citation64]. Copyright from 2018 Organic Electronics. (b) Energy level of CsPbBr3 nanosheets and CNTs. Simplified circuit diagrams of the pristine CsPbBr3 nanosheet PDs and the hybrid PDs [Citation65]. Copyright from 2017 ACS Nano. (c) Photocurrent spectra of different SWCNT/MAPbI3−xClx hybrid devices made with various densities of SWCNT. (d) Responsivity and photoconductive gain spectra of the best device [Citation67]. Copyright from 2017 Sci. Rep. (e) The energy-level diagram of photodetector device [Citation68]. Copyright from 2018 Adv. Mater. (f) Schematic of ZnO nanofiber and perovskite hybrid photodetectors and the band energy alignment between ZnO and perovskite [Citation76]. Copyright from 2017 Nano Research.
![Figure 5. (a) Schematic of the working mechanism of perovskite and carbon nanotubes composites in photodetector [Citation64]. Copyright from 2018 Organic Electronics. (b) Energy level of CsPbBr3 nanosheets and CNTs. Simplified circuit diagrams of the pristine CsPbBr3 nanosheet PDs and the hybrid PDs [Citation65]. Copyright from 2017 ACS Nano. (c) Photocurrent spectra of different SWCNT/MAPbI3−xClx hybrid devices made with various densities of SWCNT. (d) Responsivity and photoconductive gain spectra of the best device [Citation67]. Copyright from 2017 Sci. Rep. (e) The energy-level diagram of photodetector device [Citation68]. Copyright from 2018 Adv. Mater. (f) Schematic of ZnO nanofiber and perovskite hybrid photodetectors and the band energy alignment between ZnO and perovskite [Citation76]. Copyright from 2017 Nano Research.](/cms/asset/1b268cda-6e68-4150-937e-dd0327f620fc/tapx_a_1592709_f0005_oc.jpg)
Figure 6. (a) Device structure of the perovskite photodetector, and insets showed the chemical structures of the PCBM and F4-TCNQ. (b) The energy levels distribution and the carrier transport characteristics of the device under illumination [Citation85]. Copyright from 2018 Adv. Optical Mater. (c) Schematic configuration of CH3NH3PbI3/C8BTBT heterojunction photodetector. Inset showed the molecular structure of C8BTBT. (d) Responsivity of C8BTBT, CH3NH3PbI3, and CH3NH3PbI3/C8BTBT heterojunction photodetectors as a function of the light wavelength, in which the data were measured at a bias of 10 V. The responsivity was an average value of five photodetector devices [Citation88]. Copyright from 2017 Adv. Electron. Mater. (e) The EQE spectra of devices and the schematic morphologies of the infrared absorption layer and charge carrier transportation for devices, respectively [Citation89]. Copyright from 2017 Adv. Optical Mater. (f) Schematics of MAPbI3/Fullerenes photodetector and the energy diagram of each layer in devices [Citation90]. Copyright from 2017 12th IEEE.
![Figure 6. (a) Device structure of the perovskite photodetector, and insets showed the chemical structures of the PCBM and F4-TCNQ. (b) The energy levels distribution and the carrier transport characteristics of the device under illumination [Citation85]. Copyright from 2018 Adv. Optical Mater. (c) Schematic configuration of CH3NH3PbI3/C8BTBT heterojunction photodetector. Inset showed the molecular structure of C8BTBT. (d) Responsivity of C8BTBT, CH3NH3PbI3, and CH3NH3PbI3/C8BTBT heterojunction photodetectors as a function of the light wavelength, in which the data were measured at a bias of 10 V. The responsivity was an average value of five photodetector devices [Citation88]. Copyright from 2017 Adv. Electron. Mater. (e) The EQE spectra of devices and the schematic morphologies of the infrared absorption layer and charge carrier transportation for devices, respectively [Citation89]. Copyright from 2017 Adv. Optical Mater. (f) Schematics of MAPbI3/Fullerenes photodetector and the energy diagram of each layer in devices [Citation90]. Copyright from 2017 12th IEEE.](/cms/asset/45f497af-7d12-4716-ad4b-f5b5d1ecbd24/tapx_a_1592709_f0006_oc.jpg)
Figure 7. (a) Band diagrams of the CH3NH3PbI3/Si and CH3NH3PbI3/TiO2/Si samples. (b) The dark current and light current as a function of applied voltage: the CH3NH3PbI3/Si and CH3NH3PbI3/TiO2/Si photodetectors [Citation93]. Copyright from 2018 Mater. Chem. Front. (c) I–V curves of the photodetectors with different SnO2 layer under illumination. Inset: device structure of the photodetectors (upper) and work mechanism of the heterojunction photodetectors (lower) [Citation94]. Copyright from 2018 Organic Electronics. (d) PL spectra of the CsPbBr3 and CsPbBr3/ZnO films. The inset schematically represented the charge transfer at the CsPbBr3/ZnO interface under light illumination. The band profiles and working mechanisms of (d) the CsPbBr3/ZnO heterostructure photodetector were also shown [Citation96]. Copyright from 2017 J. Mater. Chem. C.
![Figure 7. (a) Band diagrams of the CH3NH3PbI3/Si and CH3NH3PbI3/TiO2/Si samples. (b) The dark current and light current as a function of applied voltage: the CH3NH3PbI3/Si and CH3NH3PbI3/TiO2/Si photodetectors [Citation93]. Copyright from 2018 Mater. Chem. Front. (c) I–V curves of the photodetectors with different SnO2 layer under illumination. Inset: device structure of the photodetectors (upper) and work mechanism of the heterojunction photodetectors (lower) [Citation94]. Copyright from 2018 Organic Electronics. (d) PL spectra of the CsPbBr3 and CsPbBr3/ZnO films. The inset schematically represented the charge transfer at the CsPbBr3/ZnO interface under light illumination. The band profiles and working mechanisms of (d) the CsPbBr3/ZnO heterostructure photodetector were also shown [Citation96]. Copyright from 2017 J. Mater. Chem. C.](/cms/asset/059d039e-095b-43c5-b029-bc5f28627a45/tapx_a_1592709_f0007_oc.jpg)
Figure 8. (a) Schematic structure and optoelectronic characteristics of the vertical structure MoS2/CH3NH3Pb3 photodetector. The effects of drain biased and illumination on carrier transportation and separation under (b) dark and (c) under illumination based on the diagram of the MoS2/CH3NH3Pb3 vertical photoconductor [Citation80]. Copyright from 2018 Adv. Mater. Interfaces. The photoresponsivity of the planar (d) and vertical (e) photodetectors based on a 2D CH3NH3PbI3 perovskite nanosheets. Incident light: 635 nm [Citation56]. Copyright from 2017 J. Phys. D. (f) Energy diagram of the perovskite photodetector composed of ITO/PEDOT:PSS/CH3NH3PbIxCl3-x/PC60BM/Zr–TiOx/Al [Citation99]. Copyright from 2018 RSC Adv.
![Figure 8. (a) Schematic structure and optoelectronic characteristics of the vertical structure MoS2/CH3NH3Pb3 photodetector. The effects of drain biased and illumination on carrier transportation and separation under (b) dark and (c) under illumination based on the diagram of the MoS2/CH3NH3Pb3 vertical photoconductor [Citation80]. Copyright from 2018 Adv. Mater. Interfaces. The photoresponsivity of the planar (d) and vertical (e) photodetectors based on a 2D CH3NH3PbI3 perovskite nanosheets. Incident light: 635 nm [Citation56]. Copyright from 2017 J. Phys. D. (f) Energy diagram of the perovskite photodetector composed of ITO/PEDOT:PSS/CH3NH3PbIxCl3-x/PC60BM/Zr–TiOx/Al [Citation99]. Copyright from 2018 RSC Adv.](/cms/asset/d3a08b04-08ba-4a5a-8836-43a541a42724/tapx_a_1592709_f0008_oc.jpg)
Figure 9. Schematics of (left) planar and (right) vertical device for in-plane and out-of-plane transport measurements, respectively. Comparison of in-plane and out-of-plane (b) mobility and (c) electrical conductance with regard to the n in (C4H9NH3)n(CH3NH3)n-1PbnI3n+1 quasi-2D perovskite SCMs [Citation100]. Copyright from 2018 ACS Nano. (d) Schematic structure and optoelectronic characteristics of the planar structure MoS2/CH3NH3Pb3 photodetector. (e) Energy band diagram of the MoS2/CH3NH3Pb3 planar photoconductor under dark and under illumination. (f) Power-dependent peculiarity of photocurrent for planar structure. The photoexcited current increased gradually and the responsivity reduced accordingly with increasing the light intensity [Citation80]. Copyright from 2018 Adv. Mater. Interfaces.
![Figure 9. Schematics of (left) planar and (right) vertical device for in-plane and out-of-plane transport measurements, respectively. Comparison of in-plane and out-of-plane (b) mobility and (c) electrical conductance with regard to the n in (C4H9NH3)n(CH3NH3)n-1PbnI3n+1 quasi-2D perovskite SCMs [Citation100]. Copyright from 2018 ACS Nano. (d) Schematic structure and optoelectronic characteristics of the planar structure MoS2/CH3NH3Pb3 photodetector. (e) Energy band diagram of the MoS2/CH3NH3Pb3 planar photoconductor under dark and under illumination. (f) Power-dependent peculiarity of photocurrent for planar structure. The photoexcited current increased gradually and the responsivity reduced accordingly with increasing the light intensity [Citation80]. Copyright from 2018 Adv. Mater. Interfaces.](/cms/asset/72372da8-edfc-4095-ab62-b37635a16152/tapx_a_1592709_f0009_oc.jpg)
Table 1. The comparison of the key parameters for perovskite-based photodetectors.