Figures & data
Figure 1. D-Amino acids in the diet. The outer ring shows examples of natural sources of D-amino acids (D-AAs) in foods. The relative D-AA contribution as a fraction of the total AA concentration in the foods is illustrated by the thickness of the arrows. Raw meat, poultry, and fish contain small amounts of D-AA, whereas raw shellfish have a higher content of D-AA. The second ring shows examples of foods with higher D-AA after cooking, fermentation, or other processing, as colour coded in the legend and as illustrated by thicker arrows.
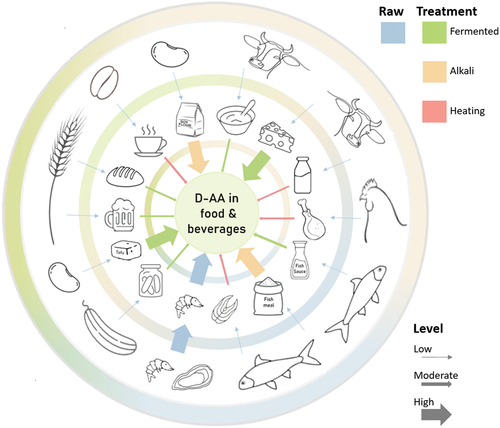
Table 1. The levels of D-alanine, D-aspartate, and D-glutamate, reported in foods and beverages.
Figure 2. Stem peptide framework of peptidoglycan in Gram-positive bacteria and cell wall components in Gram-positive and Gram-negative bacteria. Left: The peptidoglycan layer of most Gram-positive bacteria consists of linear glycan strands made of repeating disaccharide units, where MurNAc and GlcNAc are cross-linked by peptides containing L-alanine, D-glutamate, L-lysine, and D-alanine. The pentapeptide cross-links consist of glycine strands. Peptidoglycans are an integrated part of bacterial cell walls. In the middle: a cross-section of the cell wall for Gram-positive bacteria is shown; Right: a similar cross-section for Gram-negative bacteria.
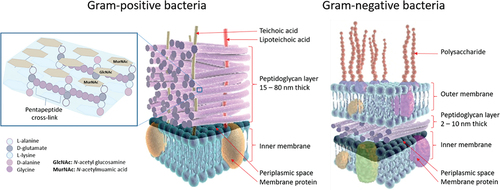
Table 2. D-Alanine and D-serine levels in human urine, plasma, and cerebrospinal fluid.
Table 3. Characteristics and function of amino acid transporters with stereospecific (re)absorption of D-amino acids in humans
Figure 4. Structure of the nephron in relation to L-and D-amino acid absorption. The cortex is depicted in the top, the outer medulla is in the middle, and the medulla is at the bottom. The figure shows the location of various nephron segments involved in D- or L-AA resorption (proximal straight tubule, proximal convoluted tubule, loop of Henle, thick ascending limb, distal convoluted tubule, connecting tubule, cortical collecting duct, and outer medullary collecting duct). D- and L-AAs are filtered through the tubule of the nephron, and about 97% of the L-AA are reabsorbed in the proximal convoluted tubule (PCT; purple colour) (S1 and S2). D-AAs, mainly D-serine, are reabsorbed in the proximal straight tubule (PST; blue colour) (S3).
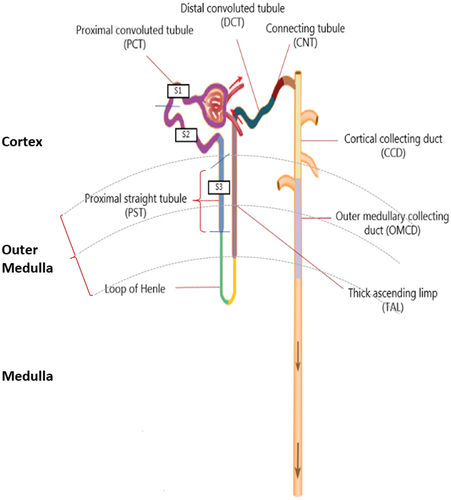
Table 4. Expression levels of D-amino acid transporters along the nephron.
Figure 5. Renal transmembrane transport of D-AAs and peptides containing one D-AA.Transporters involved in the reabsorption of D-AAs present at the proximal straight tubule (S3), the Loop of Henle, the thick ascending limp, distal convoluted tubule, cortical connecting duct, cortical collecting duct, and outer medullary collecting duct. Through the utilization of flavin adenine dinucleotide (FAD) as a cofactor, D-amino acid oxidase (DAAO) exhibits stringent stereoselectivity in facilitating the oxidative deamination of D-AAs, concomitant with the reoxidation of FADH2 via molecular oxygen, resulting in the production of hydrogen peroxide. 1. D-Aspartate, D-leucine, D-serine, D-proline, and DL- and LD-peptides are transferred through the apical membrane at the S3 mainly or solely by symporters, whereas D-leucine, D-methionine, D-phenylalanine, and D-valine are reabsorbed at the basolateral membrane by the uniporter LAT4. The known symporters involved in D-AA transport include Excitatory amino acid transporter 3 (EAAT3), Broad amino acid transporter 1 (B0AT1), Sodium- and chloride dependent transporter 3 (XTRP3), and Peptide transporter 2 (PEPT2). Peroxisomal degradation of excess D-AA by DAAO takes place between the membranes. Uniporters exchange selected D-AAs (D) for L-AAs (L) at the basolateral membrane, thereby overall favouring reuptake into the blood of D-serine, D-asparagine, D-cysteine and likely some other D-AAs. 2. Potential mechanisms for basolateral transport in the more distal part of the nephrons. D-leucine, D-methionine and D-phenylalanine are known to be reabsorbed at the basolateral membrane by LAT4. D-Alanine, and D-serine are exchanged by the antiporter Asc-1, and D-valine by both LAT4 and Asc-1.
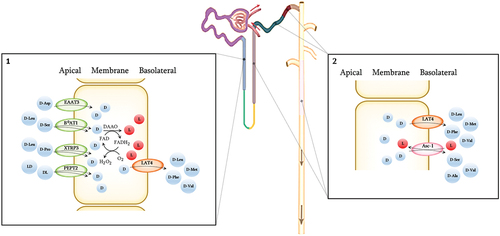
Figure 7. The NMDAR structure with its main binding sites. In addition to the binding of its agonist L-glutamate to the GluN2 unit, NMDAR signalling requires post-synaptic depolarization to remove Mg2+ blocking of the passage of cations as well as coagonistic binding of either D-serine or glycine at the GluN1. When the post-synaptic membrane is sufficiently depolarized, the NMDAR channels open, Ca2+ and Na2+ enter the neuron, and K+ exits. NMDARs may be composed of several subunits; GluN1 and GluN2A-D, as well as GluN3A-B. Functional NMDARs are di-heteromeric or tri-heteromeric receptors consisting of two GluN1 subunits or GluN1 combined with one or two different subunits, respectively.
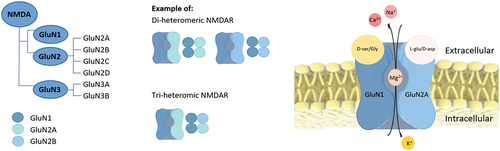
Table 5. D-Alanine and D-serine levels in serum and cerebrospinal fluid.