Figures & data
Table 1 Summarized sizes and morphologies of MnO2 NSs synthesized by different approaches
Figure 1 Schematic illustration of the single-step oxidative method with H2O2 at room temperature versus the conventional method.
Note: Reprinted with permission from Kai K, Yoshida Y, Kageyama H, et al. Room-temperature synthesis of manganese oxide monosheets. J Am Chem Soc. 2008;130(47):15938–15943.Citation25 Copyright (2008) American Chemical Society.
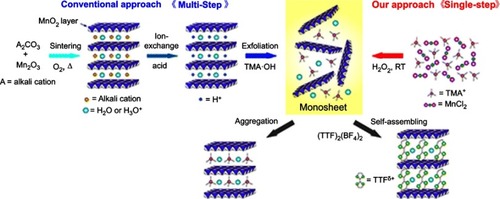
Figure 2 (A) Schematic illustration for the control experiments of reductants employed in the growth of MnO2 nanomaterials at ambient temperature (left) and TEM characterization of the corresponding products (right). Reprinted with permission from Deng R, Xie X, Vendrell M, Chang Y, Liu X. Intracellular glutathione detection using MnO(2)-nanosheet-modified upconversion nanoparticles. J Am Chem Soc. 2011;133(50):20168–20171.Citation28 Copyright 2011 American Chemical Society. (B) Schematic illustration for MnO2 NS formation based on the KMnO4 and SDS reaction. Reprinted with permission fromLiu Z, Xu K, Sun H, Yin S. One-step synthesis of single-layer MnO2 nanosheets with multi-role sodium dodecyl sulfate for highperformance pseudocapacitors. Small. 2015;11(18):2182–2191.Citation29 Copyright © 2015, John Wiley and Sons.
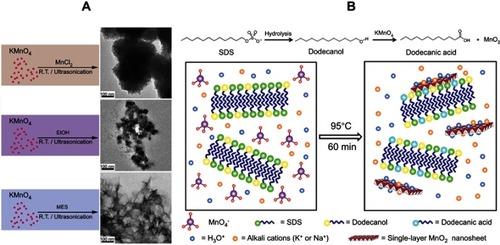
Figure 3 Schematic illustration of the diverse roles MnO2 NSs have played in the field of biomedicine.
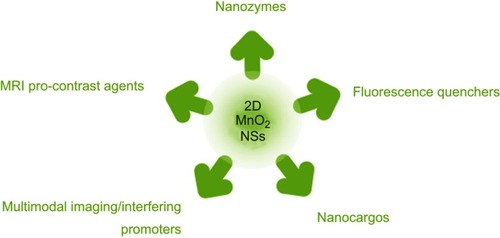
Figure 4 (A) Illustration of the MnO2 NS-based colorimetric assay for GSH quantification, where the MnO2 NSs acted as an oxidase-like nanozyme for the formation of ox-TMB and GSSG. Reprinted from Biosensors and Bioelectronics, 90, Liu J, Meng L, Fei Z, Dyson P, Jing X, Liu X. MnO nanosheets as an artificial enzyme to mimic oxidase for rapid and sensitive detection of glutathione, 69–74, Copyright (2017), with permission from Elsevier.Citation68 (B) Schematic design of the Janus protected DNA nanomachine, where miRNA-21 is employed as a model cellular RNA target (green sequence), the red X denotes DNA, PS (phosphorothioate)-DNA, 2ʹOMe (methylation)-DNA and LNA (locked nuclease acid) monomers, which are highlighted in the DNA partzymes (gray sequences). Reprinted with permission from Chen F, Bai M, Zhao Y, Cao K, Cao X, Zhao Y. MnO-nanosheet-powered protective janus DNA nanomachines supporting robust RNA imaging. Anal Chem. 2018;90(3):2271–2276.Citation69 Copyright 2018 American Chemical Society.
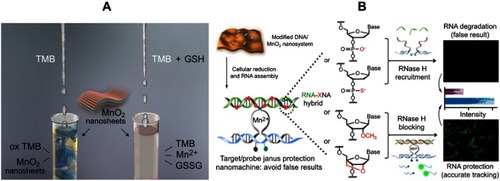
Figure 5 (A) Schematic illustration of the preparation of CDs-MnO2 NSs and the principle of the FRET-based CD-MnO2 NSs architecture for GSH sensing. Republished with permission of Royal Society of Chemistry, from A sensitive turn-on fluorescent probe for intracellular imaging of glutathione using single-layer MnO2 nanosheet-quenched fluorescent carbon quantum dots, He D, Yang X, He X, et al, 51, 79, 2015; permission conveyed through Copyright Clearence Centre, Inc.Citation170 (B) Scheme for the preparation of MnO2 NSs and the mechanism of a GQD-MnO2 NS-based optical sensing nanoplatform for monitoring GSH in MCF-7 cells. Reprinted with permission from Yan X, Song Y, Zhu C, et al. Graphene quantum dot-MnO2 nanosheet based optical sensing platform: a sensitive fluorescence“Turn Off-On” nanosensor for glutathione detection and intracellular imaging. ACS Appl Mater Interfaces. 2016;8(34):21990–21996.Citation87 Copyright 2016 American Chemical Society. (C) Schematic illustration of a g-C3N4 NS-MnO2 NS sandwich-like nanocomposite for GSH sensing. Reprinted with permission from Zhang X, Zheng C, Guo S, Li J, Yang H, Chen G. Turn-on fluorescence sensor for intracellular imaging of glutathione using g-C3N4 nanosheet-MnO2 sandwich nanocomposite. Anal Chem. 2014;86(7):3426–3434.Citation151 Copyright 2014 American Chemical Society.
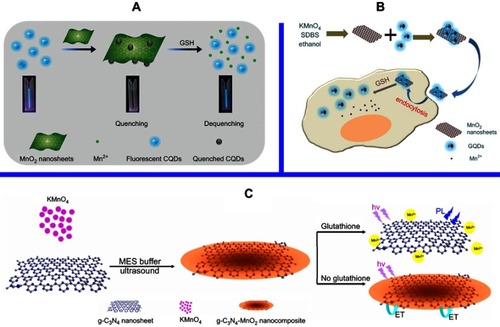
Figure 6 Schematic illustration of the MnO2 NS-mediated intracellular-hybridized chain reaction (HCR) signal amplification system for efficiently detecting miRNA-21 in living HeLa cells. The MnO2 NSs could deliver two types of hairpin DNA probes into the cytosol. Overexpressed glutathione (GSH) in HeLa cells and displacement reactions by other proteins or nucleic acids promoted the decomposition of the MnO2 NSs to release free hairpins, which assembled into double-stranded (dsDNA) polymers upon binding to the target miRNA-21. Subsequently, enhanced FRET signals were produced to realize accurate and sensitive detection. Reprinted with permission from Li J, Li D, Yuan R, Xiang Y. Biodegradable MnO2 nanosheet-mediated signal amplification in living cells enables sensitive detection of down-regulated intracellular MicroRNA. ACS Appl Mater Interfaces. 2017;9(7):5717–5724.Citation111 Copyright 2017 American Chemical Society.
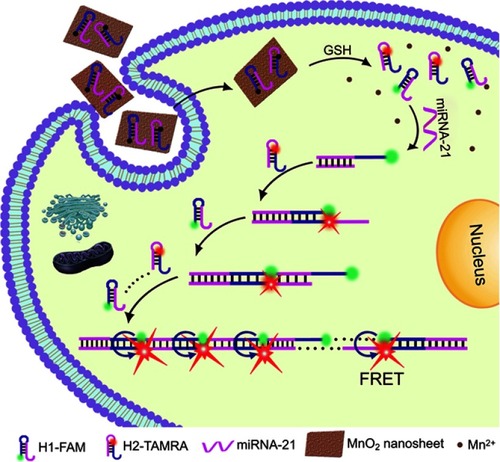
Table 2 Fluorescent biosensors based on MnO2 NSs
Figure 7 (A) Schematic illustration of the synthetic procedure for the preparation of the MSU/MnO2-CR drug delivery system. (B) Images of the MSU/MnO2-CR system before and after drug delivery under 980 nm excitation. Republished with permission of Royal Society of Chemistry, from Multifunctional MnO2 nanosheet-modified Fe3O4@SiO2/NaYF4: yb,Er nanocomposites as novel drug carriers, Zhao P, Zhu Y, Yang X, et al, 43, 2, 2014; permission conveyed through Copyright Clearence Centre, Inc.Citation138
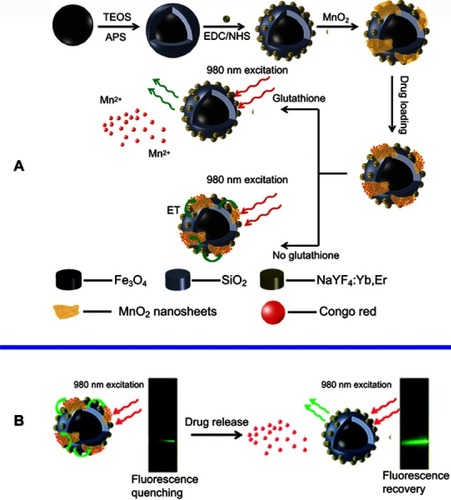
Figure 8 (A) Determination of the T1 (left) and T2 (right) relaxation rates of a MnO2 nanosheet solution (red lines) and MnO2 nanosheet solution treated with GSH (black lines). The related T1-weighted and T2-weighted MRI images were presented below. (B) Schematic illustration of the activation mechanism of the MnO2 NS-aptamer nanoprobe for fluorescence/MRI bimodal tumor cell imaging. Reprinted with permission from Zhao Z, Fan H, Zhou G, et al. Activatable fluorescence/MRI bimodal platform for tumor cell imaging via MnO2 nanosheet-aptamer nanoprobe. J Am Chem Soc. 2014;136(32):11220–11223.Citation150 Copyright 2014 American Chemical Society.
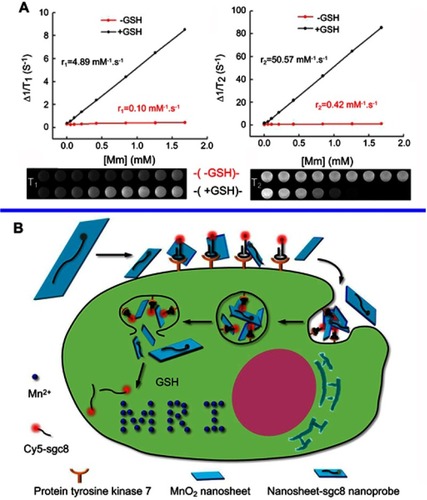
Figure 9 (A) Schematic illustration of the synthetic procedure for the PEG-MnO2 NSs. (B) Theranostic functionality of the PEG-MnO2 NSs for intracellular pH-responsive drug release and the axial and coronal T1-MRI images of 4T1 tumor-bearing nude mice before (a1, b1) and after (a2 – a9 and b2 – b9) administration of the PEG–MnO2 nanosheets within the tumor and normal subcutaneous tissue. PEG denotes ethylene glycol. Reproduced with permission from Chen Y, Ye D, Wu M, et al. Break-up of two-dimensional MnO2 nanosheets promotes ultrasensitive pH-triggered theranostics of cancer. Adv Mater Weinheim. 2014;26(41):7019–7026.Citation156 Copyright © 2014, John Wiley and Sons.
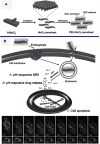
Figure 10 Schematic illustrations of the mechanism and application of mesoporous silicon (MS)@MnO2 NPs for MRI-monitored chemo-chemodynamic combination therapy. Reproduced with permission from Lin L, Song J, Song L, et al. Simultaneous fenton-like ion delivery and glutathione depletion by MnO-based nanoagent to enhance chemodynamic therapy. Angew Chem Int Ed Engl. 2018;57(18):4902–4906.Citation21 Copyright © 2018, John Wiley and Sons.
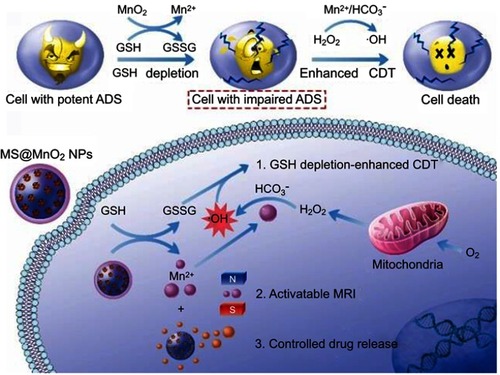
Table 3 Cytotoxicity results of various nanomaterials based on MnO2 NSs