Figures & data
Table 1 Paradigms of smart nanomedicine agents for cancer
Figure 1 (A) Schematic illustration of the pH-switchable DPP-thiophene-4-based probe for fluorescence imaging of malignant tumor. (B) TEM images of DPP-thiophene-4 at either pH 6.8 or 7.0. (C) Fluorescence imaging and corresponding signal changes of six malignant tumor-bearing mice before and after injection of DPP-thiophene-4 (10 μg/mL) at tumor tissue (red dotted cycle) and nontumor area (blue dotted cycle). Figures A to C reprinted with permission from Liu Y, Qu Z, Cao H, et al. pH switchable nanoassembly for imaging a broad range of malignant tumors. ACS Nano. 2017; 11(12):12446–12452.Citation41 Copyright © 2017, American Chemical Society. (D) Illustration of the Fe3O4-ZIF-8 assembly as pH and glutathione (GSH)-responsive T2–T1 switching magnetic resonance imaging (MRI) contrast agent. (E) Relaxivity of Fe3O4@ZIF-8 after incubation with different pH and concentrations of GSH in PBS for 3 h. (F) In vivo T1 MRI images and (G) corresponding T1 signals of 4T1 tumor-bearing mice before and after intravenous injection of Fe3O4@ZIF-8. Figures D to G are reprinted with permission from Lin J, Xin P, An L, et al. Fe3O4-ZIF-8 assemblies as pH and glutathione responsive T2–T1 switching magnetic resonance imaging contrast agent for sensitive tumor imaging in vivo. Chem Commun. 2019;55(4):478–481.Citation42 Copyright © 2019, The Royal Society of Chemistry.
Abbreviations: DPP, diketopyrrolopyrrole; ZIF, Zeolitic imidazole frameworks; GSH, glutathione.
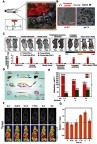
Figure 2 (A) Schematic illustration of the pH-induced one-pot fabrication of metal–organic frameworks (MOFs) with encapsulated target molecules. (B) Release profiles of doxorubicin (DOX) from DOX@ZIF-8 trigged by different pH. (C) TEM image of an MDA-MB-468 cell incubated with DOX@ZIF-8. Figures A to C reprinted with permission from Zheng H, Zhang Y, Liu L, et al. One-pot synthesis of metal–organic frameworks with encapsulated target molecules and their applications for controlled drug delivery. J Am Chem Soc. 2016;138(3):962–968.Citation47 Copyright © 2016, American Chemical Society. (D) Schematic illustration of the construction of prodrug-loaded HA/Ara-IR820@ZIF-8. (E) 1H NMR spectra of the synthesized prodrug Ara-IR820 in DMSO-d6. (F) Fluorescence imaging of the tumor-bearing mice at different times after intravenous injection with IR820, Ara-IR820@ZIF-8, and HA/Ara-IR820@ZIF-8, respectively. Figures D to F reprinted with permission from Zhang H, Li Q, Liu R, et al. A versatile prodrug strategy to in situ encapsulate drugs in MOF nanocarriers: a case of cytarabine-IR820 prodrug encapsulated ZIF-8 toward chemo-photothermal therapy. Adv Funct Mater. 2018;28(35):1802830.Citation48 Copyright © WILEY-VCH Verlag GmbH & Co. KGaA, Weinheim.
Abbreviations: DOX, doxorubicin; ZIF, Zeolitic imidazole frameworks; Ara, Cytarabine; HA , hyaluronic acid; TEM, transmission electron microscopy.
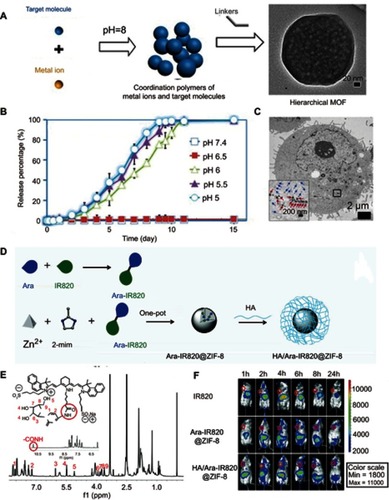
Figure 3 (A) pH-induced structural transformation of pH-sensitive magnetic nanogrenades (PMNs) and change of magnetism and photoactivity. (B) In vivo near-infrared (NIR) fluorescent imaging of HCT116 tumor-bearing mice before and after intravenous injection of different materials. Figures A and B reprinted with permission from Ling D, Park W, Park SJ, et al. Multifunctional tumor pH-sensitive self-assembled nanoparticles for bimodal imaging and treatment of resistant heterogeneous tumors. J Am Chem Soc. 2014;136(15):5647–5655.Citation54 Copyright © 2014, American Chemical Society. (C) Schematic of the structure of semiconducting polymer brush (SPB), and fabrication of SPB@POM, as well as mechanism for the acidity-triggered aggregation of SPB@POM. (D) Photoacoustic intensities of SPB and SPB@POM under different conditions. (E) Time-dependent temperature rise curves and IR thermal image (insets) for SPB@POM under different conditions. Figures C to E reprinted with permission from Yang Z, Fan W, Tang W, et al. Near-infrared semiconducting polymer brush and pH/GSH-responsive polyoxometalate cluster hybrid platform for enhanced tumor-specific phototheranostics. Angew Chem-Int Edit. 2018; 57(43):14101–14105.Citation55 Copyright © WILEY-VCH Verlag GmbH & Co. KGaA, Weinheim.
Abbreviations: GSH, glutathione; PMNs, pH sensitive magnetic nanogrenades; SPB@PON, semiconducting polymer brush and polyoxometalate cluster.
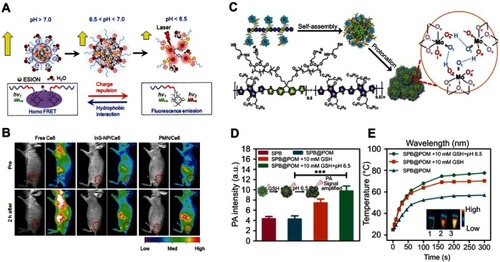
Figure 4 (A) Proposed glutathione (GSH)-mediated activation mechanism of CyA-cRGD probe. (B) Fluorescence spectra of CyA-cRGD probe with and without NEM or GSH. (C) Relative fluorescence (FL) intensity ratios of CyA-cRGD in the presence of different amino acids or metal ions. (D) Fluorescence images of CyA-cRGD in the presence of different amino acids and metal ions detected by the near-infrared (NIR) fluorescence imaging system. Figures A to D reprinted with permission from Yuan Z, Gui L, Zheng J, et al. GSH-activated light-up near-infrared fluorescent probe with high affinity to αvβ3 integrin for precise early tumor identification. ACS Appl Mater Interfaces. 2018;10(37):30994–31007.Citation63 Copyright © 2018, American Chemical Society.
Abbreviations: CYA, cyanine; RGD, a tumor-targeting unit; GSH, glutathione.
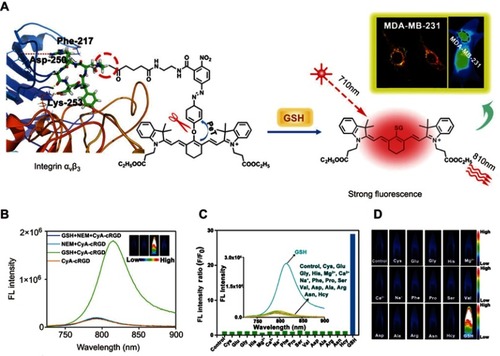
Figure 5 (A) The self-assembly and redox triggered the SN38 releasing mechanism of the OEG-2S-SN38 nanocapsule. (B) SN38 releasing curves in PBS with or without glutathione (GSH) (10 mM) at pH 7.4 or 4 at 37 °C. (C) The changes of tumor volume for different treatment groups. Figures A to C reprinted with permission from Wang J, Sun X, Mao W, et al. Tumor redox heterogeneity-responsive prodrug nanocapsules for cancer chemotherapy. Adv Mater. 2013;25(27):3670–3676.Citation68 Copyright © WILEY-VCH Verlag GmbH & Co. KGaA, Weinheim. (D) Schematic illustration of the GSH/acid-responsive polyethylene glycol (PEG)/Mn-HMSN drug carrier designed through a “manganese extraction” strategy for enhancing theranostic functions. (E) Accumulated releasing curves of Mn elements in the neutral SBF with GSH concentrations of 0, 5.0, and 10.0 mM. (F) T1-weighted magnetic resonance imaging (MRI) of tumor-bearing mice and corresponding T1 signal intensity of the tumor and liver sites before and after intravenous injection of PEG/Mn-HMSNs with a dose of 5 mg/kg. (G) Tumor-growth inhibition effect for different treatment groups. Figures D to G reprinted with permission from Yu L, Chen Y, Wu M, et al. “Manganese extraction” strategy enables tumor-sensitive biodegradability and theranostics of nanoparticles. J Am Chem Soc. 2016; 138(31):9881–9894.Citation69 Copyright © 2016, American Chemical Society.
Abbreviations: DOX, doxorubicin; i.v., intravenous; OEG, oligo(ethylene glycol); SN38, 7-ethyl-10-hydroxyl-camptothecin; PBS, phosphate-buffered saline; GSH, glutathione; HMSNs, Hollow mesoporous silica nanoparticals; PEG, polyethylene glycol; MR, magnetic resonance.
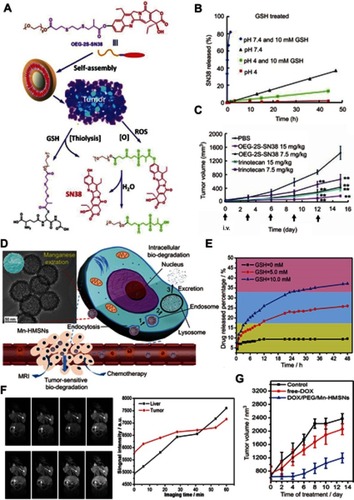
Figure 6 (A) Schematic illustration of the fabrication of the glutathione (GSH)-responsive MnMoOX nanorod for tumor theranostic application. (B) Ultraviolet–visible absorption spectra and (C) PA signal intensities of MnMoOX-PEG after incubation with different concentrations of GSH. (D) T1 relaxation rates of different concentrations of MnMoOX-PEG before and after incubation with 10 mM GSH. (E) In vivo photoacoustic imaging of tumors, and (F) T1-weighted magnetic resonance imaging (MRI) of tumor-bearing mice and corresponding T1 signal intensity of the tumor sites before and after intravenous injection of MnMoOX-PEG. (G) Tumor growth curves of mice for different treatment groups. Figures A to G reprinted with permission from Gong F, Cheng L, Yang N, et al. Bimetallic oxide MnMoOX nanorods for in vivo photoacoustic imaging of GSH and tumorspecific photothermal therapy. Nano Lett. 2018;18(9):6037–6044.Citation75 Copyright © 2018, American Chemical Society.
Abbreviations: i.v., intravenous; PEG, polyethylene glycol; PTT, photothermal therapy; GSH, glutathione; PA, photoacoustic.
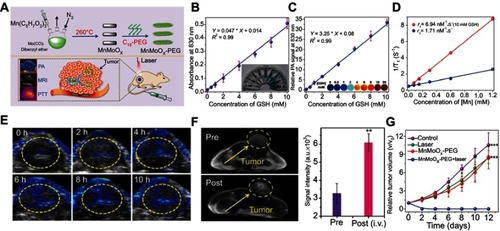
Figure 7 (A) Schematic illustration of the redox-trigged disassembly of PEG-PUSeSe-PEG micelles. (B) Cryo-TEM image of PEG-PUSeSe-PEG micelles. Releasing profiles of rhodamine B from PEG-PUSeSe-PEG micelles triggered by (C) H2O2 and (D) glutathione. Reproduced with permission. Figures A to D reprinted with permission from Ma N, Li Y, Xu H, et al. Dual redox responsive assemblies formed from diselenide block copolymers. J Am Chem Soc. 2010; 132(2):442–443.Citation86 Copyright 2010, American Chemical Society. Copyright © 2010, American Chemical Society. (E) Schematic illustration of the synthetic procedure and H2O2-triggered drug release from Ag@MSN carrier. (F) Absorbance spectra of Ag@MSNs in the presence of various concentrations of H2O2. (G) Releasing profiles of ibuprofen from Ag@IBU@MSNs in the presence and absence of H2O2. (H) Growth profiles of bacteria in LB media with different concentrations of Ag@MSNs. Figures E to H reprinted with permission from Muhammad F, Wang A, Miao L, et al. Synthesis of oxidant prone nanosilver to develop H2O2 responsive drug delivery system. Langmuir. 2015;31(1):514–521.Citation87 Copyright © 2014, American Chemical Society.
Abbreviations: PEG, polyethylene glycol; PU, polyurethane; Se-Se, diselenide bonds; MSNs, mesoporous silica nanoparticals.
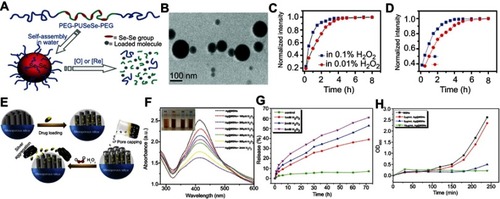
Figure 8 (A) Schematic illustration of the mechanism of H2O2-triggered O2 generation and photosensitizer release for enhanced photodynamic therapy (PDT). (B) Releasing curves for MB from HAOP nanoparticles (NPs) with and without H2O2 (100 μM). (C) Tumor growth curves of mice upon different treatments. (D) H&E staining of tumors for different groups at 24 h post treatment. Figures A to D reprinted with permission from Chen H, Tian J, He W, et al. H2O2-activatable and O2-evolving nanoparticles for highly efficient and selective photodynamic therapy against hypoxic tumor cells. J Am Chem Soc. 2015;137(4):1539–1547.Citation92 Copyright © 2015, American Chemical Society. (E) Schematic illustration of the fabrication of PCN-224-Pt. (F) Ultraviolet–visible spectra of remainding H2O2 after catalysis by PCN-224-Pt for different times at pH 7.4. (G) Degradation rates of DPBF after treatment with PCN-224 or PCN-224-Pt in the absence and presence of H2O2 under light irradiation in a N2 atmosphere at pH 7.4. (H) Photographs of mice bearing H22 tumor before and on day 14 after various treatments. (I) Relative tumor volume for different treatment groups. Figures E to I reprinted with permission from Zhang Y, Wang F, Liu C, et al. Nanozyme decorated metal–organic frameworks for enhanced photodynamic therapy. ACS Nano. 2018;12(1):651–661.Citation93 Copyright © 2018, American Chemical Society.
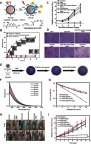
Figure 9 (A) Schematic illustration of H2O2-triggered surface oxidation of FeS2-PEG with the generation of OH. (B) MB decolorization for investigating the efficiency of the Fenton reaction under different experimental conditions. (C) Temperature curve upon irradiation by different laser intensities. (D) Relative tumor volume change after different treatments. Figures A to D reprinted with permission from Tang Z, Zhang H, Liu Y, et al. Antiferromagnetic pyrite as the tumor microenvironment-mediated nanoplatform for self-enhanced tumor imaging and therapy. Adv Mater. 2017;29(47):1701683.Citation96 Copyright © WILEY-VCH Verlag GmbH & Co. KGaA, Weinheim. (E) Mechanism of the photoacoustic imaging guiding photothermal-enhanced chemodynamic therapy. (F) MB absorbance for evaluating the efficiency of Fenton-like reaction under various conditions. (G) ESR spectra with different experimental conditions. (H) Change of relative tumor volume after different treatments. Figures E to H reprinted with permission from Liu P, Wang Y, An L, et al. Ultrasmall WO3–x@γ-poly-l-glutamic acid nanoparticles as a photoacoustic imaging and effective photothermal-enhanced chemodynamic therapy agent for cancer. ACS Appl Mater Interfaces. 2018;10(45):38833–38844.Citation98 Copyright © 2018, American Chemical Society.
Abbreviations: CDT, chemodynamic therapy; NIR, near-infrared; NP, nanoparticle; PEG, polythylene glycol; MB, Methylene blue; ESR, Electron spin resonance.
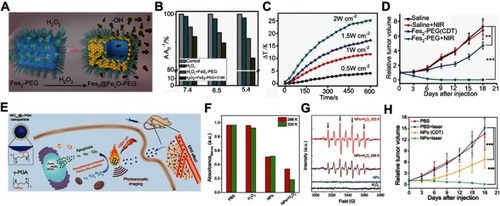
Figure 10 (A) Schematic illustration of the design of the ratiometric NIR-II fluorescence nanoprobe (NIR-II@Si) and its H2S-responsive mechanism. (B) Fluorescence (FL) spectra of NIR-II@Si in PBS (pH 7.4) before and after addition of 100 μm NaHS. (C) Time-dependent fluorescence spectra of NIR-II@Si under the presence of 100 μm NaHS. (D) In vivo fluorescence imaging of different tumor-bearing mice using an H2S-activated NIR-II@Si nanoprobe. Figures A to D reprinted with permission from Xu G, Yan Q, Lv X, et al. Imaging of colorectal cancers using activatable nanoprobes with second near-infrared window emission. Angew Chem-Int Edit. 2018;57(14):3626–3630.Citation110 Copyright © WILEY-VCH Verlag GmbH & Co. KGaA, Weinheim.
Abbreviations: NIR, near-infrared; PEG, polythylene. glycol.
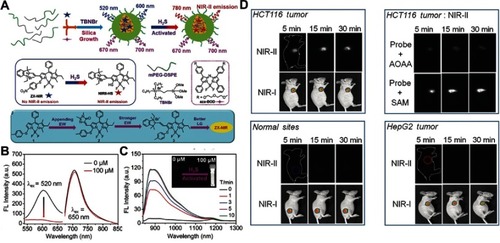
Figure 11 (A) Simple structure of copper–zinc mixed metal–organic framework NP-1 and proposed H2S-activated 1O2 generation for cancer therapy. (B) Fluorescence spectra of NP-1 after reaction with different concentrations of NaHS. (C) The linear releationship between the fluorescence intensity of NP-1 and the added concentration of NaHS. (D) Relative tumor volume change after different treatments. Figures A to D reprinted with permission from Ma Y, Li X, Li A, et al. H2S-activable MOF nanoparticle photosensitizer for effective photodynamic therapy against cancer with controllable singlet-oxygen release. Angew Chem-Int Edit. 2017;56(44):13752–13756.Citation116 Copyright © WILEY-VCH Verlag GmbH & Co. KGaA, Weinheim. (E) Schematic illustration of in situ reaction between endogenous H2S and Cu2O for activating photoacoustic imaging and photothermal therapy (PTT). (F) In vivo photoacoustic imaging and (G) corresponding signal intensity of tumor-bearing mice for different groups. (H) Time-varying relative tumor volume after different treatments. Figures E to H reprinted with permission from An L, Wang X, Rui X, et al. The in situ sulfidation of Cu2O by endogenous H2S for colon cancer theranostics. Angew Chem-Int Edit. 2018;57(48):15782–15786.Citation117 Copyright © WILEY-VCH Verlag GmbH & Co. KGaA, Weinheim.
Abbreviations: NP, nanoparticle; ZnTcpp, zinc metalated porphyrin.
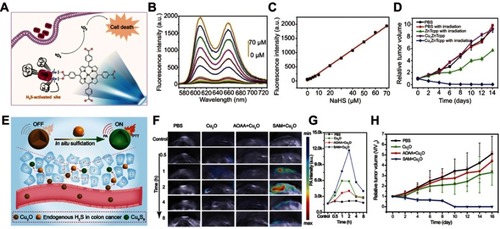