Figures & data
Figure 1 (A) Synthetic route and chemical structure of P1; (B) self-assembly of P1 in water. Reprinted with permission from Yu GC, Ma YJ, Han CY, et al. A sugar-functionalized amphiphilic pillar[5]arene: synthesis, self-assembly in water, and application in bacterial cell agglutination. J Am Chem Soc. 2013;135:10310–10313.Citation48; Copyright 2013, American Chemical Society.
![Figure 1 (A) Synthetic route and chemical structure of P1; (B) self-assembly of P1 in water. Reprinted with permission from Yu GC, Ma YJ, Han CY, et al. A sugar-functionalized amphiphilic pillar[5]arene: synthesis, self-assembly in water, and application in bacterial cell agglutination. J Am Chem Soc. 2013;135:10310–10313.Citation48; Copyright 2013, American Chemical Society.](/cms/asset/d1997e6f-698d-4d1f-b6da-677a856422fa/dijn_a_12194695_f0001_c.jpg)
Figure 2 Chemical structures of WP5 and polymer 1, as well as schematic illustration of the redox-responsive self-assembly between WP5 and 1 in water.
Notes: Reprinted with permission from Chi XD, Yu GC, Ji XF, et al. Redox-responsive amphiphilic macromolecular [2] pseudorotaxane constructed from a water-soluble pillar[5]arene and a paraquat-containing homopolymer. ACS Macro Lett. 2015;4:996–999.Citation55; Copyright 2015, American Chemical Society.
![Figure 2 Chemical structures of WP5 and polymer 1, as well as schematic illustration of the redox-responsive self-assembly between WP5 and 1 in water.Notes: Reprinted with permission from Chi XD, Yu GC, Ji XF, et al. Redox-responsive amphiphilic macromolecular [2] pseudorotaxane constructed from a water-soluble pillar[5]arene and a paraquat-containing homopolymer. ACS Macro Lett. 2015;4:996–999.Citation55; Copyright 2015, American Chemical Society.](/cms/asset/58b67b89-fdc1-44b5-842a-d010cb6058e4/dijn_a_12194695_f0002_c.jpg)
Figure 3 Synthetic route to WP6.
Notes: Reprinted with permission from Yu GC, Xue M, Zhang ZB, et al. A water-soluble pillar[6]arene: synthesis, host−guest chemistry, and its application in dispersion of multiwalled carbon nanotubes in water. J Am Chem Soc. 2012;134:13248–13251.Citation38; Copyright 2012, American Chemical Society.
![Figure 3 Synthetic route to WP6.Notes: Reprinted with permission from Yu GC, Xue M, Zhang ZB, et al. A water-soluble pillar[6]arene: synthesis, host−guest chemistry, and its application in dispersion of multiwalled carbon nanotubes in water. J Am Chem Soc. 2012;134:13248–13251.Citation38; Copyright 2012, American Chemical Society.](/cms/asset/75d5cb3d-547a-4b59-af81-16ce69ffb757/dijn_a_12194695_f0003_b.jpg)
Figure 4 (A) Chemical structures and cartoon representations of WP5, and guest molecules G and GH2; (B) Cartoon representation of the self-assembly of GH2, (WP5)2⊃GH2, and the controlled behavior.
Notes: Reprinted with permission from Zhou YJ, Li ER, Zhao R, Jie KC. CO2-enhanced bola-type supramolecular amphiphile constructed from pillar[5]arene-based host−guest recognition. Org Lett. 2018;20:4888–4892.Citation77; Copyright 2018, American Chemical Society.
![Figure 4 (A) Chemical structures and cartoon representations of WP5, and guest molecules G and GH2; (B) Cartoon representation of the self-assembly of GH2, (WP5)2⊃GH2, and the controlled behavior.Notes: Reprinted with permission from Zhou YJ, Li ER, Zhao R, Jie KC. CO2-enhanced bola-type supramolecular amphiphile constructed from pillar[5]arene-based host−guest recognition. Org Lett. 2018;20:4888–4892.Citation77; Copyright 2018, American Chemical Society.](/cms/asset/c43bbe51-8bc9-45d9-9c1f-9e54f9fa0d94/dijn_a_12194695_f0004_c.jpg)
Figure 5 Synthetic route to phosphonated pillar[5]arene (PPA[5]).
Notes: Reprinted with permission from Huang X, Wu SS, Ke XK, Li XY, Du XZ. Phosphonated pillar[5]arene-valved mesoporous silica drug delivery systems. ACS Appl Mater Interfaces. 2017;9:19638–19645.Citation30; Copyright 2017, American Chemical Society.
![Figure 5 Synthetic route to phosphonated pillar[5]arene (PPA[5]).Notes: Reprinted with permission from Huang X, Wu SS, Ke XK, Li XY, Du XZ. Phosphonated pillar[5]arene-valved mesoporous silica drug delivery systems. ACS Appl Mater Interfaces. 2017;9:19638–19645.Citation30; Copyright 2017, American Chemical Society.](/cms/asset/7d601c38-39e9-4a16-baa0-e02de5010929/dijn_a_12194695_f0005_b.jpg)
Figure 6 (A) Charge reversal process of P5NH-DCA; (B) Binding to cancer cell membrane; (C) Disrupting cancer cell membrane; (D) Killing cancer cells.
Notes: Reprinted with permission from Chang YC, Chen JY, Yang JP, et al. Targeting the cell membrane by charge-reversal amphiphilic pillar[5]arene for the selective killing of cancer cells. ACS Appl Mater Interfaces. 2019;11:38497–38502.Citation79; Copyright 2019, American Chemical Society.
![Figure 6 (A) Charge reversal process of P5NH-DCA; (B) Binding to cancer cell membrane; (C) Disrupting cancer cell membrane; (D) Killing cancer cells.Notes: Reprinted with permission from Chang YC, Chen JY, Yang JP, et al. Targeting the cell membrane by charge-reversal amphiphilic pillar[5]arene for the selective killing of cancer cells. ACS Appl Mater Interfaces. 2019;11:38497–38502.Citation79; Copyright 2019, American Chemical Society.](/cms/asset/2cd64bcd-5dc1-4684-8664-3e8cd829be1b/dijn_a_12194695_f0006_c.jpg)
Figure 7 Chemical structures and cartoons of DSP5 and TPE-Q4 and the schematic presentation of their self-assembly into a fluorescent supramolecular system for the selective detection of Fe3+ ions.
Notes: Reprinted with permission from Wang X, Lou XY, Jin XY, Liang F, Yang YW. A binary supramolecular assembly with intense fluorescence emission, high pH stability, and cation selectivity: supramolecular assembly-induced emission materials. Research. 2019;2019:1454562.Citation81; Copyright 2019, Science.
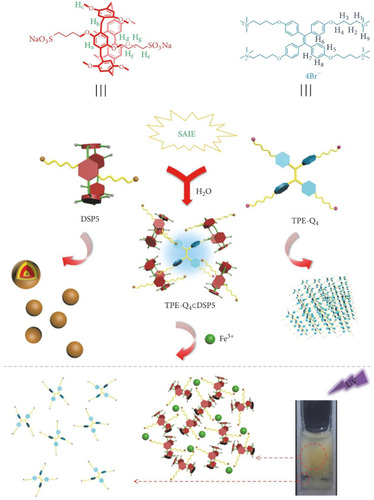
Figure 8 Synthetic route of tetra-alkylphosphine capped amphiphilic pillar[5]arene 1.
Notes: Reprinted with permission from Ogoshi T, Ueshima N, Yamagishi TA. An amphiphilic pillar[5]arene as efficient and substrate-selective phase-transfer catalyst. Org Lett. 2013;15:3742–3745.Citation82; Copyright 2019, American Chemical Society.
![Figure 8 Synthetic route of tetra-alkylphosphine capped amphiphilic pillar[5]arene 1.Notes: Reprinted with permission from Ogoshi T, Ueshima N, Yamagishi TA. An amphiphilic pillar[5]arene as efficient and substrate-selective phase-transfer catalyst. Org Lett. 2013;15:3742–3745.Citation82; Copyright 2019, American Chemical Society.](/cms/asset/43d62891-76c6-41e1-8e00-bc9c8bdce2df/dijn_a_12194695_f0008_b.jpg)
Figure 9 (A) Chemical structures and cartoon representations of 1, 2, and SDS and (B) Cartoon representation of gas-controlled self-assembly and dual-triggered release of calcein.
Notes: Reprinted with permission from Jie KC, Zhou YJ, Yao Y, Shi BB, Huang FH. CO2-responsive pillar[5]arene-based molecular recognition in water: establishment and application in gas-controlled self-assembly and release. J Am Chem Soc. 2015;137:10472–10475.Citation37; Copyright 2015, American Chemical Society.
![Figure 9 (A) Chemical structures and cartoon representations of 1, 2, and SDS and (B) Cartoon representation of gas-controlled self-assembly and dual-triggered release of calcein.Notes: Reprinted with permission from Jie KC, Zhou YJ, Yao Y, Shi BB, Huang FH. CO2-responsive pillar[5]arene-based molecular recognition in water: establishment and application in gas-controlled self-assembly and release. J Am Chem Soc. 2015;137:10472–10475.Citation37; Copyright 2015, American Chemical Society.](/cms/asset/906ab7d2-aa6c-4055-91e5-b809083a8327/dijn_a_12194695_f0009_c.jpg)
Figure 10 Schematic illustration of the formation of supramolecular vesicles and their pH-responsive drug release.
Notes: Reprinted with permission from Duan QP, Cao Y, Li Y, et al. pH-responsive supramolecular vesicles based on water-soluble pillar[6]arene and ferrocene derivative for drug delivery. J Am Chem Soc. 2013;135:10542–10549.Citation63; Copyright 2013, American Chemical Society.
![Figure 10 Schematic illustration of the formation of supramolecular vesicles and their pH-responsive drug release.Notes: Reprinted with permission from Duan QP, Cao Y, Li Y, et al. pH-responsive supramolecular vesicles based on water-soluble pillar[6]arene and ferrocene derivative for drug delivery. J Am Chem Soc. 2013;135:10542–10549.Citation63; Copyright 2013, American Chemical Society.](/cms/asset/cfe52905-566d-41f4-bd64-1685ba0bd13c/dijn_a_12194695_f0010_c.jpg)
Figure 11 Schematic illustration of the construction of supramolecular micelles (WP6⊃G1) or vesicles (WP6⊃G2) and the application of supramolecular vesicles in drug delivery.
Notes: Reprinted with permission from Hu XY, Jia KK, Cao Y, et al. Dual photo- and pH-responsive supramolecular nanocarriers based on water-soluble pillar[6]arene and different azobenzene derivatives for intracellular anticancer drug delivery. Chem Eur J. 2015;21:1208–1220.Citation64; © 2015 WILEY‐VCH Verlag GmbH & Co. KGaA, Weinheim.
![Figure 11 Schematic illustration of the construction of supramolecular micelles (WP6⊃G1) or vesicles (WP6⊃G2) and the application of supramolecular vesicles in drug delivery.Notes: Reprinted with permission from Hu XY, Jia KK, Cao Y, et al. Dual photo- and pH-responsive supramolecular nanocarriers based on water-soluble pillar[6]arene and different azobenzene derivatives for intracellular anticancer drug delivery. Chem Eur J. 2015;21:1208–1220.Citation64; © 2015 WILEY‐VCH Verlag GmbH & Co. KGaA, Weinheim.](/cms/asset/ab4db06e-29a1-4715-aa3b-d923a28c24f6/dijn_a_12194695_f0011_c.jpg)
Figure 12 Schematic illustration of the glucose-responsive supramolecular insulin delivery system. (A) Chemical structure and the mechanism of multiresponsive diphenylboronic acid guest G. (B) Supramolecular self-assembly of the host–guest complex WP5⊃G into vesicles and their successful encapsulation of insulin and GOx as well as the efficient insulin release under hyperglycemic state.
Notes: Reprinted with permission from Zuo MZ, Qian WR, Xu ZQ, et al. Multiresponsive supramolecular theranostic nanoplatform based on pillar[5]arene and Diphenylboronic acid derivatives for integrated glucose sensing and insulin delivery. Small. 2018;14:e1801942.Citation91; © 2018 WILEY‐VCH Verlag GmbH & Co. KGaA, Weinheim.
![Figure 12 Schematic illustration of the glucose-responsive supramolecular insulin delivery system. (A) Chemical structure and the mechanism of multiresponsive diphenylboronic acid guest G. (B) Supramolecular self-assembly of the host–guest complex WP5⊃G into vesicles and their successful encapsulation of insulin and GOx as well as the efficient insulin release under hyperglycemic state.Notes: Reprinted with permission from Zuo MZ, Qian WR, Xu ZQ, et al. Multiresponsive supramolecular theranostic nanoplatform based on pillar[5]arene and Diphenylboronic acid derivatives for integrated glucose sensing and insulin delivery. Small. 2018;14:e1801942.Citation91; © 2018 WILEY‐VCH Verlag GmbH & Co. KGaA, Weinheim.](/cms/asset/608bc052-d854-4056-a813-544b3f92ee5e/dijn_a_12194695_f0012_c.jpg)
Figure 13 Chemical structures of G1, G2, and G3, as well as illustrations of the controlled drug release in response to the five stimuli.
Notes: Reprinted with permission from Jiang L, Huang X, Chen D, et al. Supramolecular vesicles coassembled from disulfide-linked benzimidazolium amphiphiles and carboxylate-substituted pillar-[6]arenes that are responsive to five stimuli. Angew Chem Int Ed. 2017;56:2655–2659.Citation73; © 2017 Wiley‐VCH Verlag GmbH & Co. KGaA, Weinheim.
![Figure 13 Chemical structures of G1, G2, and G3, as well as illustrations of the controlled drug release in response to the five stimuli.Notes: Reprinted with permission from Jiang L, Huang X, Chen D, et al. Supramolecular vesicles coassembled from disulfide-linked benzimidazolium amphiphiles and carboxylate-substituted pillar-[6]arenes that are responsive to five stimuli. Angew Chem Int Ed. 2017;56:2655–2659.Citation73; © 2017 Wiley‐VCH Verlag GmbH & Co. KGaA, Weinheim.](/cms/asset/86610dc0-0720-47f4-bb77-b8e2587e9c65/dijn_a_12194695_f0013_c.jpg)
Figure 14 Cartoon representation of constructing dual-target supramolecular vesicles and their properties in efficient drug delivery.
Notes: Reprinted with permission from Shang K, Wang Y, Lu YC, Pei ZC, Pei YX. Dual-targeted supramolecular vesicles based on the complex of galactose capped pillar[5]arene and triphenylphosphonium derivative for drug delivery. Isr J Chem. 2018;58:1205–1209.Citation49; © 2018 Wiley‐VCH Verlag GmbH & Co. KGaA, Weinheim.
![Figure 14 Cartoon representation of constructing dual-target supramolecular vesicles and their properties in efficient drug delivery.Notes: Reprinted with permission from Shang K, Wang Y, Lu YC, Pei ZC, Pei YX. Dual-targeted supramolecular vesicles based on the complex of galactose capped pillar[5]arene and triphenylphosphonium derivative for drug delivery. Isr J Chem. 2018;58:1205–1209.Citation49; © 2018 Wiley‐VCH Verlag GmbH & Co. KGaA, Weinheim.](/cms/asset/d34fe028-f1ea-41e2-bcc2-41ae893ce73c/dijn_a_12194695_f0014_c.jpg)
Figure 15 Schematic illustration of the synthesis of CAAP5, the host-guest complexation with galactose derivatives (G), formation of vesicles (CAAP5G), and their GSH/pH dual-responsive drug release.
Notes: Reprinted with permission from Lu YC, Hou CX, Ren JL, et al. A multifunctional supramolecular vesicle based on complex of cystamine dihydrochloride capped pillar[5]arene and galactose derivative for targeted drug delivery. Int J Nanomed. 2019;14:3525–3532.Citation90; Copyright 2019, Dovepress.
![Figure 15 Schematic illustration of the synthesis of CAAP5, the host-guest complexation with galactose derivatives (G), formation of vesicles (CAAP5G), and their GSH/pH dual-responsive drug release.Notes: Reprinted with permission from Lu YC, Hou CX, Ren JL, et al. A multifunctional supramolecular vesicle based on complex of cystamine dihydrochloride capped pillar[5]arene and galactose derivative for targeted drug delivery. Int J Nanomed. 2019;14:3525–3532.Citation90; Copyright 2019, Dovepress.](/cms/asset/8d980c01-df5f-4609-92f1-f892c9485af8/dijn_a_12194695_f0015_c.jpg)
Figure 16 Cartoon of the self-assembly and drug-loading process of a vesicle based on Trp-modified pillar[5]arene and a galactose derivative and its possible cellular pathways.
Notes: Reprinted with permission from Yang K, Chang YC, Wen J, et al. Supramolecular vesicles based on complex of Trp-modified pillar[5]arene and galactose derivative for synergistic and targeted drug delivery. Chem Mater. 2016;28:1990–1993.Citation89; Copyright 2016, American Chemical Society.
![Figure 16 Cartoon of the self-assembly and drug-loading process of a vesicle based on Trp-modified pillar[5]arene and a galactose derivative and its possible cellular pathways.Notes: Reprinted with permission from Yang K, Chang YC, Wen J, et al. Supramolecular vesicles based on complex of Trp-modified pillar[5]arene and galactose derivative for synergistic and targeted drug delivery. Chem Mater. 2016;28:1990–1993.Citation89; Copyright 2016, American Chemical Society.](/cms/asset/58349811-6844-4d64-8ead-57f471087d41/dijn_a_12194695_f0016_c.jpg)
Figure 17 Schematic design of the drug–drug conjugate SVs for co-delivery of different anticancer drugs. Reprinted with permission from Shao W, Liu X, Sun GP, Hu XY, Zhu JJ, Wang LY. Construction of drug-drug conjugate supramolecular nanocarriers based on water-soluble pillar[6]arene for combination chemotherapy. Chem Commun. 2018;54:9462–9465.Citation43; Copyright 2018, Royal Society of Chemistry.
![Figure 17 Schematic design of the drug–drug conjugate SVs for co-delivery of different anticancer drugs. Reprinted with permission from Shao W, Liu X, Sun GP, Hu XY, Zhu JJ, Wang LY. Construction of drug-drug conjugate supramolecular nanocarriers based on water-soluble pillar[6]arene for combination chemotherapy. Chem Commun. 2018;54:9462–9465.Citation43; Copyright 2018, Royal Society of Chemistry.](/cms/asset/41abf91b-2181-4002-9c84-34dcd4e67d3a/dijn_a_12194695_f0017_c.jpg)
Figure 18 Construction of multifunctional supramolecular vesicles for combination cancer therapy.
Notes: Reprinted with permission from Wang Q, Tian L, Xu JZ, et al. Multifunctional supramolecular vesicles for combined photothermal/photodynamic/hypoxia-activated chemotherapy. Chem Commun. 2018;54:10328–10331.Citation74; Copyright 2018, Royal Society of Chemistry.
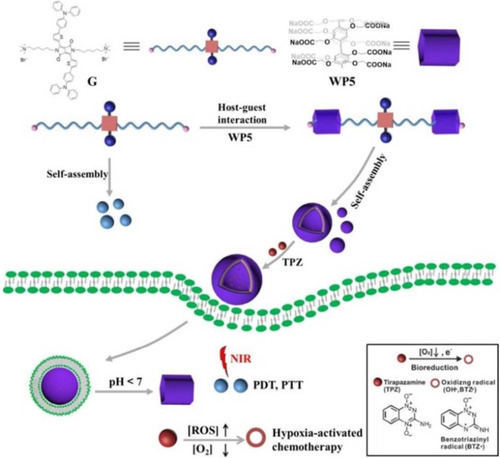
Figure 19 Synthetic route of ferrocenium capped amphiphilic PA[5], Illustration of the formation of cationic vesicles, and their redox-responsive drug/siRNA release.
Notes: Reprinted with permission from Chang YC, Yang K, Wei P, et al. Cationic vesicles based on amphiphilic pillar[5]arene capped with ferrocenium: a redox-responsive system for drug/siRNA co-delivery. Angew Chem Int Ed. 2014;53:13126–13130.Citation88; © 2014 WILEY‐VCH Verlag GmbH & Co. KGaA, Weinheim.
![Figure 19 Synthetic route of ferrocenium capped amphiphilic PA[5], Illustration of the formation of cationic vesicles, and their redox-responsive drug/siRNA release.Notes: Reprinted with permission from Chang YC, Yang K, Wei P, et al. Cationic vesicles based on amphiphilic pillar[5]arene capped with ferrocenium: a redox-responsive system for drug/siRNA co-delivery. Angew Chem Int Ed. 2014;53:13126–13130.Citation88; © 2014 WILEY‐VCH Verlag GmbH & Co. KGaA, Weinheim.](/cms/asset/aae206ce-ed66-4f25-ad1c-14717123b966/dijn_a_12194695_f0020_c.jpg)