Figures & data
Figure 1 The physiological process of normal wound and diabetic wound. Unlike normal wounds, diabetic wounds are characterized by impaired angiogenesis, excessive inflammatory macrophages. Excessive production of matrix metalloproteinases (MMPs) at the wound site, and hyperglycemia leads to an increase ROS that prevent the formation of healthy tissue.
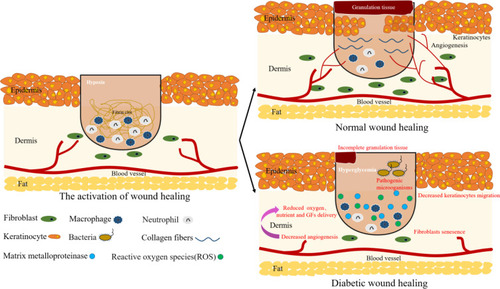
Figure 2 Schematic illustration of the categories of biomaterials used on diabetic wounds. Biomaterials are loaded with bioactive molecules (including GFs, genes/proteins/peptides, stem cells/exosomes, etc.) and non-bioactive substances (including metal ions, oxygen, etc.) to promote diabetic wound healing.
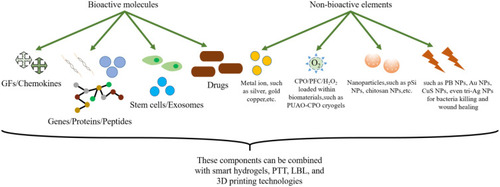
Table 1 Growth Factors in Nanoparticles/Hydrogels/Scaffolds Used in Experimental Diabetic Wound Healing Studies
Figure 3 Changes in GFs in diabetic wounds. (A) Changes in growth factors in diabetic wounds and their effects on angiogenesis and ECM. (B) Hyperglycemia leads to the production of oxygen free radicals.
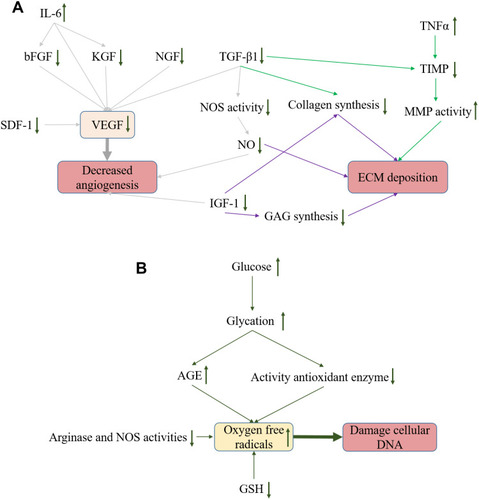
Table 2 Genes/Proteins/Peptides Used in Diabetic Wound Healing are Summarized. Most of the Genes are miRNA, and There are Both Natural and Synthetic Peptides
Table 3 Summary of Stem Cell/Exosomes Towards Effective Control of Diabetic Wounds
Table 4 Delivery of Drug/Natural Macromolecular Bioactive Substances Systems with Effective Control of Chronic/Diabetic Wounds
Figure 4 Development of a transdermal drug delivery system for DFO. (A) DFO patch is administered through transdermal drug delivery system into the dermis to perform its functions. (B) Functional diagram of DFO and its regulation in the HIF-1a signaling pathway.
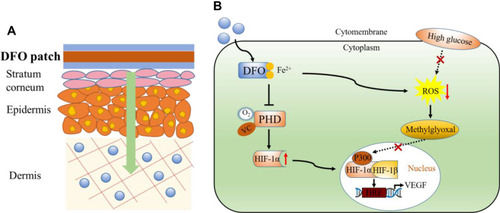
Table 5 Metallic and Metal Oxide Nanomaterials are Used in Diabetic Wound Repair
Table 6 Summary of Current O2 Delivery Systems
Table 7 Other Approaches for Diabetic Wound Repair
Figure 6 Schematic diagram of BSA-CuS antibacterial therapy. Reprinted with permission from Zhao Y, Cai Q, Qi W, et al. BSA-CuS nanoparticles for photother- mal therapy of diabetic wound infection in vivo. Biol Chem ChemBiol. 2018;3:9510–9516. Copyright 2018, John Wiley and Sons.Citation213 (A) HRTEM image of the BSA-CuS nanoparticles. (B) Schematic illustration of BSA-CuS nanoparticles as photothermal agent for photothermal antibacterial therapy in vitro and in vivo.
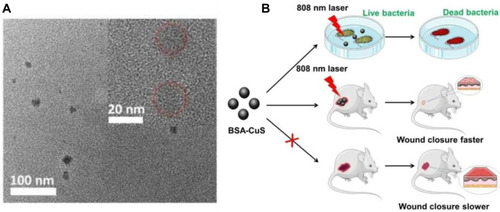
Table 8 To Summarize the Role of Multifunctional Hydrogel in Diabetic Wound Healing
Figure 7 Schematic diagram of hydrogel synthesis. (A) Scheme of poly-carboxybetaine (PCB) hydrogel dressing for the detection of pH value and glucose concentration in wound exudate. Reprinted with permission from Zhu YN, Zhang JM, Song JY, et al. A multifunctional pro-healingzwitterionic hydrogel for simultaneous optical monitoring of pH and glucose in diabetic wound treatment. Adv Funct Mater.2019:1905493. Copyright 2019, John Wiley and Sons.Citation239 (B) The formation of OxOBand from PUAO-CPO cryogels with ADSC-exos. Reprinted with permission from Shiekh PA, Singh A, Kumar A. Exosome laden oxygen releasing antioxidant and antibacterial cryogel wound dressing OxOBandalleviate diabetic and infectious wound healing. Biomaterials.2020;249:120020. Copyright 2020, Elsevier.Citation181
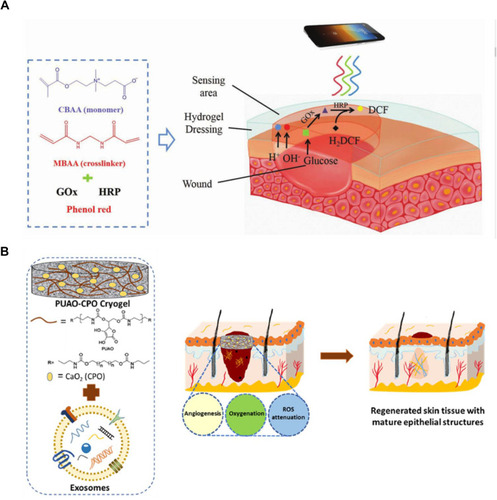
Figure 8 Schematic diagram of material synthesis. (A) Schematic of the hierarchical structure of LbL films into a single coating. The first (X) film is a hydrolytically degradable undercoating, while the second (Y) film contains the siRNA to be delivered. (B) Side-on schematic of hierarchical LBL film architecture. Reprinted with permission from Castleberry SA, Almquist BD, Li W, et al. Self-assembled wounddressings silenceMMP-9 and improve diabetic wound healing in vivo. Adv Mater. 2016;28:1809–1817. Copyright 2016, John Wiley and Sons.Citation79 (C) Schematic illustration of the synthesis procedure and rESW-responsive oxygen release from Nano-PFC. Adapted from Wang S, Yin C, Han X, et al. Improved healing of diabetic footulcer upon oxygenation therapeutics through oxygen-loading nanoperfluorocarbontriggered by radial extracorporeal shock wave. Oxid Med Cell Longev. 2019;2019:5738368. Creative Commons license and disclaimer available from: http://creativecommons.org/licenses/by/4.0/legalcode.Citation189
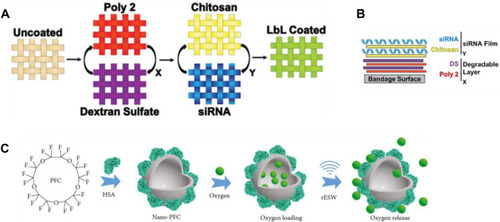
Figure 9 Potential therapies for diabetic wound repair. Strategies for manipulating the regeneration of diabetic wounds include the use of hydrogels (loaded with small molecules and stem cells, etc.), photothermal therapy, and materials that release oxygen. All of these elements have been demonstrated to have an effect on in vitro and in vivo models of wound healing. These repair mechanisms include vascularization, less ROS production, oxygen release, and antimicrobial resistance. Therefore, combining these strategies will undoubtedly change the result of diabetic wound healing.
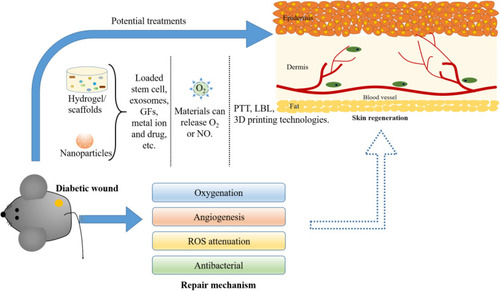