Figures & data
Figure 1 Examples of common nanomaterials used for targeted bone delivery. Titanium nanotubes, gold nanoparticles, calcium phosphate nanoparticles, and mesoporous silica nanoparticles (MSNs) constitute the inorganic nanomaterials. The organic nanomaterials include chitosan nanoparticles, poly(L-lactide-co-glycolide) PLGA nanoparticles, and liposomes. MSC: mesenchymal stem cell. Adapted with permission from Drug Discovery Today, Vol /edition number 22(9), Cheng H, Chawla A, Yang Y, et al, Development of nanomaterials for bone-targeted drug delivery, Pages No. 1336–1350, Copyright (2017), with permission from Elsevier.Citation125
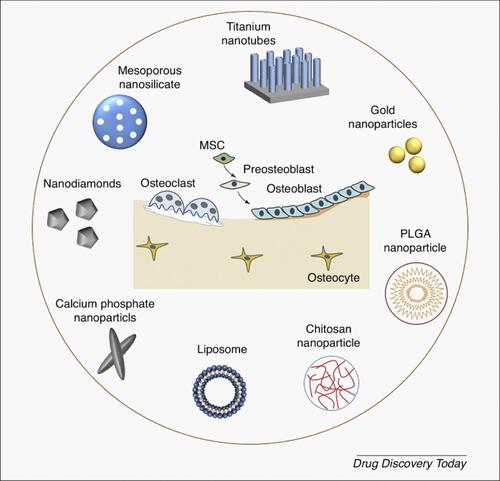
Figure 2 Schematic diagrams for the structure of RGD-CIS-liposomes and RGD-PbS-liposomes. Adapted with permission from Journal of Controlled Release, Vol /edition number 196, Wang F, Chen L, Zhang R, Chen Z, Zhu L, RGD peptide conjugated liposomal drug delivery system for enhance therapeutic efficacy in treating bone metastasis from prostate cancer, Pages No. 222–233, Copyright (2014), with permission from Elsevier.Citation131

Figure 3 Schematic representation of modified multifunctional MSNs loaded with anti-tumor drug DOX for targeted bone cancer treatment. Adapted with permission from Acta Biomaterialia, Vol 65, Martínez-Carmona M, Lozano D, Colilla, M, Vallet-Regí M, Lectin-conjugated pH-responsive mesoporous silica nanoparticles for targeted bone cancer treatment, Pages No. 393–404, Copyright (2018), with permission from Elsevier.Citation133

Figure 4 Representative image of exosome biogenesis and interaction with the recipient cell. (A) Early endosomes bud into MVBs (multivesicular bodies) as ILVs (intraluminal vesicles) from which exosomes are formed by merging with the plasma membrane. (B) Released exosomes carry a variety of components mainly proteins, lipids, and nucleic acids which can modulate the function once entered into the target cell. (C) Exosomes in the extracellular space can enter into the recipient cells by different mechanisms including (i) receptor binding, (ii) phagocytosis, and (iii) membrane fusion. Adapted with permission from Gulei D, Irimie AI, Cojocneanu-Petric R, Schultze JL, Berindan-Neagoe I. Exosomes-Small Players, Big Sound. Bioconjugate Chemistry. 2018; 29(3):635–648. Copyright © 2018 American Chemical Society.Citation173
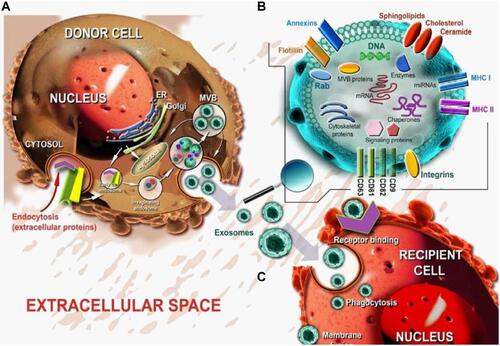
Figure 5 Illustration of regulation of bone homeostasis by the transfer of various biologically active molecules via bone-derived exosomes. Adapted with permission from Xie et al (2017). Copyright © 2016 The Authors. Journal of Cellular and Molecular Medicine published by John Wiley & Sons Ltd and Foundation for Cell and Molecular Medicine. This article is an open access article under the terms of the Creative Commons Attribution License (http://creativecommons.org/licenses/by/4.0/), which permits use, distribution, and reproduction in any medium, provided the original work is properly cited.Citation67
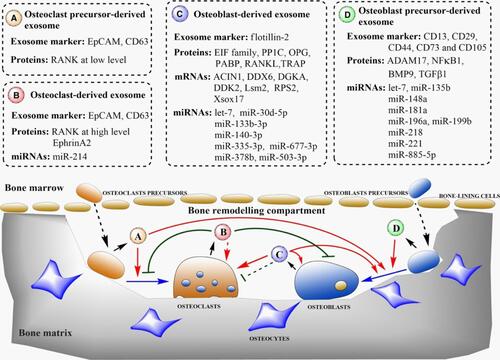
Figure 6 Schematic representation of RANK-RANKL mediated network of interaction between bone cells via the exosomal transfer of miRNA-214-3p. Adapted with permission from Gao et al Copyright © 2018, The Author(s). This is an open access article distributed under the terms of the Creative Commons CC BY license (http://creativecommons.org/licenses/by/4.0/), which permits unrestricted use, distribution, and reproduction in any medium, provided the original work is properly cited.Citation192
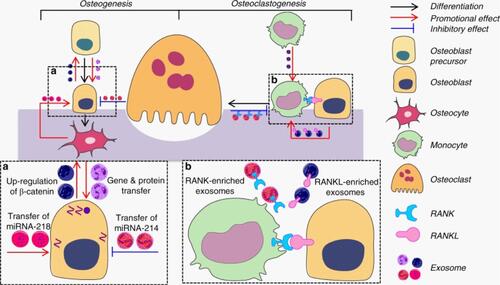
Figure 7 Various advantages associated with opting for exosomes as a drug delivery system. Reproduced from Peng, H; Ji, W; Zhao, R; Yang, J; Lu, Z; Li, Y; Zhang, X, Exosome: a significant nano-scale drug delivery carrier. Journal of Materials Chemistry B. 2020;8(34):7591–7608 with permission from Royal Society of Chemistry.Citation208
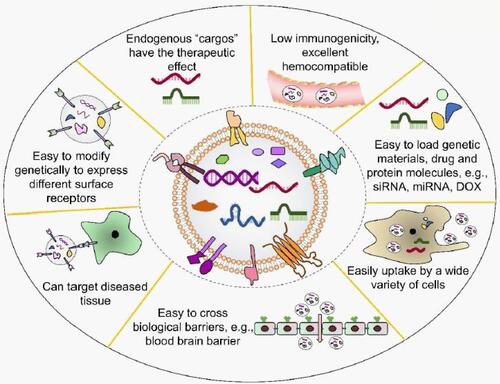
Figure 8 Bone targeting efficiency of EC-Exos in vivo. Biophotonic images showing tissue distribution of DiI labeled exosomes in mice injected with A) PBS, B) DiL reagent, C) DiI-labeled BMSCs-Exos, D) DiI-labeled MC3T3-Exos, and E) DiI-labeled EC-Exos. Adapted with permission from Song H, Li X, Zhao Z et al. Reversal of Osteoporotic Activity by Endothelial Cell-Secreted Bone Targeting and Biocompatible Exosomes. Nano Letters. 2019;19(5):3040–3048. Copyright © 2019 American Chemical Society.Citation222
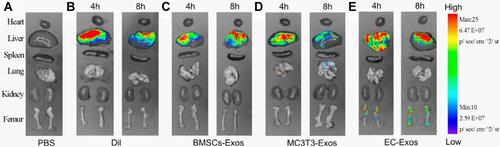
Figure 9 (A) Different approaches for drug incorporation into macrophage-derived exosomes in the presence of catalase. The novel exosomal-based catalase formulation (ExoCAT) efficiently crossed the blood-brain barrier showing the antioxidant effect in the neuronal cells of the PD mouse model. (B) The different loading formulations were examined by I) western blot, II) catalytic enzymatic activity, and III) catalase release.Citation213 All data were represented as mean±S.E.M (n=4); data were analysed using t-test; *p < 0.05, **p < 0.05. Adapted with permission from Journal of Controlled Release, Vol /edition number 207, Haney MJ, Klyachko NL, Zhao Y, et al, Exosomes as drug delivery vehicles for Parkinson’s disease therapy, Pages No. 18–30, Copyright (2015), with permission from Elsevier.Citation213
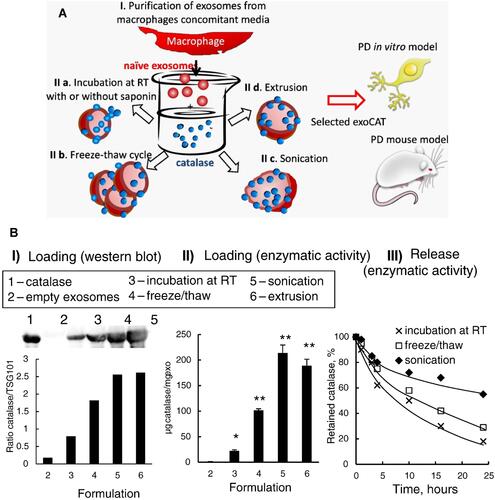
Figure 10 (A) I) Quantification of internalized Dox, Exo-Dox in MG63 cells for 1h, 4h, and 24h in terms of fluorescence intensity, II) cell viability of MG63 cells exposed to different concentrations of Exo-Dox and free Dox. (B) I) Quantification of internalized Dox, Exo-Dox in H9C2 cells for 1h, 4h and 24h in terms of fluorescence intensity, II) cell viability of H9C2 cells exposed to different concentrations of Exo-Dox and free Dox. Bars represent mean±standard deviation (n=3); data were analysed with two-way ANOVA; n.s.: no significant difference, ****P < 0.0001. Adapted with permission from Wei H, Chen J, Wang S et al Nanodrug Consisting Of Doxorubicin And Exosome Derived From Mesenchymal Stem Cells For Osteosarcoma Treatment In Vitro. Int J Nanomedicine. 2019;14:8603–8610. Dove Medical Press (DMP) publishes many of its articles under a Creative Commons Attribution Non-Commercial license (CC-BY-NC). This allows for the non-commercial reuse of the published paper so long as the published paper is fully attributed.Citation224
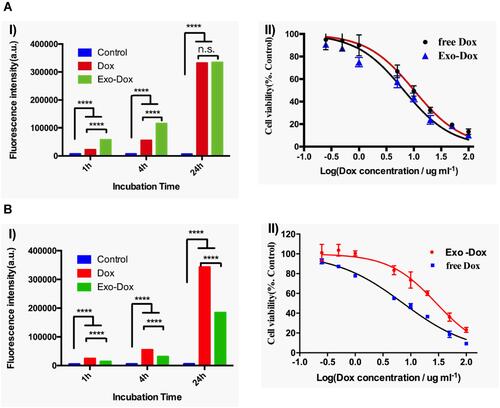
Figure 11 (A) Schematic illustration of aptamer functionalized exosomes to promote bone regeneration. (B) Schematic of conjugation procedure between the aptamer and STExos. (C) Representative FMT images of FL signals showing significantly more accumulation of STExo or STExo-Aptamer in the bone as compared to spleen, lungs, liver, and kidney. Reproduced from Luo ZW, Li FXZ, Liu YW et al Aptamer-functionalized exosomes from bone marrow stromal cells target bone to promote bone regeneration. Nanoscale. 2019;11(43):20884–20892 with permission from Royal Society of Chemistry.Citation225
Abbreviations: Lu, lungs; Li, liver; Sp, spleens; Ki, kidneys.
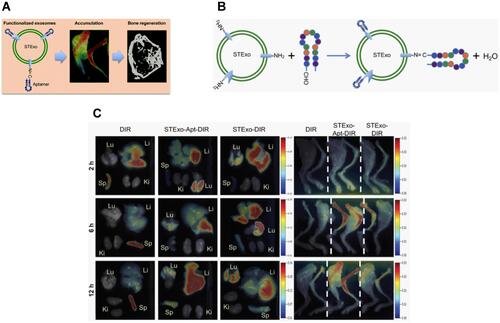
Figure 12 (A) Schematic illustration showing the preparation of novel biomimetic nanoparticles FPC-Exo/Dex, for enhanced targeting based on folic acid (FA) receptor–ligand interaction and better treatment of RA. (B) I) Real-time fluorescence imaging of CIA mice after i.v. injection with free DiD, Lip/Dex, Exo/Dex, and FPC-Exo/Dex, II) ex-vivo imaging of plasma and organs after 24 hr of i.v. injection, III) semi-quantitative fluorescence intensity in joints and plasma. Data were expressed as mean±SD (n=3) and analysed using two-way ANOVA; *p < 0.05, **p < 0.01, ***p < 0.001, ****p < 0.0001 vs mice treated with DID/FPC-Exo/Dex, #p < 0.05, ##p < 0.01, ###p < 0.001 are mice treated with DID/Lip/Dex compared to DID/Exo/Dex. (C) Histopathology analysis of ankle joints I) representative H&E staining, II) representative SO staining, III) histopathology score of SO staining. Data were expressed as mean±SD (n=3) and analysed using one-way ANOVA with Dunnett’s multiple comparisons test; **p < 0.01, ***p < 0.001 vs mice treated with saline; #p < 0.05 is mice treated with Lip/Dex compared to with Exo/dex. Adapted with permission from Yan et al (2020). This article is licensed under a Creative Commons Attribution 4.0 International License, which permits use, sharing, adaptation, distribution and reproduction in any medium or format, as long as you give appropriate credit to the original author(s) and the source, provide a link to the Creative Commons license, and indicate if changes were made. (http://creativecommons.org/licenses/by/4.0/).Citation228
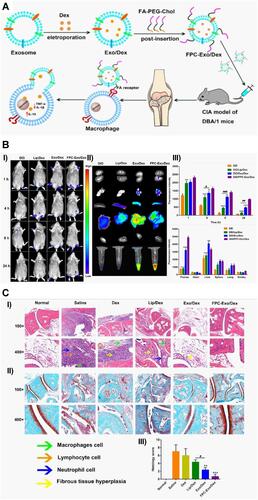
Table 1 Patents Related to the Diagnostic and Therapeutic Application of Exosomes Towards Bone Disorders
Table 2 Innovative Methods of Exosome Modifications for Improved and Targeted Drug Delivery
Figure 13 Synthesis of liposome-exosome hybrid nanosystem. (A) Schematic illustration of the three mainly used methods: freeze-thaw, incubation, and sonication for the synthesis. Adapted with permission from Elkhoury et al (2020). Copyright © 2020 by the authors. License MDPI, Basel, Switzerland. This is an open access distributed under the terms of the Creative Commons (CC BY) license (http://creativecommons.org/licenses/by/4.0/).Citation249 (B) Fusion of RAW 264.7 cell-derived exosomes with liposomes by using freeze-thaw technique. Adapted with permission from Sato et al Copyright © 2016, The Author(s). This is an open access article distributed under the terms of the Creative Commons CC BY license, which permits unrestricted use, distribution, and reproduction in any medium, provided the original work is properly cited.Citation251
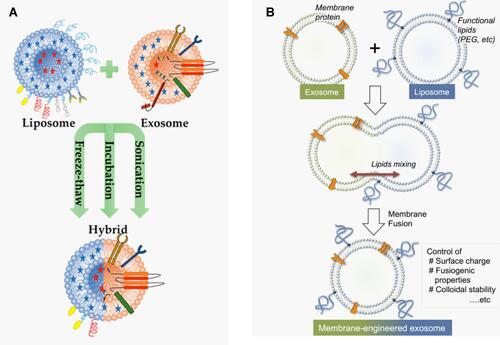
Figure 14 Representative results showing cellular tropism of cRGD-Exosomes (surface-modified exosomes) in vitro. (A) Fluorescence microscopic images of native and modified exosomes, green color indicates DiI-stained lipid membranes (of only native exosomes) and red is Cy5.5 that indicates the peptide-functionalized cy5.5 labeled exosomes, scale bar: 5 µm. The arrows indicate the colocalization between both the cy5.5 and DiI. (B) Western blotting of integrin subunits αv, β3. β5, α5, β1, αIIb from two cell lines U87 and HeLa cells that represent different integrin expression patterns, the results indicate that HeLa does not express αvβ3 while U87 cells express high levels of αvβ3. (C) Flow cytometry results indicate that the uptake level as measured by the fluorescence intensity of cRGD-Exo by αvβ3-positive U87 cells is about 3-times higher than that of exosomes. (D) The relative percentage of Cy5.5-positive HeLa or U87 cells compared to Cy5.5-Exo group, c(RGDyK) peptide acts dose-dependently. Data were represented as mean±SEM (n=5); n.s., not significant; ***P < 0.001 by Student’s t-test. (E) Fluorescence images of Cy5.5-labeled exosomes or Scr-Exo (exosomes with functionalized scrambled peptide) and cRGD-Exo post 30 minutes incubation with U87-GFP and HeLa cells. Green indicates U87-GFP cells, magenta color represents Cy5.5-labeled exosomes, Scr-Exo, or cRGD-Exo and blue color indicates nuclei stained with Hoechst. cRGD-Exo entered more efficiently into U87-GFP cells as compared rest groups. The lower panel figure shows magnified images; arrows indicate internalized exosomes; scale bar, 30 µm. Adapted with permission from Biomaterials, Vol /edition number 150, Tian T, Zhang H-X, He C-P, et al, Surface functionalized exosomes as targeted drug delivery vehicles for cerebral ischemia therapy, Pages No. 137–149, Copyright (2018), with permission from Elsevier.Citation216
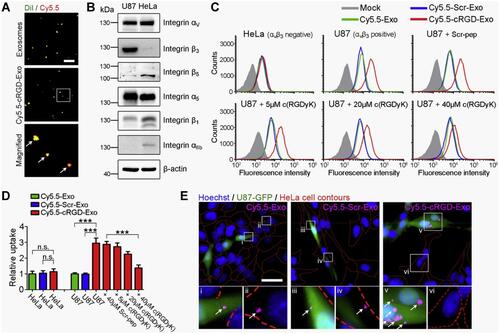
Figure 15 (A) Characterization of EXO and IDEM. I) Size (nm) and concentration (particles/mL) as per NTA, II) the number of CD63 positive EXO and IDEM obtained from ELISA (*P < 0.05), III) positive signal in flow cytometry for APC-CD81 antibody stained EXO and IDEM, IV) particle morphology revealed from scanning electron microscopy, V) presence of tsg-101 marker obtained from Western blotting. (B) I) Effect on 3D spheroid ovarian cancer model at 24h, 48h, 72h and 96h of treatment with 5µg/mL of EXO and IDEM. Red arrows showing loss of integrity, change in color due to necrosis, II) percentage of cell viability showing greater effectiveness of IDEM-DOXO than only DOXO at 24 h and 96h (*P < 0.05). Data indicating EXO-DOXO to be most effective at all-time points (***P < 0.001 at 72h and 96h). Data were analysed by a two-tailed Student’s t-test and represented as mean±standard deviation; *P < 0.05, **P < 0.01, ***P < 0.001. Adapted with permission from Pisano S, Pierini, I, Gu Jet al Immune (Cell) Derived Exosome Mimetics (IDEM) as a Treatment for Ovarian Cancer. Front Cell Dev Biol. 2020;8: 553576.Copyright © 2020 Pisano, Perini, Gu, Gazze, Francis, Gonzalez, Conlan and Corradetti. This is an open access distributed under the terms of the Creative Commons Attribution License (CC BY).Citation256
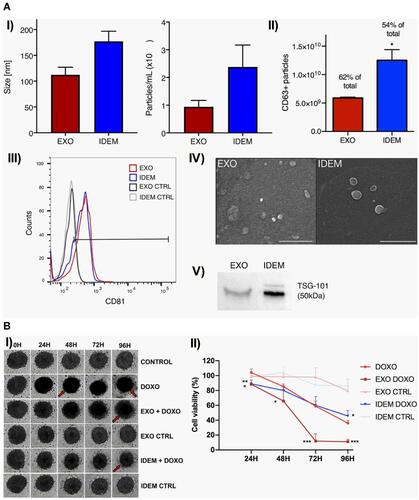