Figures & data
Figure 2 Representative nanocarriers for drug delivery, ie, liposomes (left) and polymeric nanoparticles (right). Liposomes are self-assembling vesicles with a bilayered membrane structure containing amphiphilic molecules (phospholipids) and hydrophobic and hydrophilic groups that self-assemble in water. Polymeric nanoparticles are biocompatible and biodegradable polymeric nanoformulations in which drugs are dissolved, entrapped, or conjugated to the surface of the nanoparticles.
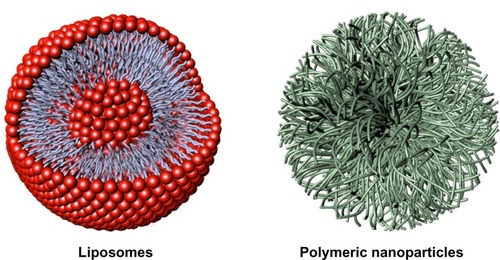
Table 1 Examples of nontargeted nanosystems in clinical use for anticancer therapy
Figure 3 Strategies adopted for drug targeting and localization of nanosystems to tumor cells and tissues.
Notes: (A) Passive drug targeting. Circulating nanoparticles passively extravasate in solid tumor tissue via the enhanced permeability of blood vessels, ie, through the disorganized and leaky vasculature surrounding the solid tumor coupled with the absence of lymphatic drainage, and preferentially accumulate in tumor cells (the EPR effect). (a) The drug is released into the extracellular matrix and diffuses through the cells and tissue. (B) Active drug delivery. Once nanoparticles passively extravasate and concentrate in the target tissue via the EPR effect, the presence of ligands grafted onto the nanoparticle surface enable active targeting of the nanoparticles to receptors that are overexpressed on tumor cells or tissue, resulting in enhanced uptake and internalization via receptor-mediated endocytosis. (b) Tumor-specific ligands on the nanoparticles bind to cell surface receptors, triggering internalization of the nanoparticles into the cell through endosomes on which, due to an internal acidic pH, the drug is released from the nanoparticles and diffuses into the cytoplasm. (C) Active drug targeting to endothelial cells. Nanoparticles can be targeted to bind to angiogenic endothelial cell surface receptors with the aims of: enhancing drug accumulation in the tumor endothelium, thereby inhibiting growth of blood vessels supplying the tumor rather than inhibiting tumor cells per se (c); and improving delivery of chemotherapeutic agents to tumor cells via the EPR effect with the potential to act synergistically in targeting both the vascular tissue and tumor cells. (D) Triggered drug delivery by stimuli-sensitive nanomedicines. Nanoparticles passively accumulate in the tumor via the EPR effect. After localization at the target site or while circulating in the tumor vasculature (d), the nanoparticles can be activated by external stimuli (eg, hyperthermia, light, magnetic fields, ultrasound) that induce release of the payload drugs. Images adapted from J Control Release, 161(2), Lammers T, Kiessling F, Hennink we, Storm G, Drug targeting to tumors: principles, pitfalls and (pre-) clinical progress, 175–187, Copyright 2012, with permission from Elsevier.Citation10
Abbreviation: EPR, enhanced permeability and retention.
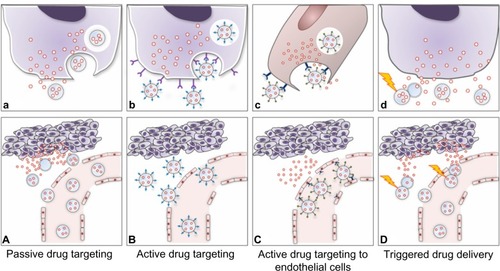
Table 2 Tumor-targeted nanomedicines in clinical development
Figure 5 Graphic representation of BIND-014 composed of a biodegradable and hydrophobic PLA polymeric core and a hydrophilic PEG corona decorated with small-molecule (a pseudomimetic PSMA-directed dipeptide) targeting ligands, and a semisynthetic taxane (docetaxel) as an encapsulated anticancer drug.
Note: Images adapted with the permission of BIND Therapeutics (by Gael McGill), Harvard Medical School, and Digizyme Inc.
Abbreviations: PLA, poly(lactic acid); PEG, poly(ethylene glycol); PSMA, prostate-specific membrane antigen.
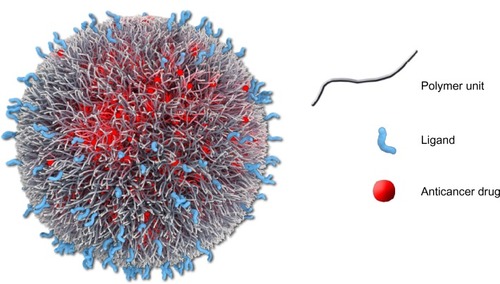
Figure 6 Development of BIND-014 by high-throughput technology. Multifactorial optimization of polymeric nanosystems was performed by varying a range of formulation process parameters and physicochemical nanoparticle properties. The green dotted line indicates optimized nanoparticle parameters.
Notes: Molecular weight expressed in Dalton (Da); zeta potential expressed in millivolts (mV); diameter expressed in nanometers (nm); initial release rate calculated by mass flow per unit time (%/h). From Hrkach J, Von Hoff D, Mukkaram Ali M, et al. Preclinical develop ment and clinical translation of a PSMA-targeted docetaxel nanoparticle with a differentiated pharmacological profile. Sci Transl Med. 2012;4(128):128ra139.Citation116 Adapted with permission from AAAS.
Abbreviations: NP, nanoparticles; PEG, poly(ethylene glycol); PL(G)A, poly(lactic acid) or poly(D,L-lactide-co-glycolide).
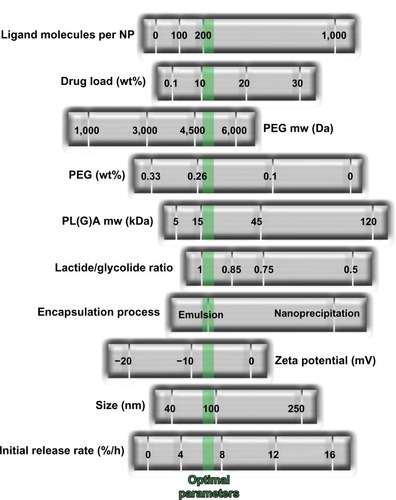