Figures & data
Scheme 1 This study primarily focuses on the introduction, applications, challenges, and future prospects of anesthetic nanomedicines.
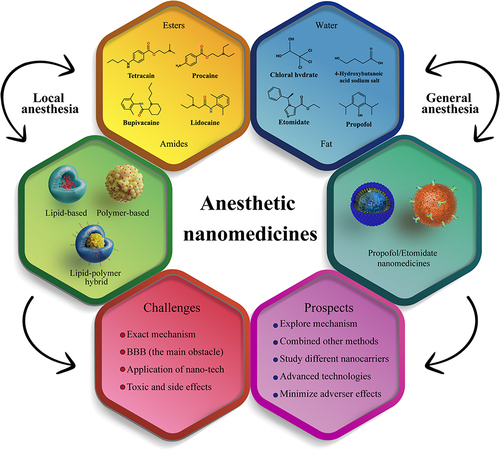
Table 1 Properties of Local Anesthetic Nanomedicines
Figure 1 (a) The duration of nerve block after administering tetracaine, along with 2-minute exposure to 300 mW/cm² irradiation immediately following injection. (b) The nerve block resulting from the irradiation of P407-CM-T at different irradiances and exposure durations. (c) The impact of energy density on the duration of the induced nerve block (n = 4). (d) The temporal profile of nerve block elicited by several instances of light activation was assessed, where the blue arrows indicate the triggering of LED for a period of 2 minutes at 300 mW/cm². (e) The average duration of nerve block following each triggering event in d (n = 4). Reproduced from Zhang W, Ji T, Li Y, et al. Light-triggered release of conventional local anesthetics from a macromolecular prodrug for on-demand local anesthesia. Nat Commun. 2020;11(1):2323. Creative Commons.Citation51
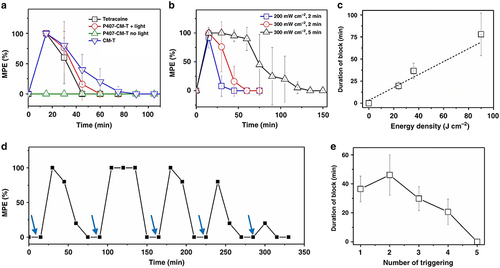
Figure 2 A polymer-TTX conjugate, which contains a high concentration of TTX and is designed for slow release, is positioned near a nerve. The delivery system includes a chemical permeation enhancer that facilitates the entry of TTX into the nerve. The hyperbranched structure of the polymer-TTX conjugate shown here has a TTX loading capacity that is significantly greater than the conjugates produced in the current experiment. Reproduced from Zhao C, Liu A, Santamaria CM, et al. Polymer-tetrodotoxin conjugates to induce prolonged duration local anesthesia with minimal toxicity. Nat Commun. 2019;10(1):2566. Creative Commons.Citation57
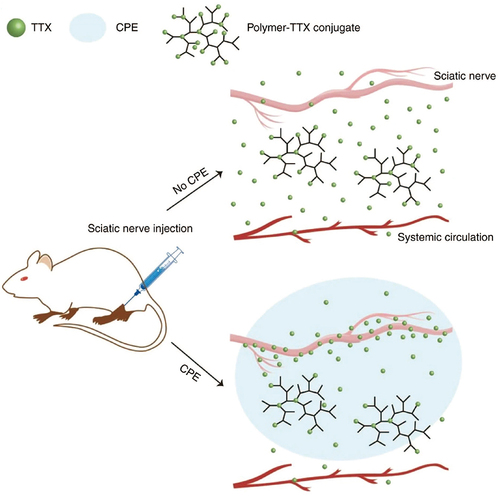
Figure 3 (a) The process of producing LDC@LPHNs for use in local anesthetics applications. (b) The effect of various concentrations of LDC (0, 1, 5, 10, 20 μM) on the proliferation of NIH-3T3 cells after 24 hours of treatment.; Evaluation of the anesthetic effects in a Swiss animal model in vivo; Observation of vocalization response phase progression triggered by electrical shocks in mice after subcutaneous administration of LDC alone, LDC@NPs, and LDC@LPHNs formulations as analgesics. Reprinted from Process Biochem, volume102, Wang Y, Qin M, Hou J, Chen Y. In vitro and in vivo evaluation of a lidocaine loaded polymer nanoparticle formulation co-loaded with lidocaine for local anesthetics effect. 333–340, copyright (2021), with permission from Elsevier.Citation42
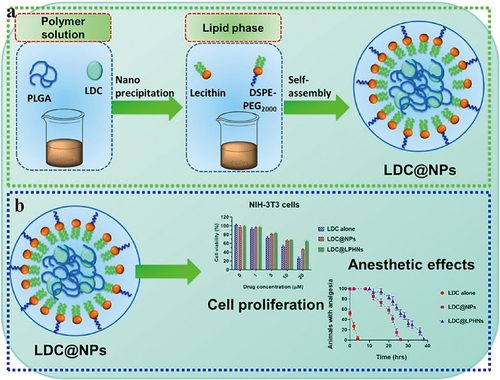
Table 2 Properties of General Anesthetics
Table 3 Properties of General Anesthetic Nanomedicines
Figure 4 (A) GQY’s chemical structure. (B) Chemical structure of pyrene and general anesthetics, such as propofol, etomidate, and ET26. (C) Schematic depiction of GQY drug injection. The hydrophobic side chains of glutamine and tyrosine are depicted in purple. Reprinted with permission from Dove Medical Press. Liu J, Peng F, Kang Y, et al. High-loading self-assembling peptide nanoparticles as a lipid-free carrier for hydrophobic general anesthetics. Int J Nanomedicine. 2021;16:5317–5331.Citation85
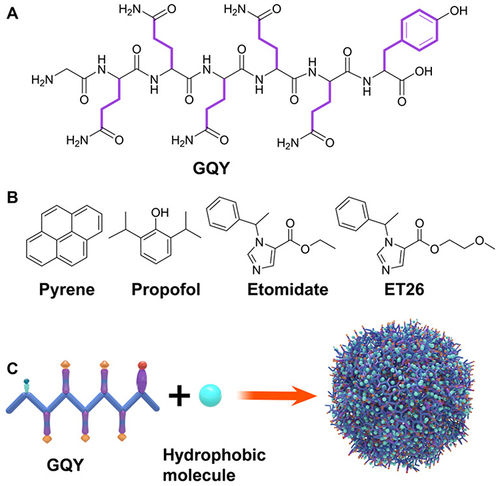
Figure 5 (a) The process of creating HLPAH nanoclusters. (b) The BBB-triggered HLPAH nanoclusters to be released in vivo. Reprinted from Biomaterials, volume 113, Gao W, Liu Y, Jing G, et al. Rapid and efficient crossing blood-brain barrier: hydrophobic drug delivery system based on propionylated amylose helix nanoclusters. 133–144, Copyright (2017), with permission from Elsevier.Citation84
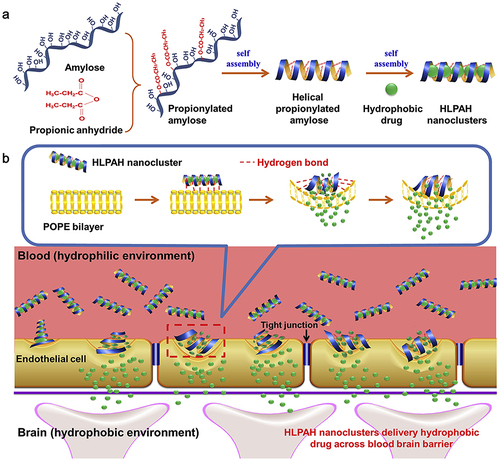