Figures & data
Figure 1 Structure of liposomes and examples for membrane components.
Notes: (A) Schematic representation of a liposome. The vesicle is formed by a membrane bilayer of phospholipids enclosing an aqueous internal core that can be loaded with hydrophilic molecules (1). The vesicle surface is often shielded by a polymer coating, eg, polyethylene glycol (2). Lipophilic molecules can be incorporated into the membrane bilayer (3). To destabilize the membrane for facilitating drug release, surfactants (eg, lysolipids) are incorporated into the membrane (4). Surface modification with antibodies, antibody fragments, or ligands yields formulations for active targeting towards the desired epitope (5). Incorporation of cationic lipids like DPTAP yields vesicles for endosomal targeting (6). Cholesterol is incorporated to stabilize the formulations (7). (B) Examples of amphiphilic molecules forming lamellar structures.
Abbreviations: DPPC, 1,2-dipalmitoyl-sn-glycero-3-phosphocholine; DPPGn, 1,2-dipalmitoyl-sn-glycero-3-phosphodiglycerol; DPTAP, 1,2-dipalmitoyl-3-trimethylammonium-propane.
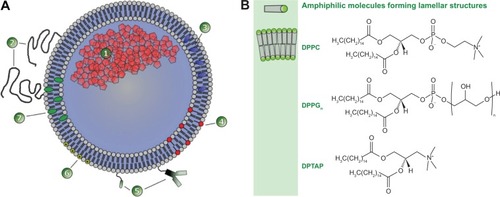
Figure 2 Different methods of drug loading into pre-formed liposomes.
Notes: (A) Active drug loading of weak base molecules (eg, doxorubicin) into preformed liposomes with a pH gradient. In the basic extraliposomal buffer, the drug is uncharged and able to transfer across the membrane bilayer. Inside, the drug is protonated due to the acidic buffer and trapped. The loading method reaches an encapsulation efficacy of up to 98%. (B) Passive drug loading (eg, gemcitabine) is achieved by incubating the drug and vesicles at temperatures where the membrane is in the liquid-disordered phase state and therefore permeable for the drug. After cooling, the membrane is in the solid gel phase state, and its permeability is negligible. Because the passive loading is an equilibrium process depending on the ratio between intraliposomal and extraliposomal volume, the encapsulation efficacy is low and the formulation has to be purified from the nonencapsulated drug.
Abbreviation: dFdC, gemcitabine; DOX, doxorubicin.
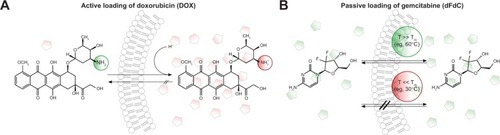
Figure 3 Schematic illustration of targeting concepts with liposomes.
Notes: (A) Passive targeting of drug encapsulated in liposomes is achieved by extravasation of the vesicles into the tumor tissue due to the leaky tumor vasculature (1). This enhanced permeability and retention effect is the rate-limiting step in vesicle accumulation and requires highly stable liposomes with a long circulation half-life in the bloodstream. Even liposomes with targeting moieties attached to their surface (eg, immunoliposomes) have to exploit the enhanced permeability and retention effect to interact with their targets (2). An alternative approach is endothelial targeting with cationic liposomes (3). The drug delivered by stable liposomes is not fully bioavailable and does not reach the tumor cells in sufficient amounts (4). Even after endocytosis of the vesicles, drug delivery is limited, since the drug fails to escape from the endosomal compartment (5) and is subsequently degraded in the lysosome. (B) Intravascular drug release is achievable by thermosensitive liposomes. Release is externally steerable by changing the focus of local heating. After entering the heated tissue, the drug is released into the bloodstream (6), generating a high local drug concentration. The concentration gradient increases the penetration depth of the drug inside tumors (7). The heat-triggered drug release inside the target area overcomes the limitation of traditional targeting concepts, because the drug is fully bioavailable and able to enter its site of action inside the cell (8).
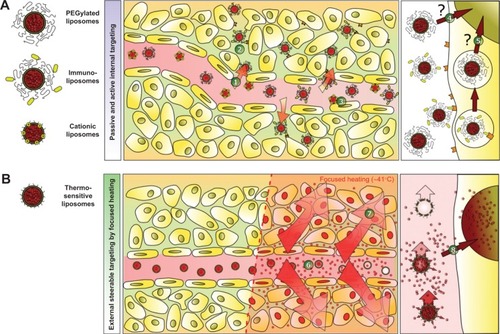
Figure 4 Factors affecting drug release from thermosensitive liposomes.
Notes: (A) The encapsulated drug is released by passive transfer driven by a concentration gradient (1). At body temperature, the phospholipids are in the solid gel (Lβ) phase state characterized by low permeability to hydrophilic compounds (2). By increasing the temperature above the phase transition temperature (Tm), the phospholipids are in a liquid-crystalline (Lα) phase state with higher permeability to hydrophilic compounds, because of the higher disorder and movement in the phospholipid packing (3). Around Tm, permeability is the highest because of coexistence of membrane areas in both phases (4). Permeability is further mediated by disturbances in lipid packing induced by lipid incorporation (5), lipid loss (7), and interaction with blood components (8–10). As a specific mechanism of ultrafast drug release from lysolipid-containing TSL, the formation of membrane pores (6) by lysolipids around Tm was shown. (B) Tm of DPPC/DSPC/DPPG2 50:20:30 (mol/mol) (DPPG2-TSL) measured by dynamic scanning calorimetry. (C) Temperature-dependent release of hydrophilic compounds from DPPG2-TSL measured in fetal calf serum.
Abbreviations: CF, carboxyfluorescein; dFdC, gemcitabine; DOX, doxorubicin; DPPC, 1,2-dipalmitoyl-sn-glycero-3-phosphocholine; DSPC, 1,2-distearoyl-sn-glycero-3-phosphocholine; DPPG2, 1,2-dipalmitoyl-sn-glycero-3-phosphodiglycerol; T, temperature; Tm, phase transition temperature; TSL, thermosensitive liposomes.
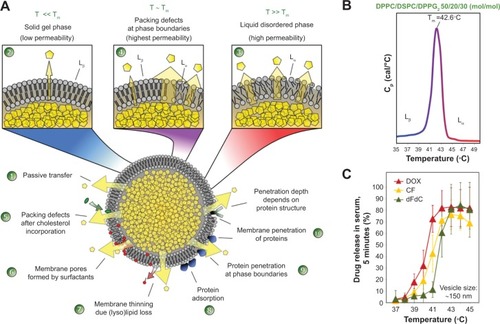
Figure 5 Micellar-forming amphiphilic molecules as membrane component in liposomes.
Notes: (A) Example of micellar-forming amphiphilic molecules (surfactants) used in liposomal formulations. DSPE-PEG2000 is used to increase the circulation half-life of liposomes, and lyso-PC, Brij78, and HePC can be used in thermosensitive formulations to facilitate drug release. (B) Surfactants are incorporated into the membrane bilayers at a low molecular content, but induce formation of usually unwanted structures (eg, membrane pores, dissolution of liposomes) at high concentrations.
Abbreviations: DSPE-PEG2000, 1,2-distearoyl-sn-glycero-3-phosphoethanolamine-N-methoxy(PEG)-2000; lyso-PC, acyl-sn-glycero-3-phosphocholine; Brij78, polyoxyethylene (20) stearyl ether; HePC, hexadecylphosphocholine.
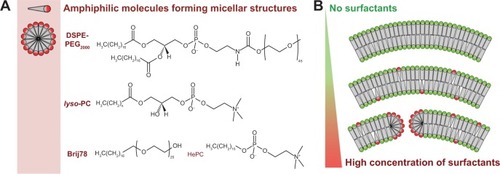
Table 1 Overview of thermosensitive liposomes