Figures & data
Figure 1. Wobble modifications in tRNAs required to decipher AUA codons in 3 domains of life. (A) Chemical structures of modified nucleosides found at the first letter of anticodons in tRNAs responsible for AUA codons. (B) Anticodons with wobble modifications in tRNAs for AUA codons in 3 domains of life.
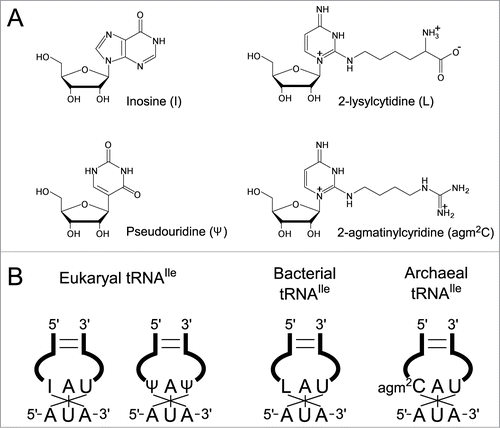
Figure 2. A single modification changes both codon and amino acid specificities of tRNAIle2. Precursor tRNAIle2 with CAU anticodon behaves like tRNAMet, since it accepts Met and decodes AUG codons. In bacteria, C34 is modified to L34 by TilS using Lys and ATP as substrates. tRNAIle2 with LAU anticodon accepts Ile and decodes AUA codons. In archaea, C34 is modified to agm2C34 by TiaS, using agmatine and ATP as substrates. tRNAIle2 with agm2CAU anticodon accepts Ile and decodes AUA codons.
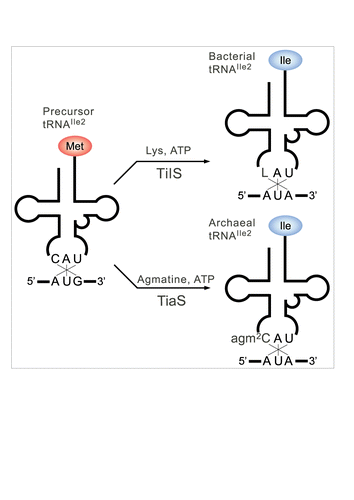
Figure 3. Chemical structures of wobble cytidine modifications base-pairing with A. (A, B) Two models of L-A base pairings. As several resonance forms of L are possible, the positive charge of the base is delocalized. “R” represents a side chain of L. Imine tautomer of L pairs with A in a Watson-Crick geometry (A). Enamine tautomer of L pairs with A in a wobble geometry (B). (C) An agm2C34-A3 base pair at the ribosomal A-site observed in the crystal structure of a 70S ribosome in complex with tRNAIle2 and an AUA codon. An imine tautomer of agm2C is described as a representative of a number of possible tautomers. Coordinates are obtained from 3ZN7.
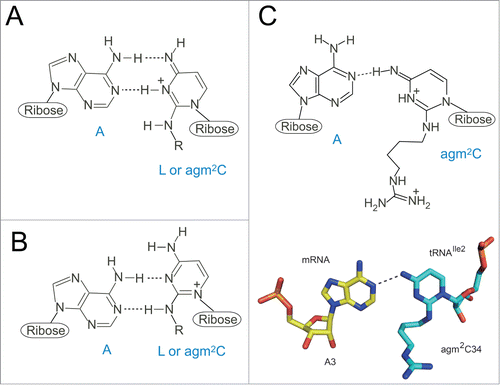
Figure 4 (See previous page). Comparison of tRNA recognition by TilS and TiaS. (A) Overall structure of the GkTilS-tRNAIle2 complex. The NTD, CTD1, and CTD2 are green, brown, and pink, respectively. The helical linker that connects NTD and CTD1 is gray. tRNAIle2 is yellow. (B) Overall structure of the AfTiaS in complex with tRNAIle2 and ATP. The TCKD, FLD, OBD, and ZRD are depicted in green, cyan, brown, and pink, respectively. ATP is represented as a stick model, and its carbon atoms are depicted in white. (C) Interactions between GkTilS CTD2 and the tRNAIle2 acceptor stem. (D) Interactions between AfTiaS ZRD and the tRNAIle2 acceptor stem. Hydrogen bonds are represented by dotted lines.
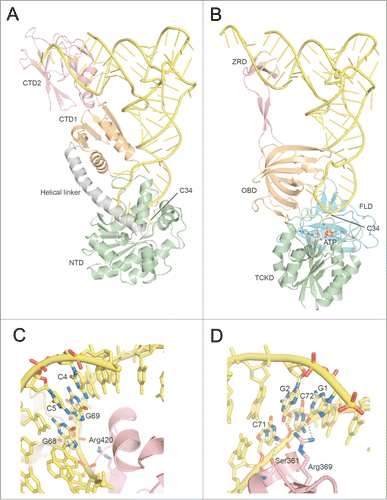
Figure 5. Anticodon recognition and catalytic sites of TilS and TiaS. (A) Recognition of the anticodon loop of tRNAIle2 by NTD of GkTilS. (B) The structure of the active site of the GkTilS-tRNAIle2 complex, superimposed with the ATP bound to the catalytic site of AaTilS. The target nucleoside, C34, is represented as a yellow stick model. The ATP observed in the AaTilS-ATP complex is superimposed (white stick model). The ATP is situated near the N-type ATP pyrophosphatase motif (PP-loop) (blue). (C) Recognition of the anticodon loop of tRNAIle2 in the AfTiaS-tRNAIle2-ATP ternary complex structure. (D) The structure of the active site of the AfTiaS-tRNAIle2-ATP ternary complex structure. C34 and ATP are represented as yellow and white stick models, respectively. The catalytic residues (Asp8, Asp9, Asp11, and pThr18) are also represented as stick models. (E) The structure of the active site of the AfTiaS-tRNAIle2-AMPcPP-agmatine quaternary complex structure. C34, AMPcPP, and agmatine are represented as yellow, white, and light blue stick models, respectively. The catalytic residues (Asp8, Asp9, Asp11, and pThr18) are also represented as stick models. The coloring schemes of the proteins are the same as those in .
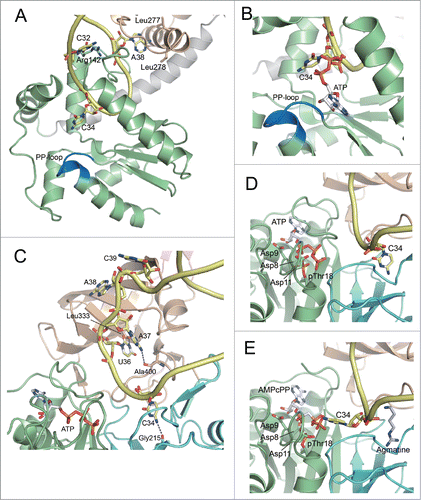
Figure 6. Reaction schemes for formation of L and agm2C (A) Two-step lysidine formation catalyzed by bacterial TilS. TilS activates the C2 carbonyl group of C34 by adenylation and releases PPi. Then, the ϵ-amino group of lysine attacks the adenylated C2 group to release AMP and complete lysidine formation. (B) The 3-step reaction of agm2C formation catalyzed by TiaS. First, TiaS hydrolyzes the α-β phosphodiester bond of ATP to produce AMP and PPi. Second, the C2 carbonyl oxygen of C34 attacks the γ-phosphorous atom to form the phosphorylated C34 (p-C34) intermediate, releasing β-Pi. Third, the primary amino group of agmatine attacks the C2 carbon of the p-C34 intermediate to release γ-Pi and form agm2C.
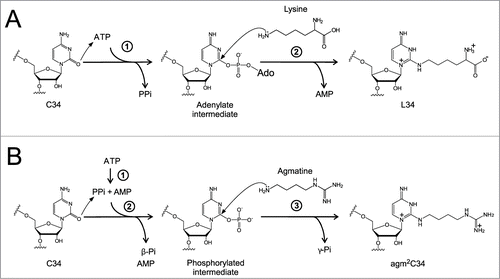
Table 1. Organisms lacking tilS or tiaS.