Figures & data
Figure 1. Models for boundary formation. (A) Classical models for cell sorting and tissue separation based on differences in cell-cell adhesion and cortical contractility. Sorting may be achieved (A) by cell-type specific expression of different cell adhesion molecules (CAMs) with stronger affinity for homotypic binding than for heterotypic interaction, or (A’) by differences in adhesive strength between cell types (differential adhesion hypothesis, or DAH), resulting for instance from different levels of adhesion molecules. (A”) Difference in cell cortex contractility can also lead to cell sorting. Recently, adhesion and contractility have been integrated into a single description of adhesive cell interactions, but for simplicity of depiction they are shown in separate panels here. B. Cell sorting/tissue separation may also be produced by the complementary expression of repellent cell surface cues, which would trigger local cortex contraction and cell repulsion at heterotypic contacts, a process termed contact inhibition.
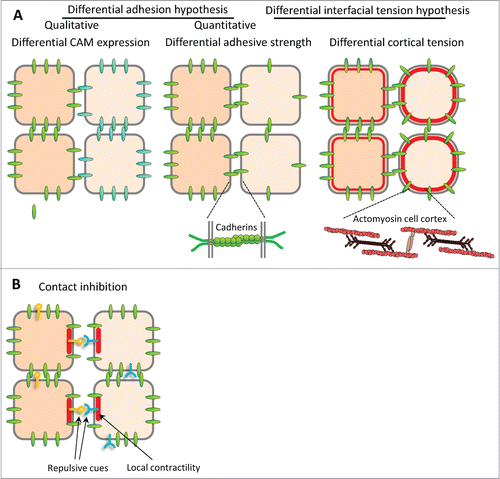
Figure 2. Ephrin and Eph receptor expression in the early Xenopus embryo. Transcripts of 7 ephrins and 11 Eph receptors are detected in the early Xenopus stages. The color code symbolizes relative mRNA levels, determined by real-time RT-PCR using tissues dissected from late blastula (stage 9.5), early gastrula (stage 10.5) mid gastrula (stage 11.5) and early neurula (stage 14). Gray color indicates that levels were too low and variable to be quantified.
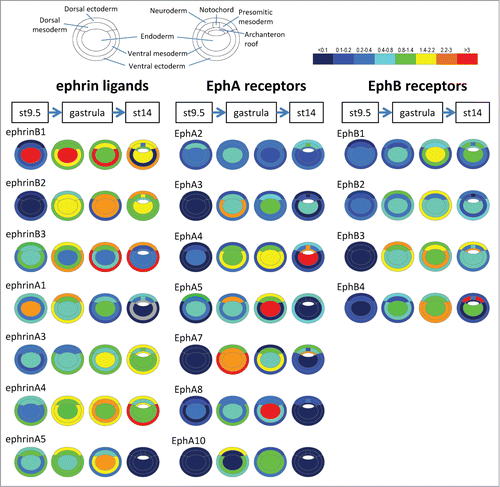
Figure 3. Ephrin and Eph receptor distribution at vertebrate embryonic boundaries. Each diagram represents the pattern observed in the species where most studies were performed: (A) Dorsal ectoderm-mesoderm boundary in the early Xenopus gastrula. (B) Ventral ectoderm-mesoderm boundary in the middle Xenopus gastrula. (C) Notochord-presomitic boundary in the late Xenopus gastrula. (D) Somitogenesis in zebrafish. (E) Hindbrain segmentation in zebrafish. (E’) Comparative diagram from available data in the 4 species, zebrafish (z), Xenopus (x), chicken (c) and mouse (m). Species abbreviated in black denotes expression, in red absence of (or low) expression in the corresponding segment. Absence of symbol indicates that the gene was not investigated in this species. Note that the ephrinB3 gene is altogether absent from chicken. (F) Eye field segregation in zebrafish. In (A–C), the font and box size represent relative enrichments. Comparative data on expression levels are not available for the other systems, but in a few instances lower expression was clearly observed in the hindbrain (ephrinB2 in r1,r3 and r6, EphA4 in r2, EphB4 in r4). Patterns were built based on expression data from:Citation35,36,38,47 for (A–C), Citation32,42,48,52,59,122-125 for (D), Citation33,48,53-56,124,126-128 for (E), and Citation37 for (F), complemented with the Mouse Gene Expression Databases for zebrafish (http://zfin.org) and mouse (http://www.informatics.jax.org/gxd).
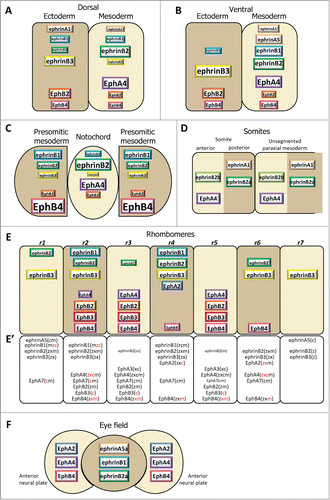
Table 1. Summary of functional results implicating ephrin-Eph signaling in formation of vertebrate boundaries
Figure 4 (See previous page). Ephrin-Eph signaling in embryonic tissues. (A) Multiple activities and cross-talks. Ephrin-Eph receptor interactions typically activate an intracellular signal that induces reorganization of the actin cytoskeleton, including increased actomyosin contraction, leading to retraction. This repulsive activity is thought to be triggered only at high levels of signal. It requires disruption of the ephrin-Eph bonds which connect the 2 cells. This can occur either by endocytosis, or by shedding of the ephrin/Eph extracellular domains by ADAMs proteinases (not shown). It is believed that, under certain condition, in particular under low ephrin-Eph signaling, ephrin-Eph interactions contribute on the contrary to cell-cell adhesion. Note that the exact mechanisms that control adhesive versus repulsive modes are not well understood. Like many other ligand-receptor systems, ephrin-Eph signaling influences other aspects of cell function, such as gene regulation or cell-matrix adhesion. Ephrins and Eph receptors have been found to directly associate with other cell surface components, such as tight junctions. The effect of these interactions is still poorly understood. When co-expressed in the same cell, ephrins and Eph receptors may also establish cross-talks, which are generally considered to lead to signal dampening. (B–E) Examples of ephrin-Eph configurations (top 2 cell rows) and of predicted consequences of loss-of-function on signaling and adhesion within and between tissues (lower rows). (B’–E’) Resulting effect on tissue cohesion/separation. Sorting/separation is symbolized by a gap between the cells. (B) Embryonic tissues express multiple ephrins and Eph receptors. In the simplest cases, they are expressed in fully complementary patterns, such that signaling is exclusively triggered at the tissue interface, where repulsion antagonizes cadherin cell adhesion, resulting in low effective adhesion. Lowering ephrins or Ephs is predicted to decrease repulsion/increase adhesion at the tissue interface and cause their fusion, without major effect on intratissular contacts. Note that the systematic expression of several ephrins and/or several Ephs observed in all systems creates partial - and sometimes even full - redundancy. As a consequence, more than one of these components may need to be removed to inhibit separation. (C) In more complex cases, expression is only partially complementary. Here ephrins and Eph receptors can also interact in one or both tissues. These intracellular interactions can generate repulsion, which may confer to the tissue different physical properties, e.g., lower rigidity and higher migration. (D) Under certain circumstances, Ephrin-Eph intratissular signaling may generate a pro-adhesive activity, effectively increasing tissue cohesion. In this case, ephrin/Eph depletion is predicted to decrease tissue cohesion, and could even in principle result in artificial sorting from the tissue of origin, while concomitant inhibition of repulsion across the boundary could lead the depleted cells to join the opposite tissue. (E) In a situation where intratissular signaling is weakly repulsive (C), partial loss-of-function may switch from repulsive to adhesive mode. Depleted cells may sort into a compact independent population.
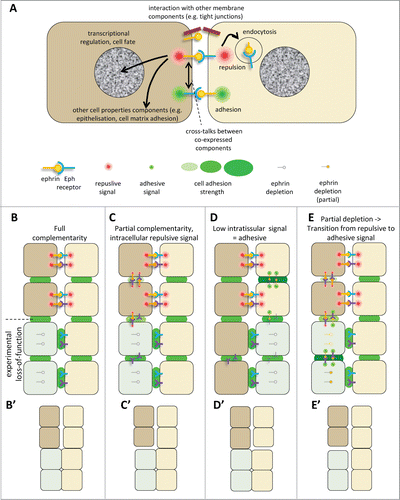
Table 2. Preferred ephrin-Eph pairs. A. Pairs formed by ephrinB ligands. B. Pairs formed by ephrinA ligands. In vitro: Summary of measurements of interactions between purified recombinant extracellular domains.Citation62,65,67,111-121 The symbolized strengths of the interactions are symbolized by ++ (strong, Kd < 2 nM), + (medium, Kd ∼2–10 nM), weak (+/−, >10nm) and – (not detected). Note that affinities varied significantly between reports. +(−) and ++(−) indicate contradictory data. ND, not determined. *: EphrinAs were reputed not to bind EphBs. Strong interaction was reported byCitation65 for ephrinA4 with EphB1-3 However, interaction for other ephrinAs was only determined with EphB2. Embryo: Functional assays for ephrinB1-3 and cognate receptors using stimulation of Xenopus tissues with soluble preclustered ephrin/Eph fragments.Citation47
Figure 5. Ephrin-Eph code at embryonic boundaries. The same code, based on selective ephrin-Eph pairs, is used in different configurations. In each case, repulsion results strongest at the tissue interface. (A–C) Simplified examples, taking into consideration a subset of ephrins and Eph receptors. A. Full complementary ephrin/Eph expression accounts for separation of the eye field and of the central hindbrain segments. (B) In the early gastrula, significant intracellular signaling occurs in the mesoderm, but strongest repulsion is restricted to the ectoderm-mesoderm contacts. EphrinB3 and EphB4, both enriched in the ectoderm, do not interact. (C) Hypothetical diagram of ephrin-Eph signaling in the somites. Segmentation may involve the combined activation of EphA4 by ephrinB2 and ephrinA1. EphA4 may be expressed in a gradient, with highest levels coinciding with the boundary. The distribution of ephrinB2 remains unclear, but may span the whole segment, thus activating some intratissular signal in the anterior half. (D) Complete description of ephrinB-Eph signaling at the ectoderm-mesoderm boundary. Individual contributions of each functional pair are semi-quantitatively symbolized by red lines of different thicknesses.
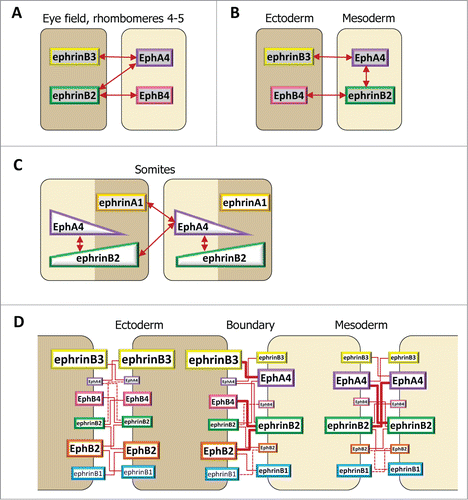