Figures & data
Figure 1. Examples of direct virulence gene regulation by the RpoE ESR. Two inducing signals (shown as lightening bolts) are required for activation. One is binding of a misfolded outer membrane protein (OMP) to DegS (AlgW in P. aeruginosa), which causes a conformational change that induces its proteolytic activity. The other is off-pathway LPS molecules, which bind to RseB (MucB in P. aeruginosa) and dissociate it from the antisigma factor RseA (MucA in P. aeruginosa). Both signals are required in order for DegS to cleave the periplasmic domain of RseA. The truncated RseA is then cleaved by the IM protease RseP (MucP in P. aeruginosa). This releases the cytoplasmic domain of RseA in complex with RpoE (σE; AlgU/T in P. aeruginosa), which is degraded by cytoplasmic proteases to release RpoE. RpoE-containing RNA polymerase binds directly to the algD promoter to induce alginate biosynthesis in P. aeruginosa and to the epsC promoter to induce T2SS production in V. cholerae. In E. coli K-12 the micA promoter is RpoE-dependent and drives the production of a non-coding sRNA that inhibits phoP translation (RpoE also induces 2 other sRNAs, RybB and MicL, which are not shown; see text). The predicted base pairing between MicA sRNA and phoPQ mRNA is conserved in pathogens including Salmonella, Shigella and Enterobacter. Therefore, RpoE might downregulate the virulence-associated PhoPQ regulon in multiple species. Mucoid P. aeruginosa strains from the lungs of individuals with cystic fibrosis most often have mutations that destroy antisigma factor RseA/MucA function, which leads to constitutive activation of RpoE, high algD operon expression and the production of large amounts of the exopolysaccharide, alginate.
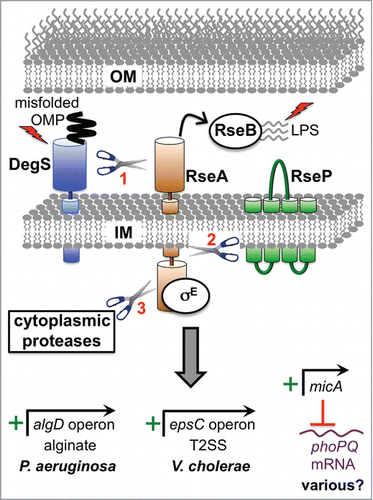
Figure 2. Examples of direct virulence gene regulation by the Cpx ESR. The Cpx response can be induced by various signals, with inputs entering at different points (surface attachment via NlpE to CpxA, external environmental changes via CpxA, and metabolic changes that alter the phosphorylation state of CpxR independently from CpxA). CpxP acts as a chaperone for misfolded proteins, directing them to destruction by the DegP protease (see text) and also negatively regulates the system, perhaps by interacting with CpxA. Phosphorylated CpxR activates the promoters of protein degradation factors (e.g., degP), protein-folding factors (e.g. dsbA) ands in multiple species, as well as many other genes associated with inner membrane functions. DegP and DsbA can reduce stress by promoting efficient assembly of the bundle forming pilus and Pap pilus in EPEC and UPEC, respectively. In addition, CpxR represses the promoters of the bfp and pap operons encoding these pili. In Y. pseudotuberculosis (Y. ptb) CpxR represses expression of several components of the Ysc-Yop T3SS. In Shigella sonnei CpxR directly activates the promoter of the gene encoding the major virulence regulator VirF, which leads to increased T3SS production because one VirF-induced target is the gene encoding the T3SS positive regulator NlpE/VirB (see text). CpxR also controls the T4SS in L. pneumophila by inducing genes encoding structural components (icm/dot) and by activating or repressing genes encoding some of the effectors exported by the T4SS.
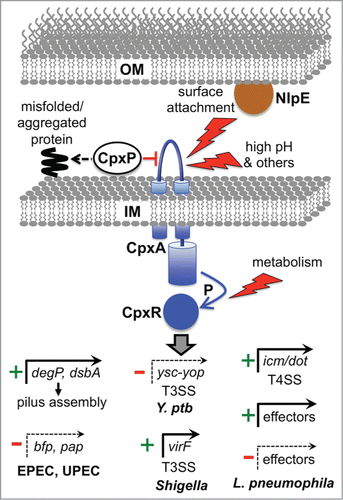
Figure 3. Examples of virulence gene regulation by the Rcs ESR. The Rcs system is induced by various signals deleterious to the outer membrane such as osmotic shock, desiccation, and overproduction of envelope proteins. Detection of some signals involves accessory proteins such as RcsF and others not shown. All signals converge of the IM histidine kinase RcsC, which transfers phosphate (P) to the cytoplasmic response regulator RcsB via the intermediary IM protein RcsD. RcsB can regulate target promoters as a homodimer or as a heterodimer with RcsA, or with the RcsA homologues RmpA and RmpA2 that are found in hypermucoviscous (HMV) strains of K. pneumoniae. Positively controlled target promoters include galF in encapsulated E. coli K strains, which increases precursors available for Group I capsule production. Similarly, ugd is an activated target in Salmonella, which is needed to make a precursor for LPS modification that increases CAMP resistance. In Y. enterocolitica (Y. ent) RcsB is involved in activation of the major operon encoding regulatory and structural components of the Ysa T3SS, but it is not known if this regulation is direct. Very recent data from Y. pseudotuberculosis (Y. ptb) has also shown that RcsB activates genes encoding the Ysc T3SS via direct induction of the lcrF gene, encoding the master Ysc T3SS regulator (see text). In Salmonella, RcsB drives the production of an antisense transcript encompassing the distal end of the fliLMNOPQR operon, which is involved in downregulating multiple virulence genes including those encoding components of the SPI-1 and SPI-2 T3SS systems. In HMV K. pneumoniae, RcsB, most likely as a heterodimer with RmpA or RmpA2, activates the cps operon responsible for capsular polysaccharide production.
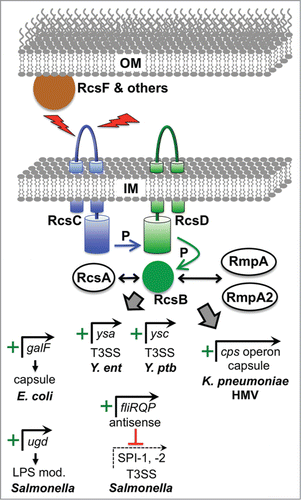
Figure 4. Virulence gene regulation by 2-component ESRs in Gram-positive bacteria. The ApsSR (also known as GraSR) system is activated by a variety of antimicrobial molecules, which are detected by ApsS. This leads to transfer of phosphate (P) from ApsS to ApsR, which can then bind to its target promoters. In some pathogens, ApsR targets include the promoters of dltABCD and mprF, which encode proteins involved in modifying teichoic acids and phospholipids, respectively, to reduce the net negative charge of the cell envelope and increase CAMP resistance. Another ApsR-dependent promoter drives vraFG expression, which encodes an ABC transporter that might be involved in efflux of toxic molecules out of the cell and/or their detection. The activity of the WalKR system is hypothesized to depend on the level of the peptidoglycan biosynthesis intermediate Lipid II, which might provide a report of cell wall synthesis activity and a healthy status of replicating cells. Furthermore, in B. subtilis WalK has been shown to interact with FtsZ at the division septum where cell wall metabolism is highly active, which might promote high WalK activity. WalK phosphorylates the response regulator WalR, which has many targets involved in cell division and cell wall metabolism. In. S. pneumoniae, one WalR-dependent promoter controls the pspA gene, which encodes the well-characterized virulence factor Pneumococcal Surface Protein A that is involved in complement evasion. In S. mutans, the WalKR system affects genes important for biofilm formation and attachment to tooth surfaces, with WalR binding directly to the promoters of at least 3 of them (gtfB, gtfC and ftf). In S. aureus, constitutive activation of WalR generates an inducing signal for the SaeSR system, which increases the production of many cell surface and secreted virulence factors. However, the SaeSR-inducing signal that is generated by WalR activation is not known.
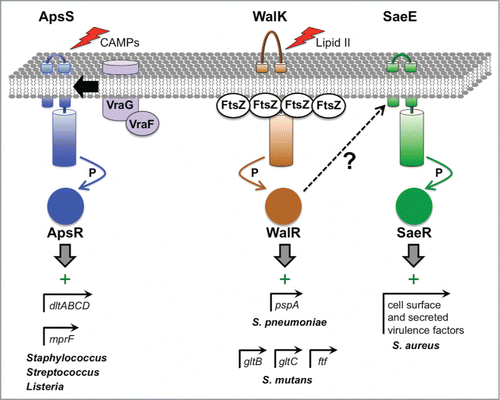