Figures & data
Table 1. Gram-positive eSTK-regulated transcription factors involved in virulence and infectionFootnotec
Figure 1. Schematic diagram showing the functional roles of eukaryotic serine-threonine kinases (eSTKs) and phosphatases (eSTPs). eSTKs contain an N-terminal cyclic AMP (cAMP)-dependent Protein Kinase domain (PKA; colored blue) and usually a C-terminal sensory domain. In non-mycobacterial Gram-positive bacteria the sensory domain is often a PASTA domain (purple half-rings). PASTA domains have been shown to bind free muropeptides (yellow balls) and cell wall-targeting antibiotics (e.g. β-lactams, orange diamonds). Once activated, eSTKs will dimerize and their intracellular kinase domains phosphorylate pleiotropic targets to produce specific cellular responses. eSTPs can, in turn, remove the phosphate(s) from an eSTK or substrates. Targets include metabolic enzymes, cell wall building and cell division regulatory enzymes such as DivIVA or FtsZ, translational machinery, and transcription factors (TFs) including sigma factors (σ) and possibly RNA polymerase (RNAP) holoenzyme subunits.
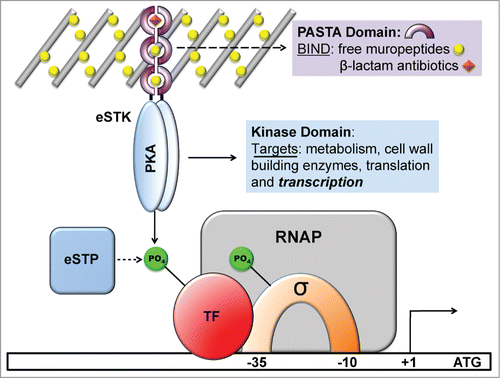
Figure 2. Atomic structures of eSTKs and eSTPs. (A) 3.0 Å atomic structure cartoon/surface of the PknB eSTK from M. tuberculosis (PDB code 1MRU; ref. Citation21). The two distinct lobes of the structure can easily be seen: (i) the N-terminal PKA subdomain consisting primarily of β-strands (colored green), and (ii) the a C-terminal subdomain almost entirely composed of α-helices (colored gray). Residues that make up the catalytic active pocket are colored in cyan. These residues include the metal (magnesium) binding domain, conserved Hank's-type ATPase active site motif “DXXPXN” and substrate interaction domain. The phosphothiophosphoric acid-adenylate ester (or AGS) substrate is colored red and magnesium ions colored magenta. (B) Cartoon/surface representation of the 2.65 Å atomic structure of the eSTP from S. agalactiae (PDB code 2PK0; ref.Citation48). The Flap domain, thought to be involved with substrate interactions, is colored green. The conserved “DMGG” catalytic pocket motif that coordinates 2 of the 3 magnesium ions (colored magenta) is shown in cyan. In the DMGG motif the sulfur atoms are colored yellow, nitrogens blue and oxygens red.
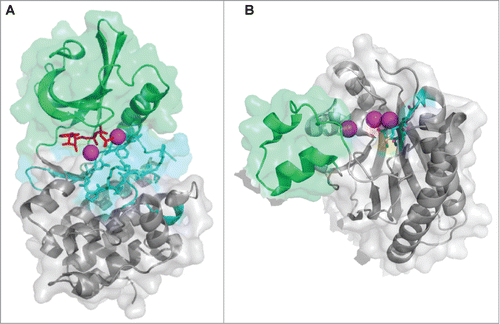
Figure 3. Structure and activation of REC domains. (A) Structure of the PhoB 2-component response regulator REC domain from Escherichia coli (PDB 1B00; ref. 158) shown in the inactivated, unphosphorylated state. PhoB exhibits a classical REC (α/β)5 topology, and α4-β5-α5 canonical dimeric interface. The proposed alternative dimer interface is the α1-α5.Citation63,169 The tyrosine and threonine involved in ‘Y/T’ coupling are colored in orange. Notice the tyrosine is protruding into the solvent area of the α4-β5-α5 face to presumably prevent dimerization and activation. Key residues involved in the active pocket phosphorylation events are colored cyan, including the invariant aspartate residue (D53) that is phosphorylated by the cognate histidine kinase. Nitrogen atoms are colored blue and oxygen atoms red. (B) Structure of activated, phosphorylated PhoB (Phospho-PhoB; PDB 1ZES; ref. 62). Upon D53 phosphorylation, the conserved Y/T residues move toward the catalytic pocket (the Y movement is denoted by the arrow) to coordinate the new phosphate group (colored yellow) and allow the dimer to form. The magnesium ion and surrounding electron cloud (Mg) is shown in magenta. (C) Overlay of PhoB and Phospho-PhoB structures from A and B. The black arrows indicate the movements of the Y/T residues to allow dimerization about the α4-β5-α5 interface.
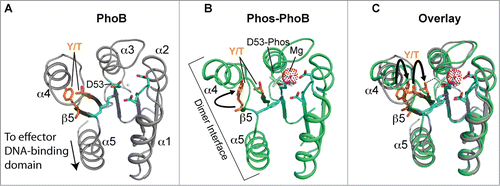
Figure 4. Overview of eSTK control of transcription in S. aureus. Schematic diagram showing the signaling pathways involved in eSTK control of S. aureus regulatory factors mentioned in this text. Phosphorylation is denoted by the circle with a “P.” Red circles indicate serine or threonine phosphorylation, brown cysteine phosphorylation and blue aspartate phosphorylation. Virulence and other traits that the regulators control are also described, as are regulated operons (if known). The TCS regulators GraR and VraR are phosphorylated by Stk1, as are the OCS regulators CcpA, MgrA and SarA. MgrA is phosphorylated by Stk1 on residues Ser110 and Ser113, and homologous sites yet to be verified experimentally are found in SarA and SarZ (see ). However, aside from being phosphorylated by Stk1, SarA is also phosphorylated by the SA0077 (Stk2) kinase on threonine residues. Stk2 is only present in some S aureus strains harboring methicillin resistance. The question mark labels indicate that the exact site(s) of phosphorylation has not been identified. TM, transmembrane region.
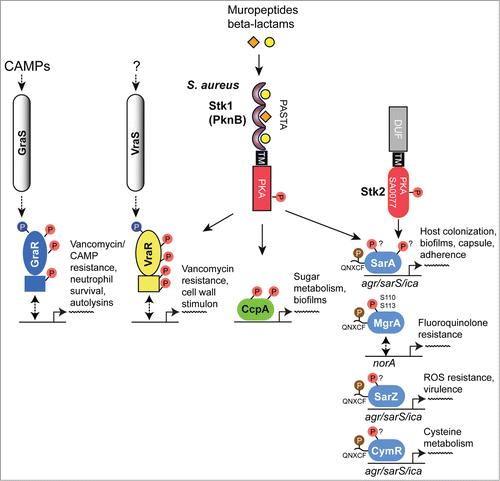
Figure 5. eSTK phosphorylation sites in S. aureus transcription. Domain architectures and identified phospho-residues (Serine (S), Threonine (T) or Cysteine (C), indicated by the arrows) are shown on the left. Some corresponding published atomic structures, or structural models that we have generated here as part of this review are shown on the right. Important residues are indicated in the structures. MgrA, SarZ, SarA and CymR all belong to the same family of transcription factors referred to here as the SarA/MgrA family and are regulated by phosphorylation of a conserved N-terminal cysteine residue (ref. 88). Only MgrA has been verified as having 2 additional eSTK-regulated sites (Ser110 and Ser113 within the C-terminal dimerization domain; ref. 82). Homologous Ser110/113 sites in SarZ, SarA and CymR regulators were found using 1- and 3-dimensional alignments. DD, dimerization domain; wHTH, winged helix-turn-helix domain; lac-HTH, LacI family HTH domain; Lux-HTH, LuxR family HTH domain; PBD, periplasmic binding domain; REC, receiver domain. Structures that are shown as dimers are depicted with one monomeric unit in color, and the other sister monomeric unit in gray. Phosphorylated residues are shown in red, except the phosphorylated cysteine in the SarZ structure is shown in orange, and the Ser101 and T119 sites in the VraR structure are colored yellow. The SarZ and VraR PDB codes used were 3HSE (ref. 159) and 4GVP (ref. 63), respectively. The GraR DNA-binding domain (DBD) atomic model was generated using a PhoB structure as a molecular template (PDB 1GXP; ref. 160) with Swiss-Model (ref. 161). All atomic figures were created with MacPyMol (ref. 162).
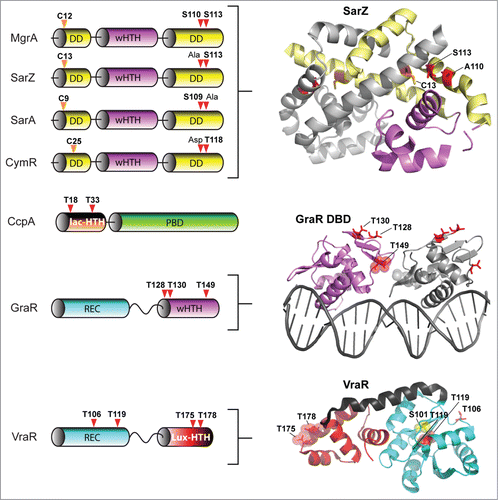
Figure 6. Overview of eSTK control of transcription in M. tuberculosis. Schematic diagram showing the signaling pathways involved in eSTK control of M. tuberculosis regulatory factors mentioned in this text. Phosphorylation is denoted by the circle with a “P.” Red circles indicate serine or threonine phosphorylation and blue aspartate phosphorylation. Virulence and other traits that the regulators control is described, as are regulated operons (if known). LBD, lipid-binding domain; β-Pro, β-propeller domain; TM, transmembrane region. Other abbreviations are also described in the text.
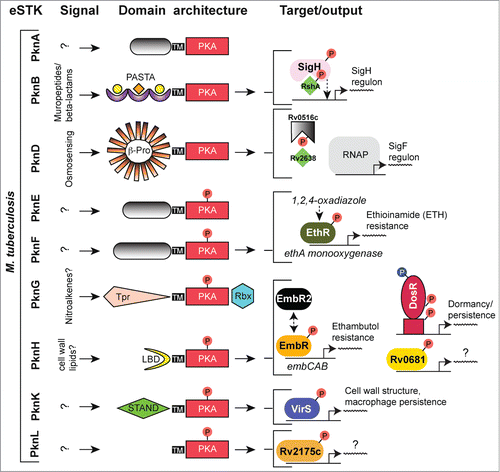
Figure 7. eSTK phosphorylation sites in M. tuberculosis transcription factors. Annotation is as per . Domain architectures are shown on the left, and 3-dimensional atomic structures and models on the right. The PDB codes for the DosR DNA-binding domain (DBD) and the EmbR-forkhead-associated domain (FHA; shown in blue) in complex with an interacting peptide (in magenta) and phosphate (shown in red) are 3C3W (ref. 163) and 2FF4 (ref. 114), respectively. The RshA anti σ-factor structure was generated with Swiss-Model using an available crystal structure from the anti-sigma factor ChrR (PDB code 2Q1Z; ref. 164) as a molecular template. eSTK phosphorylated residues are shown in red. Abbreviations not previous described are tet-HTH, TetR family helix-turn-helix domain; WHG, tryptophan-histidine-glycine domain presumed to bind a ligand or unknown effector molecule; UnKn ED, unknown effector domain; BTAD, bacterial transcriptional activator domain; wHTH*, wHTH domain missing helix 3; anti-σ: anti-sigma factor; anti-σ ID, anti-sigma factor interacting domain; PD, phosphorylation domain.
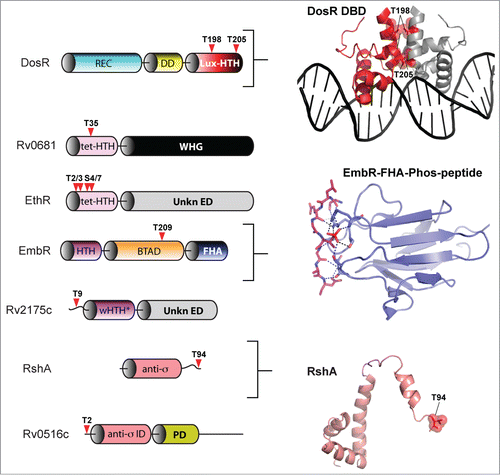
Figure 8. Overview of eSTK control of transcription in the streptococci. Schematic diagram showing the signaling pathways involved in eSTK control of Group A Streptococci (GAS), Group B Streptococci (GBS) and S. pneumoniae regulatory factors mentioned in this text. In GAS and GBS the PASTA-containing eSTK is called Stk1, and in S. pneumoniae StkP. Stk1 from both GAS and GBS modulates the TCS regulator CovR in these species, whereas WalR has thus far only been shown to be phosphorylated by Stk1 in GAS. Similarly, StkP modulates the TCS regulators RR06 and RitR in S. pneumoniae. The question mark shown above RitR indicates that aside form StkP phosphorylation, regulation from other input signals has not been identified. The phosphorylation PTM is denoted by the circle with a “P.” Red circles indicate serine or threonine phosphorylation, whereas blue indicates aspartate phosphorylation. Virulence and other traits that the regulators control are also described. Transmembrane region (TM). The TCS histidine kinases CovS, WalK and HK06 have 2 TMs each that are not annotated here for purposes of simplicity.
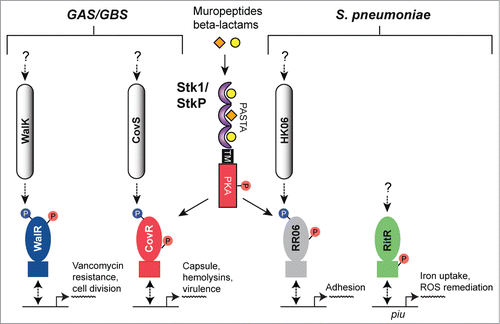
Figure 9. Comparison of CovR and VraR Receiver (REC) domain phosphorylation sites. (A) (left panel) Cartoon representation of an atomic model of the CovR REC domain. The model was created with Swiss-Model (ref.Citation161) using the CovR sequence from S. agalactiae and the PhoP structure from M. tuberculosis (PDB code 3R0J; ref.Citation165) as a molecular template. Phosphorylated residues are shown in red. The conserved histidine kinase phosphorylated aspartate (Asp53) is shown in green. Residues that would potentially pose a steric clash with the phosphorylated Thr65 are shown in yellow. (right panel) Surface representation of the left panel rotated 180 degrees. Notice that Thr65 is solvent accessible, but resides in a deep cleft within the interior of the protein. (B) The VraR atomic (REC) structure taken from ref.Citation63 showing the eSTK-regulated Thr119 (colored red) and potential residues that might clash with it in its phosphorylated form (colored yellow). The conserved Asp55 is shown in green.
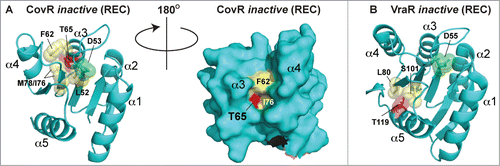
Figure 10. Possible mechanisms of eSTK/eSTP regulation of transcription. (A) eSTK phosphorylation of a transcription factor can cause either a negative effect (left) or positive effect (right) on the recruitment of transcriptional machinery through direct sigma factor and/or RNA polymerase (RNAP) interactions. (B) eSTK phosphorylation can also directly influence transcription factor binding to target DNA by either obstructing sister monomer interactions, or by directly affecting its physical hydrogen-bonding to target DNA. These influences can result in either a negative (left panel) or positive (right panel) effect on σ-factor/RNAP recruitment. (C) eSTK phosphorylation of both anti-sigma factors (-σ) and anti-anti-sigma factors (−2σ) can result in negative (left) or positive (right) effects on σ-factor/RNAP recruitment.
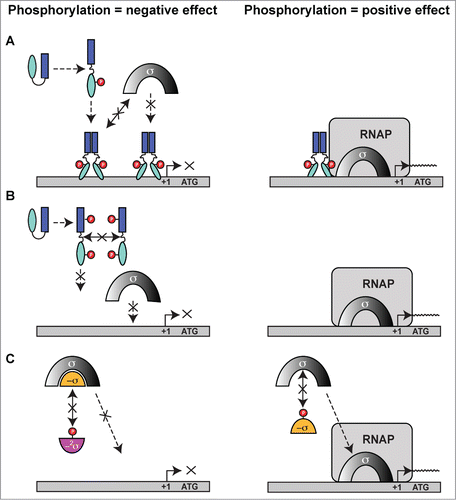