Figures & data
Figure 1 A stereoview of the cartoon representation of human p58C/3Q36. the 4Fe-4S cluster and the side chains of coordinated cysteine residues are shown as sticks.
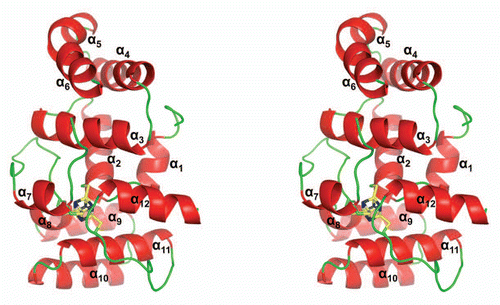
Figure 2 Comparison of the human p58C/3Q36 structure determined in current work with the corresponding domain structures reported for the yeast and human proteins. (A) Structure-based amino acid sequence alignment of the human and yeast primase 4Fe-4S domains. The sequences corresponding to α-helices and β-strands are highlighted by grey and yellow color, respectively. Conserved residues are shown in red and residues not in the final model are highlighted by cyan color. Residues involved in 4Fe-4S cluster coordination are indicated by triangles. (B and C) Comparison of the human p58C/3Q36 with the structures of the (B) yeast (3LGB) and (B) human (3L9Q) 4Fe-4S cluster domains. The labeled regions exhibiting the significant differences are discussed in the text
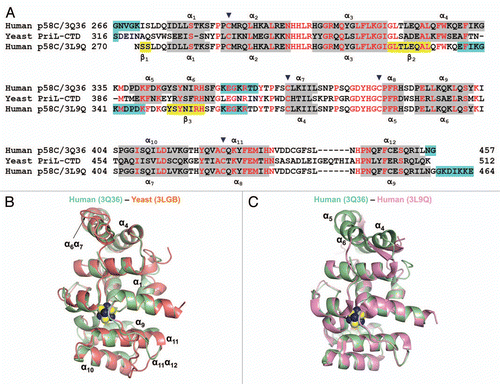
Figure 3 Examples of Far UV spectra of human p58C/3Q36 obtained at lower and high pH. The spectra are shown for the protein dialyzed against MES pH 6.5 and 40 mM NaCl (smooth line), and bicine pH 9.0 and 200 mM Li2SO4 (dotted line).
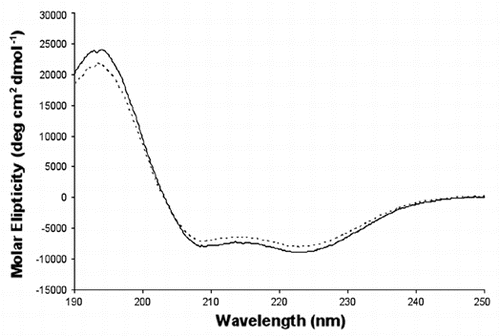
Figure 4 Crystal packing of the primase large subunit 4Fe-4S cluster domains. The figure highlights surrounding of helices α4, α5 and α6 in (A) human p58C/3Q36 and (B) yeast PriL-CTD and (C) β-strands in human p58C/3L9Q.
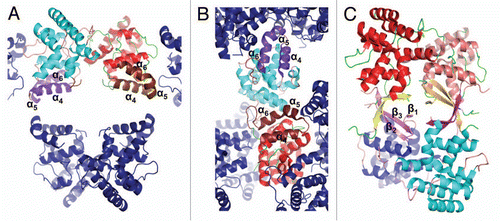
Figure 5 Comparison of yeast PriL-CTD and human p58C/3Q36 with the ssDNA-binding fragment of DASH cryptochrome 3 from Arabidopsis thaliana (PDB code 2VTB). The yeast PriL-CTD (residues 334–423) and human p58C/3Q36 (285–374) were superimposed with A. thaliana DASH cryptochrome 3 (373–457) with rmsd of 1.36 Å for 72 matched Cα atoms and rmsd of 1.32 Å for 66 matched Cα atoms, respectively. The protein fragments are drawn as cartoons. The FAD and ssDNA bound to a DASH cryptochrome 3 are shown as sticks.
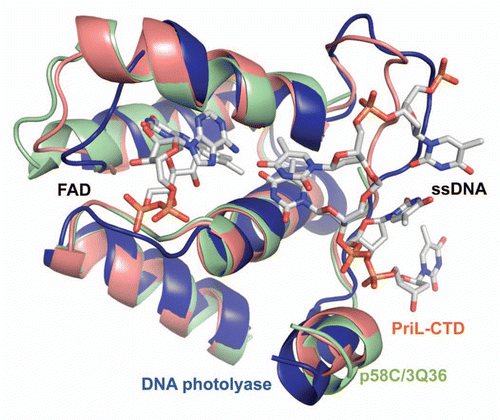
Figure 6 A proposed model of primer length counting and primer-template dissociation from DNA primase. (A) Initiation of primer synthesis. (B) Synthesis of unit length RNA primer causes a sterical hindrance. (C) Sterical hindrance induces the conformational change, resulting in RNA primer-template dissociation from primase and its transfer to Pol α.
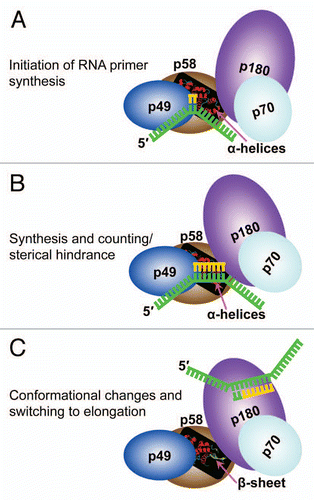
Table 1 Data collection and refinement statistics